Inhibition of S-adenosyl-L-homocysteine hydrolase alleviates alloimmune response by down-regulating CD4+ T-cell activation in a mouse heart transplantation model
Introduction
In the methionine cycle, methyltransferase utilizes S-adenosylmethionine (SAM) as a methyl donor and forms S-adenosyl-L-homocysteine (SAH) as a by-product, regardless of the protein, nucleic acid, or small molecule substrate. S-adenosyl-L-homocysteine hydrolase (SAHH) is a highly conserved enzyme that catalyzes the reversible hydrolysis of SAH to adenosine (ADO) and L-homocysteine (HCY). SAHH knockdown impacts cellular methylation potential, cell morphology, cell cycle, and proliferation rates (1). Inhibition of SAHH in vitro and in vivo results in the accumulation of SAH, an inhibitor of methyltransferase that utilizes SAM as the methyl group donor. SAH is a potent inhibitor of SAM-dependent methyltransferases, and most biological methylation reactions are catalyzed by methyltransferases with SAM as the methyl donor (2). The use of advanced immunosuppressive therapies can prevent acute allograft rejection. However, in some cases, immunosuppressive therapies fail, leading to early organ graft rejection. Furthermore, excessive immunosuppression can render patients prone to infectious diseases.
There are 3 types of SAHH inhibitors (3): type I and type II inhibitors irreversibly inactivate the enzyme, whereas type III inhibitors reversibly bind to the open form of SAHH, inhibiting its 3-oxidative and the 5-hydrolytic activity. DZ2002 is a reversible SAHH inhibitor, whereas AdOx is an irreversible SAHH inhibitor (4).
Lymphocyte activation and function are highly dependent on transmethylation reactions (5), and alloimmunity is closely associated with CD4+ T cell-mediated immune responses. Therefore, we hypothesized that SAHH inhibitors may be effective in allograft immunosuppression. The aim of this study was to investigate the effects of the reversible SAHH inhibitor DZ2002 and irreversible SAHH inhibitor AdOx on mouse cardiac transplantation, as well as on CD4+ T cells and Th1/Th17/regulatory T cell (Treg)-mediated alloimmune responses in allograft rejection.
We present the following article in accordance with the ARRIVE reporting checklist (available at http://dx.doi.org/10.21037/atm-20-2899).
Methods
Mice
Male BALB/c and C57BL/6 mice (6–8 weeks old; 20–25 g) were purchased from the Animal Center of Tongji Medical College. Mice were bred in pathogen-free conditions at the Animal Center of Tongji Medical College. We generated a murine heterotopic heart transplantation (HTx) model using BALB/c mice as donors and C57BL/6 mice as recipients. Recipients were divided into 3 groups (n=5 for each group) and were treated daily with an intraperitoneal injection (i.p.) of either 30 mg/kg DZ20002 (MCE, USA), 3 mg/kg AdOx (SELLECK, USA), or DMSO after transplantation. Experiments were performed under a project license (IACUC Number: 2358) granted by the Tongji Medical College, HUST Institutional Animal Care and Use Committee, in compliance with institutional guidelines for the care and use of animals.
Histological analysis of acute allograft rejection
Mice were anesthetized and disinfected, and the spleens were isolated on day 7. Allograft tissue sections were stained with hematoxylin and eosin (H&E) and Masson’s trichrome. Severe damage was histologically determined by assessing the extent of inflammatory cell infiltration, myocardium cell injury with interstitial inflammation, and rejection PR scores. High-power fields (400×) in the allograft were scored for each animal; scoring was performed on blinded slides. Immunohistochemical staining for CD4 was performed, and the number of positive cells was averaged from 5 random high-power fields.
Cell preparation
Cells were isolated from spleens in RPMI medium supplemented with 1% FBS. Following red blood cell lysis, the cell suspension was filtered using a 40-µm mesh. CD4+ T cells were purified from spleens and lymph nodes via magnetic selection according to the manufacturer’s instructions (Miltenyi Biotec). Purified CD4+ T cells were cultured in 12-well plates at 1×106 cells/well with anti-CD3 (2 µg/mL) and anti-CD28 (2 µg/mL) monoclonal antibodies.
Flow cytometry
Lymphocyte suspensions were prepared from the excised spleens. Cells were stained using fluorescently labeled monoclonal antibodies. The following monoclonal antibodies were used (all from BD Biosciences, San Diego, CA, USA): FITC-anti-CD4, PE-CY7-anti-CD8, APC-anti-CD44, PE-anti-CD62L, APC-anti-T-bet, PE-anti-Rorγt, PE-CY7-anti-Foxp3, FITC-anti-annexin-V, PI-APC-anti-IFN-γ, PE-anti-IL-17A, PE-CY7-anti-IL4, and APC-CY7 Live/Dead. For intracellular cytokine staining, cells were stimulated using a Cell Activation Cocktail (with brefeldin A) (BD Biosciences, San Diego, CA, USA) (2 µg/mL) for 4 h and then collected for intracellular staining. Marker expression was assessed by flow cytometry using a FACS Calibur Flow Cytometer (BD Biosciences, San Diego, CA, USA). Flow cytometry data were analyzed using Flowjo V10 (Tree Star, Ashland, OR, USA).
