Comparison of internal target volumes defined by three-dimensional, four-dimensional, and cone-beam computed tomography images of a motion phantom
Introduction
High precision radiation therapy (HPRT) allows a high dose of radiation to be delivered to the target volume while reducing the dose to organs at risk. However, the tumor motion induced by respiration during positioning and treatment delivery introduces a major challenge. In patients with non-small cell lung cancer (NSCLC) who undergo HPRT, accurate target delineation is of crucial importance.
Three-dimensional computed tomography (3DCT) is widely used in the delineation of target volume. However, high speed 3DCT with axial or helical mode always underestimates the tumor motion and distorts the reconstructed images of the thorax and abdomen (1). According to The International Commission on Radiation Units and Measurements Report 62 (ICRU62), to ensure dose coverage for mobile tumors, the internal target volume (ITV) includes the range of target motion during respiration on the basis of CTV (2). According to population-based motion information and guidelines, in conventional 3DCT-based treatment planning, the ITV is generated by drawing a margin on the basis of the gross tumor volume (GTV). However, the ITV contoured by this method is always larger than the real ITV, resulting in an overdose to normal tissue and geometric miss (3). Li et al. reported that the median degree of inclusion (DI) of planning target volume (PTV)3DCT in PTV4DCT was 51.60%, and the median DI of PTV4DCT in PTV3DCT was 99.94% for middle and lower lung cancer with free breathing (4).
Four-dimensional CT (4DCT), which allows data of full respiratory movement to be obtained, is widely used to assess respiration-induced tumor motion. After the 4DCT images have been acquired, a maximum-intensity projection (MIP) image is usually reconstructed to efficiently delineate the target volume (5,6). The ITV generated from MIP images of 4DCT scans has been widely considered to be the golden standard for the definition of individual targets (7-9). However, because the individual ITVs acquired in 4DCT simulation are not always consistent with the real ITVs in radiation delivery, the accuracy of this technique has been questioned. Moreover, under the conditions of irregular breathing and lesion motion amplitude >2 cm, the accuracy of ITVs obtained with 4DCT is reduced further (10-13).
A cone-beam CT (CBCT) scanner mounted on the gantry of an accelerator with a scan time of approximately 60 seconds during image acquisition can be used to obtain respiration information. Previous studies have shown a good volume consistency between CBCT and 4DCT (14,15). The visualized target volume in CBCT can be considered as an individual ITV which is similar to that of 4DCT. In the absence of 4DCT, ITVCBCT scans may act as a suitable substitute for ITV.
Though the 4DCT has been the golden technique to get individual ITV for SBRT of isolated lung cancer. It is difficult to abandon the isotropic margin accounting for the tumor motion in 3DCT based treatment planning. So, to investigate the individual ITV with different CT techniques and choose the optimal one or a combination of them is still a thing of great sense to do.
This study aimed to explore the volumetric and positional variations of GTV from different 3DCT scanning modes, and to compare the consistency of ITV between different 3DCT scan modes and CBCT and 4DCT by using a motion phantom and data from patients with NSCLC.
We present the following article in accordance with the MDAR reporting checklist (available at http://dx.doi.org/10.21037/atm-20-6246).
Methods
This study was approved by the Ethics Committee of Fujian Cancer Hospital, and written informed consent was obtained from all patients. All procedures performed in this study involving human participants were in accordance with the Declaration of Helsinki (as revised in 2013).
Respiratory phantom study
Motion phantom
The QUASARTM Programmable Motion Phantom (Modus Medical Devices Inc., London, ON, Canada) with a cubic target simulating sinusoidal motion, was chosen, and is shown in Figure 1. Three motion amplitudes (A) were set (5, 10, and 15 mm), and three motion frequencies (F) were set (20, 15, and 10 times/min). The theoretical ITV can be calculated based on the given diameter, motion, and magnitude of the motion target.
Patient characteristics
The 3DCT and CBCT images of 12 NSCLC patients who had been treated with intensity-modified radiation therapy (IMRT) between 2011 January and 2014 December were retrospectively analyzed. The patients had a median age of 65 (range, 60–78) years old. All of the patients were pathologically confirmed and were considered unsuitable for surgery. The patient and tumor characteristics are shown in Table 1.
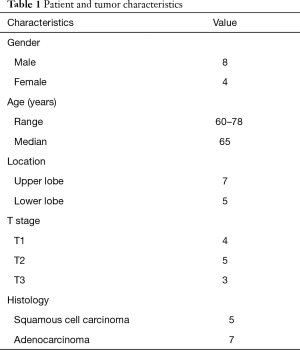
Full table
Acquisition of CT images
3DCT scanning of the phantom
The 3DCT images were acquired with a Philips Brilliance CT Big Bore scanner (Philips Medical Systems, Inc., Cleveland, OH, USA). The phantom was placed on the scanning bed and used to simulate the movement of a lung tumor in the cranial–caudal (CC) direction. Three scan modes were set as follows: (I) pitch =1.06, rotation time =1.0 s; (II) pitch =0.813, rotation time =1.5 s; and (III) pitch =0.313, rotation time =1.5 s. The 3DCT scanning parameters were as follows: a 2-mm slice thickness, 120 kV scan voltage, and 398 mA electric current. The DICOM data of the moving phantom were reconstructed with a 1-mm slice thickness and transferred to the Pinnacle treatment planning system (Version 9.2).
4DCT scanning of the phantom
For the 4DCT images, the motion parameters of the phantom were set as mentioned above. The scanning process involved a 50-second scanning time and a slice thickness of 2 mm. The respiratory signal was recorded by tracking the trajectory of the infrared markers placed on the phantom with the Real-time Position Management™ System (RPM, Varian Medical Systems, Palo Alto, CA, USA). The 4DCT images were sorted into 10 bins according to the phase of the breathing signal. MIPs of the 4DCT data sets were then generated and transferred to the Pinnacle treatment planning system (Version 9.2) to contour the ITV4DCT.
CBCT scanning of the phantom
Cone-beam CTs were acquired using a Varian® Trilogy linear accelerator fitted with an On-Board Imager® (OBI) with system version 1.4.13.0 (Varian Medical Systems, PaloAlto, CA). The same setup, frequency settings, and slice thickness were used for CBCT scanning. The setting parameters were as follows: Super mode; collimator, S20, filter, F0; frame number, 1,320; frame clockwise rotation range of the rack, −180° to +180°. The obtained images were reconstructed with a 1-mm slice thickness and transferred to the Pinnacle treatment planning system to contour the ITVCBCT.
3DCT and CBCT scanning of the patients
The 12 NSCLC patients were immobilized in the supine position with the arms raised above the head using vacuum bags. The 3DCT scanning was performed during free breathing using a Philips Brilliance CT Big Bore scanner (Philips Medical Systems, Inc., Cleveland, OH, USA). Images were reconstructed with a 2-mm slice thickness, and transferred to the Pinnacle treatment planning system. For the initial radiation treatment, CBCT scanning was used to verify the tumor position. The CBCT images were acquired by rotation from −180° to +180° and transmitted to the Pinnacle treatment planning system after reconstruction with a slice thickness of 2 mm.
GTV contouring and ITV generation
The GTV, ITV3DCT, ITVCBCT, and ITVMIP were contoured by the same oncologist. The GTV was contoured under the same lung window conditions for each patient, with a window width of 1,600 Hu and a window level of −600 Hu. The ITVCBCT was contoured with a window width of 400 Hu and a window level of 40 Hu. The volume difference was calculated using the Dice similarity coefficient (Dsc) (16) and volume difference (ΔV). Dsc represents the degree of overlap between the two volumes, with the Dsc value approaching 1 as the agreement improves and if the two volumes do not overlap at all, the Dsc will be zero. The Dsc is used to evaluate the match index of the two volumes and is defined as follows:
[1]
ΔV illustrates how the A is located within the B and is described as follows:
[2]
Statistical analysis
All statistical analyses were performed using the SPSS17.0 package (SPSS Inc, Chicago, IL, USA). The Wilcoxon test was used to compare the tumor volumes and the central position gained by different scanning modes. Differences were considered to be significant if the P value was less than 0.05.