Western blotting
Western blotting was performed as previously described (6). Briefly, allografts were harvested and lysed on ice using RIPA Lysis Buffer (Beyotime, Shanghai, China) containing a protease inhibitor PMSF (Beyotime). Protein concentrations were determined using a protein assay kit (eBioscience). Equal amounts of proteins were resolved by 10% SDS-polyacrylamide gel electrophoresis (PAGE) and transferred onto nitrocellulose membranes. Proteins were visualized using HRP-conjugated anti-mouse or anti-rabbit IgG and the ECL system (Amersham Biosciences).
Statistical analysis
Data are presented as the mean ± standard deviation (SD). Kaplan-Meier survival plots were used to assess mean graft survival and compared using a two-tailed Student’s t-test. Data were analyzed using Prism 7 (GraphPad Software, La Jolla, CA, USA). P values <0.05 were considered statistically significant (*, P<0.05; **, P<0.01; ***, P<0.001).
Results
SAHH inhibition prolongs graft survival time in the HTx mouse model
To determine the effect of SAHH inhibition on acute allograft rejection in the HTx model, mice were treated with DZ2002 or AdOx. Heart grafts from control mice exhibited extensive cellular infiltration and severe tissue damage 7 days after transplantation (Figure 1A,B). By contrast, heart grafts from the DZ2002 and AdOx-treated mice showed intact myocytes, and very few infiltrating cells 7 days after transplantation. The PR scores in AdOx and DZ2002 groups were significantly lower than the control group (Figure 1C). In addition, AdOx was more potent in attenuating acute rejection than the reversible SAHH inhibitor DZ2002. Both AdOx and DZ2002 significantly prolonged graft survival time compared with the control group (Figure 1D).
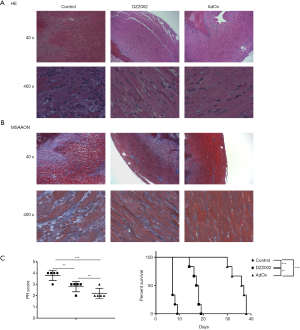
SAHH inhibition suppresses T cell activation in the HTx mouse model
To study the mechanism by which SAHH inhibitors attenuate acute allograft rejection, we firstly analyzed the activation markers of T cells isolated from the HTx mice spleens by flow cytometry. Effector T cell displayed an activated CD44+CD62L− T cell phenotype. The percentage of CD44+CD62L−CD4+ T cells in both DZ2002 and AdOx groups was significantly lower than in the control group (Figure 2A,B). However, there was no significant difference between the DZ2002 group and AdOx group. Among CD8+ T cells, the percentage of CD44+CD62L− cells in AdOx group was markedly lower than in the control and DZ2002 groups, while the percentage in the DZ2002 group was not significantly different from the control group (Figure 2C,D). Hence, inhibition of methyltransferases in the methionine cycle by SAHH inhibitors reduces T cell activation in the HTx model.
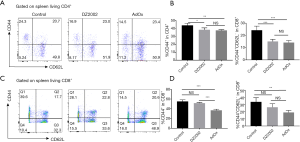
SAHH inhibition suppress effector T cell differentiation and function in the HTx mouse model
Although T cell activation was impaired by SAHH inhibitors, more than 60% of T cells in both inhibitor groups were activated compared with control. Differentiation and function of activated T cells upon SAHH inhibition were investigated. To this end, we assessed the expression of the key transcription factors of Th1 and Th17 cells (T-bet and RORγt, respectively) by flow cytometry. The percentage of T-bet+CD4+ T (Figure 3A,B) cells and RORγt+CD4+ T (Figure 3A,C) cells in both inhibitor-treated groups were significantly decreased compared with the control group.
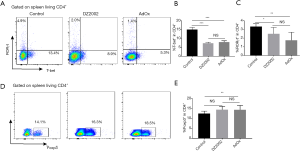
Irreversible SAHH inhibition facilitates Treg differentiation in the HTx mouse model
Besides effector T cells, Tregs also have an important role in rejection or tolerance in transplantation immunity. To investigate the effects of SAHH inhibition on Treg differentiation, we detected the expression of Foxp3 in the HTx model by flow cytometry. Although DZ2002 did not affect Treg differentiation, AdOx significantly enhanced the differentiation of Tregs (Figure 3D,E). These results suggest that irreversible SAHH inhibition induces immune-suppressive responses in the HTx model.