Results
Motion phantom study
Geometrical variations of GTV from 3DCT scanning
GTV was contoured on 162 series of 3DCT images, and the length of the GTV in the CC direction along with the target motion was analyzed. The median length of the GTV was 38 mm (range, 18–59 mm). The characteristics of GTV length are shown in Figures 2 and 3.
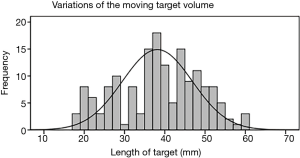
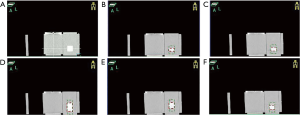
Centroid position deviation derived from the three 3DCT scanning modes
The position of the center of the mass (COM) in the CC direction was analyzed. The mobility vector in the x, y direction was 0, and the vector in the z direction was the amplitude of the phantom. Figures 4 and 5 show the deviation of the target center. As more respiration information was incorporated into scanning mode III, the mean deviation of COM derived was significantly smaller than that of scanning modes I and II (P<0.001). The variation in the centroid position of scan mode I, II and III were 2.3±4.7 mm (range, −10–16 mm), 2.6±4.0 mm (range, −5.5–11.5 mm), and 1.0±1.4 mm (range, −2–5 mm), respectively.
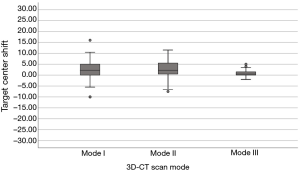
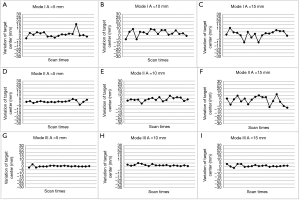
The length of the ITVCBCT compared to that of ITVMIP
ITVCBCT was positively correlated with the amplitude of the phantom. The ITV length from CBCT showed a deduction rate of 3.1–9.3% compared to that of 4DCT. The MIP derived from 4DCT with a clear visualization of the target without motion artifacts is taken as the golden standard for estimating the target motion. For the three amplitudes (A=5 mm, 10 mm, 15 mm), the mean ratio of ITV from CBCT to 4DCT was 0.97, 0.91, and 0.91, respectively. Table 2 shows the characteristics of the ITVCBCT.
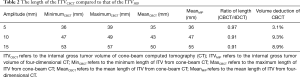
Full table
Comparison of the target length between ITV3DCT, ITVCBCT, and ITVMIP
Compared to those with scanning modes I and II, the ITV derived from scanning mode III showed a good consistency with ITVMIP and ITVCBCT, with a better DSC and smaller ΔV. The Dsc between ITVI, ITVII, ITVIII, and ITVMIP were 0.78±0.77 (range, 0.45–0.91), 0.86±0.1 (range, 0.57–0.99), and 0.94±0.05 (range, 0.77–1.0), respectively. The ΔV between ITVI, ITVII, ITVIII and ITVMIP was 29.67% (range, 0–63.89%), 17.22% (range, 0–57.45%), 6.46% (range, 0–23.4%), respectively (Figure 6). The mean ratio of ITVI, ITVII, ITVIII to ITVCBCT were 1.21, 0.91, and 1.06, respectively, and the mean ratios to ITVMIP were 1.16, 0.85, and 0.95, respectively. ITVI with a margin of 10-mm on CC direction and ITVII with a margin of 0-mm on the basis of GTV showed poor consistency with ITVCBCT and ITVMIP (P<0.05). ITVIII without a margin showed good consistency with ITVMIP and ITVCBCT (P=0.044 and P=0.082, respectively). The data are shown in Table 3.
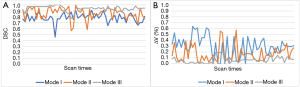
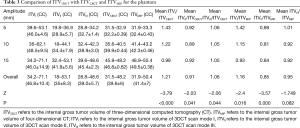
Full table
Patients
CBCT and 3DCT were performed in 12 patients with lung cancer who had previously received IMRT. The ITVCBCT and ITV3DCT [with a 1-cm extension on the CC direction, and a 0.5-cm extension on the left-right (LR) and superior-inferior (SI) directions] were compared, and the volume difference between them was statistically significant (P=0.002). The difference between ITVCBCT and ITV3DCT was expressed by V, and the mean value of V was 35.4 cm3. The mean Dsc and ΔV between ITV3DCT and ITVCBCT were 0.50 and 60.76%, respectively. Data was shown in Tables 4,5 and Figure 7.
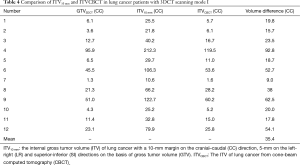
Full table
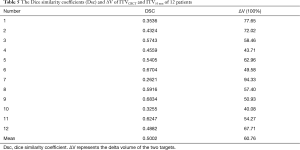
Full table
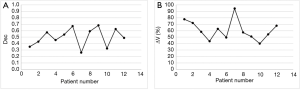
Discussion
Physiologic motions, especially respiratory movement, are known to influence the precision of radiation procedures in the treatment of thoracic tumors. The images obtained by conventional 3DCT only represent the random shapes and volumes during the period of respiration and cannot truly reflect all of the necessary respiratory movement information. Slow scanning methods include slow spiral CT and CBCT, are more reproducible and can better capture tumor movement as a result of the larger CTV and a higher accuracy rate (17,18). The ITV from 4DCT is a fusion of the GTVs contoured from the 10 respiratory phases during scanning and is considered to be the golden standard for ITV contouring (7-9).
In order to validate that the image from conventional 3DCT scan was the instant shape and volume of a phase from respiratory cycle that comes randomly, we applied three different 3DCT scanning modes by adjusting the pitch and rotation time to scan the phantom in our study. We found out the variation of the target volume and central position were significantly correlated with the scan mode. For the cubic target with a diameter of 30 mm, the obtained length of the GTV varied greatly from 18–59 mm. The ITV became larger as the motion amplitude increasing for scan mode II and III, while this relationship can’t be detected by scan mode I. The mean and standard deviation of the centroid shift decreased as the scan time being longer for ITVI, ITVII and ITVIII with value of 2.3±4.7, 2.6±4.0, and 1.0±1.4 mm, respectively. In this study, the motion cycle of the phantom was 3–6 seconds for three motion amplitudes. For scanning mode I with a pitch value of 0.813 and a rotation time of 1.0 seconds, the acquisition time was shorter than a motion cycle, leading to the great variation in centroid position and volume of GTV. While the scanning mode III with a smaller pitch value of 0.313 and a longer rotation time of 1.5 seconds resulted in a longer image acquisition time than the motion cycle. As more respiration information was incorporated into scanning mode III, the mean deviation of the central position and the target volume derived was significantly smaller than that of scanning modes I and II (P<0.001). We also found out that the deviation of central position became significantly greater as the motion amplitude increased (P<0.05) under same scanning mode. This result indicated that the tumor motion may contribute to increasing the target volume variation, because a tumor with larger motion amplitude is likely to produce more residual motion artifacts. Similar results were also reported by Chen et al. (1), who demonstrated that the volume and position of the targets obtained by conventional spiral CT scanning were obviously different and the obtained GTV was distorted after 3D reconstruction. Li (4) also evaluated the centroid shifts of PTVs derived from 3DCT and demonstrated the uncertainty of a target derived from 3DCT. In our study, we found the GTV3DCT from scan mode I, II underestimate the MIP volume by 28%, 15% respectively and they were statistically significant with P value of 0.000. This result was similar to the previous study from Lingaiah et al. (15), who found that the GTV by helical mode scanning underestimate the MIP volume by 13% and 21% respectively and was statistically significant with a P value of 0.00. In addition, our study showed the GTV3DCT from scan mode III underestimate the MIP volume only by 5% and this difference was no statistically significant (P=0.082). Thus, scan mode III could effectively decrease the uncertainty and variation from the variation of respiration. This certainly due to the smaller pitch value and longer rotation time of scan mode III which guarantee much more time to acquire much more target motion information.
By analyzing the Dsc and ΔV between targets, the difference in ITV obtained through different imaging techniques can be more clearly established. The Dsc between targets was directly influenced by the shift of the target central position. In our study, we evaluated the Dsc between ITV3DCT, and ITVCBCT and ITVMIP. For the 3DCT scan mode I and II, the ITV obtained was much larger than the ITVCBCT or ITVMIP for the phantom. A good similarity between ITVIII and ITVMIP with a mean Dsc and ΔV of 0.94 and 6.46% was showed in our study. The mean ratio of ITVIII to ITVCBCT and ITVMIP was 1.06 and 0.95, respectively. The present data suggest that scanning mode III, with a pitch of 0.313 and rotation of 1.5 seconds, could obtain considerably more respiration information and had a good consistency with 4DCT. The ITVs from scan mode III may be considered as individual ITVs for IMRT in lung cancer.
The data of 12 patients with lung cancer who had previously received radiotherapy were also analyzed. The defined ITV3DCT with a margin of 10 mm in the CC direction and 5 mm in the SI and LR directions showed an increasing volume of 35.27 cm3 and a mean size ratio of 3.1 compared to ITVCBCT. Poor consistency was revealed between the ITVCBCT and ITV3DCT, with a mean DSC of 0.50 and ΔV of 60%. These results support those of some previous studies (3,19,20). For instance, Li et al. investigated thirty-one patients with NSCLC underwent 3DCT and CBCT scans of the thorax during free breathing and found the mean Dsc between the two image modalities was 0.66 and the ratio of ITV3DCT to ITVCBCT was 2.33. For the 12 patients undergo 3DCT scan mode I, the corresponding ITV derived was much larger than the ITVCBCT, which resulted in good coverage of tumor motion while carrying a high possibility of unnecessary irradiation to normal tissue.
CBCT images may suffer from projection deduction due to the interplay between the gantry rotation and the motion target. The volume from CBCT possessed great reproducibility for a certain amplitude in this study, this suggested that the image projection deduction may have no correlation with the amplitude of motion. In our study, the ITV obtained from CBCT showed a volume deduction of 3.1–9.3% compared to the ITV obtained from the MIP image of 4DCT. This result was similar to those of some reports; for instance, Wang et al. (14) reported a volume difference of less than 8%. Our study showed that the volume ratio of CBCT to 4DCT was 0.93. Lingaiah et al. (15) reported that CBCT was well correlated with the 4DCT volume, with a ratio of 1.02. Although the ITVCBCT was slightly smaller than the ITV4DCT, it contained considerably more respiratory information due to its longer scan time.
Previous studies (10,21) have reported that under sinusoidal breathing conditions, CBCT and 4DCT can realistically reflect the actual range of tumor respiratory motion; however, when the respiratory amplitude is too large, or the respiratory curve is irregular, the ITVs obtained by CBCT and 4DCT are often partially deleted, making them smaller than that of the theoretical ITV. Under such conditions, the volumes of CBCT and 4DCT can be reduced by 20–30% and the length of the ITV can be reduced by 7–11 mm. Our study also evaluated the difference in ITV gained from different CT scans and came to a similar conclusion.
In this article, we verified the randomness of GTV from 3DCT scanning and investigate the deviation of ITV from different 3DCT scan modes and different CT scan techniques. There is still much work to do to improve the techniques of 3DCT and 4DCT and to explore a optimized combination of these techniques.
Our study has some limitations that need to be addressed. First, the study used only three sinusoidal phantom motions, which were constrained to the CC direction, and the data of the patients involved were collected and analyzed retrospectively. In addition, though we set strict criteria for collecting the patients’ data, the 12 number of the patients was also limited. These situations are certainly ideal; however, it may not be the case in a clinical setting. Further investigations involving 3D target motion and non-sinusoidal motion patterns as well as a larger amount of real patients’ breathing patterns between 3DCT scanning mode III, CBCT, and 4DCT would be worthwhile.
Conclusions
Our results indicated a good correlation between the ITV got from 3DCT scan mode III and MIP volume. The ITV from CBCT showed a deduction rate of 3.1–9.3% compared to 4DCT. In cases where 4DCT scan imager is not possible, this “slow” 3DCT scan mode III or CBCT scan may be considered for determining ITV volumes, with a small additional isotropic margin for safer treatment. The GTV obtained from 3DCT scan mode I had a strong uncertainty, and the target centroid position deviated with great randomness; the ITV formed on the basis of this GTV3DCT was larger than the ITVCBCT or ITV4DCT, which may lead to geographical miss radiation of the target and excessive radiation of surrounding normal tissue.
Acknowledgments
None.
Footnote
Reporting Checklist: The authors have completed the MDAR reporting checklist. Available at http://dx.doi.org/10.21037/atm-20-6246
Data Sharing Statement: Available at http://dx.doi.org/10.21037/atm-20-6246
Conflicts of Interest: All authors have completed the ICMJE uniform disclosure form (available at http://dx.doi.org/10.21037/atm-20-6246). The authors have no conflicts of interest to declare.
Ethical Statement: The authors are accountable for all aspects of the work in ensuring that questions related to the accuracy or integrity of any part of the work are appropriately investigated and resolved. This study was approved by the Ethics Committee of Fujian Cancer Hospital, and written informed consent was obtained from all patients. All procedures performed in this study involving human participants were in accordance with the Declaration of Helsinki (as revised in 2013).
Open Access Statement: This is an Open Access article distributed in accordance with the Creative Commons Attribution-NonCommercial-NoDerivs 4.0 International License (CC BY-NC-ND 4.0), which permits the non-commercial replication and distribution of the article with the strict proviso that no changes or edits are made and the original work is properly cited (including links to both the formal publication through the relevant DOI and the license). See: https://creativecommons.org/licenses/by-nc-nd/4.0/.
References
- Chen GT, Kung JH, Beaudette KP. Artifacts in computed tomography scanning of moving objects. Semin Radiat Oncol 2004;14:19-26. [Crossref] [PubMed]
- Wambersie A, Landberg T. ICRU report 62, prescribing, recording and reporting photon beam therapy (supplement to ICRU Report 50). ICRU News. 1999.
- Li F, Li J, Xing J, et al. Analysis of the advantage of individual PTVs defined on axial 3D CT and 4D CT images for liver cancer. J Appl Clin Med Phys 2012;13:4017. [Crossref] [PubMed]
- Li FX, Li JB, Zhang YJ, et al. Comparison of the planning target volume based on three-dimensional CT and four-dimensional CT images of non-small-cell lung cancer. Radiother Oncol 2011;99:176-80. [Crossref] [PubMed]
- Underberg RW, Lagerwaard FJ, Slotman BJ, et al. Use of maximum intensity projections (MIP) for target volume generation in 4DCT scans for lung cancer. Int J Radiat Oncol Biol Phys 2005;63:253-60. [Crossref] [PubMed]
- Mori S, Kanematsu N, Asakura H, et al. Projection-data based temporal maximum attenuation computed tomography: determination of internal target volume for lung cancer against intra-fraction motion. Phys Med Biol 2007;52:1027-38. [Crossref] [PubMed]
- Cai J, Read PW, Sheng K. The effect of respiratory motion variability and tumor size on the accuracy of average intensity projection from four-dimensional computed tomography: an investigation based on dynamic MRI. Med Phys 2008;35:4974-81. [Crossref] [PubMed]
- Hughes S, McClelland J, Chandler A, et al. A comparison of internal target volume definition by limited four-dimensional computed tomography, the addition of patient-specific margins, or the addition of generic margins when planning radical radiotherapy for lymph node-positive non-small cell lung cancer. Clin Oncol (R Coll Radiol) 2008;20:293-300. [Crossref] [PubMed]
- Gabryś D, Kulik R, Trela K, et al. Dosimetric comparison of liver tumour radiotherapy in all respiratory phases and in one phase using 4DCT. Radiother Oncol 2011;100:360-4. [Crossref] [PubMed]
- Clements N, Kron T, Franich R, et al. The effect of irregular breathing patterns on internal target volumes in four-dimensional CT and cone-beam CT images in the context of stereotactic lung radiotherapy. Med Phys 2013;40:021904. [Crossref] [PubMed]
- Britton KR, Starkschall G, Tucker SL, et al. Assessment of gross tumor volume regression and motion changes during radiotherapy for non-small-cell lung cancer as measured by four-dimensional computed tomography. Int J Radiat Oncol Biol Phys 2007;68:1036-46. [Crossref] [PubMed]
- James SS, Mishra P, Hacker F, et al. Quantifying ITV instabilities arising from 4DCT: a simulation study using patient data. Phys Med Biol 2012;57:L1-7. [Crossref] [PubMed]
- Pötter R, Georg P, Dimopoulos JC, et al. Clinical outcome of protocol based image (MRI) guided adaptive brachytherapy combined with 3D conformal radiotherapy with or without chemotherapy in patients with locally advanced cervical cancer. Radiother Oncol 2011;100:116-23. [Crossref] [PubMed]
- Wang Z, Wu QJ, Marks LB, et al. Cone-beam CT localization of internal target volumes for stereotactic body radiotherapy of lung lesions. Int J Radiat Oncol Biol Phys 2007;69:1618-24. [Crossref] [PubMed]
- Lingaiah R, Ali MA, Kulsum U, et al. GTV volume estimation using different mode of computer tomography for lung tumors in stereotactic body radiation therapy. Polish Journal of Medical Physics Engineering 2019;25:29-34. [Crossref]
- van Dam IE, van Sörnsen de Koste JR, Hanna GG, et al. Improving target delineation on 4-dimensional CT scans in stage I NSCLC using a deformable registration tool. Radiother Oncol 2010;96:67-72. [Crossref] [PubMed]
- Lagerwaard FJ, Van Sornsen de Koste JR, Nijssen-Visser MR, et al. Multiple "slow" CT scans for incorporating lung tumor mobility in radiotherapy planning. Int J Radiat Oncol Biol Phys 2001;51:932-7. [Crossref] [PubMed]
- van Sörnsen de Koste JR, Lagerwaard FJ, Nijssen-Visser MR, Graveland WJ, et al. Tumor location cannot predict the mobility of lung tumors: a 3D analysis of data generated from multiple CT scans. Int J Radiat Oncol Biol Phys 2003;56:348-54. [Crossref] [PubMed]
- Underberg RW, Lagerwaard FJ, Cuijpers JP, et al. Four-dimensional CT scans for treatment planning in stereotactic radiotherapy for stage I lung cancer. Int J Radiat Oncol Biol Phys 2004;60:1283-90. [Crossref] [PubMed]
- Li F, Li J, Ma Z, et al. Comparison of internal target volumes defined on 3-dimensional, 4-dimensonal, and cone-beam CT images of non-small-cell lung cancer. Onco Targets Ther 2016;9:6945-51. [Crossref] [PubMed]
- Vergalasova I, Maurer J, Yin FF. Potential underestimation of the internal target volume (ITV) from free-breathing CBCT. Med Phys 2011;38:4689-99. [Crossref] [PubMed]