SAHH inhibition induced a weaker Th1/Th17 response in cardiac rejection
The function of different effector CD4+ T cell subtypes was investigated by staining for different cytokines after T cell stimulation with phorbol myristate acetate (PMA) and ionomycin. The percentage of INF-γ+CD4+ (Figure 4A,B) cells and IL-17+CD4+ (Figure 4A,C) T cells in both inhibitor-treated groups were lower compared with the control group. The percentage of IL-4-expressing CD4+ T cells in both inhibitor-treated groups were also significantly lower compared with the control group (Figure 4D,E). These results suggest that both SAHH inhibitors suppress effector T cell differentiation and function; however, the irreversible SAHH inhibitor (AdOx) is more potent than the reversible SAHH inhibitor (DZ2002).
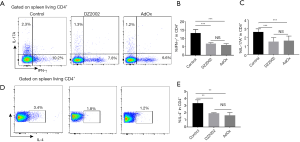
SAHH inhibition impairs CD4+ T cell infiltration in cardiac allografts
Infiltration of activated CD4+ T cells induces cell-mediated rejection of cardiac allografts. Allograft tissue sections were subjected to immunohistochemical staining, which revealed profound infiltration of CD4+ T cells 7 days post-operation (Figure 5A). Semiquantitative analysis of representative high magnification fields revealed that the number of CD4+ cells infiltrated in the allograft was decreased compared with the DMSO group (Figure 5B). These results suggest that both reversible and irreversible SAHH inhibition down-regulate CD4+ T cell responses in the HTx model. SAHH inhibition also induced Bim expression in both allografts and CD4+ T cells (Figure 5C,D). Bim expression and activity are regulated at the transcriptional, translational, and post-translational levels, and act as an apoptotic activator to ensure tissue integrity. The increased Bim expression suggests that SAHH inhibition may induce apoptosis during alloimmune responses.
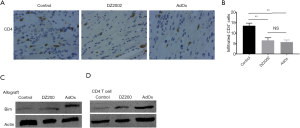
Discussion
In this study, we investigated the immunosuppressive effects of reversible (DZ2002) and irreversible (AdOx) SAHH inhibitors in a BALB/C to C57B/6 mouse cardiac transplantation model. Both types of SAHH inhibitors markedly prolonged the survival time of cardiac allografts. The therapeutic effects of reversible SAHH inhibitors suppressed pathogenic Th1/Th17 development and the activation of CD4+ T cells, induced apoptosis of activated CD4+ T cells, and prolonged cardiac allograft viability. The reversible SAHH inhibitor (DZ2002) shows a more promising potential due to its mild cytotoxicity.
SAH is a SAM-dependent transmethylase inhibitor of SAHH, which induces SAH accumulation that can lead to hypotransmethyl activity. The activation and proliferation of lymphocytes are dependent on SAM-mediated transmethylation reactions (7). Transmethylation inhibition by SAHH inhibitors can lead to immunosuppression and CD4+ T cell-mediated alloimmunity. Thus, DZ2002 and AdOx were used to assess the therapeutic potential of SAHH inhibition in a solid organ transplantation model. A murine HTx model was used to assess the immunosuppressive effects of SAHH inhibitors in cardiac transplantation. The results demonstrated that both types of SAHH inhibitors significantly prolonged cardiac allograft survival. Allograft injury was greatly reduced 7 days post-transplantation, CD4+IFNγ+ subsets, and CD4+IL17A+ subsets were decreased with both DZ2002 and AdOx treatment, and cardiac allograft infiltrated CD4+ T cells were also decreased. SAHH inhibition decreased SAHH activity resulting in a dose-dependent elevation of plasma SAH levels (8,9). Compared with AdOx, DZ2002 was less potent in inhibiting immune responses, but DZ2002 was more likely to have dose-dependent effects.
SAHH inhibition may lead to unexpected effects as SAHH is widely distributed throughout the body. AdOx induces G2/M arrest and apoptosis in vitro, as well as inhibits NF-κB (10). DZ2002 affects antigen-presenting cell (APC) function by reducing surface expression of a number of glycoproteins, including CD80 and CD86 (5). APCs are also critical in transplant rejection for inducing an immune response in cardiac rejection. Antiviral activities of the SAHH inhibitors are partly attributed to their inhibition of SAHH effects (10). CD4+ T cell-mediated immune transmethylation is required for CD4+ T cell activation, and methylation is important during CD4+ T cell activation (11-13). All these combined effects may help to explain the potent effects of SAHH inhibitors, especially AdOx, in cardiac transplantation.
CD44 is an indicative marker for effector-memory T cells, which also express CD62L. Effector-memory T cells circulate in the periphery and have immediate effector functions upon encountering alloantigens. Our results show that both types of inhibitor decreased the expression of CD4+CD44+ and CD4+CD44+CD62L− T cells on day 7 post-transplantation. The SAHH inhibitors decreased the activation of CD4+ and CD8+ T cells, both of which play important roles in allograft rejection. Our results also suggest that SAHH inhibitors may induce apoptosis. Possibly, SAHH inhibitors hinder the activation of CD4+ T cells and, at the same time, induce apoptosis (14). Coordinated expression and activation of Bim shape immune responses and ensure tissue integrity (15,16). Hildeman et al. (17) provided evidence that activated lymph cell death in vivo is mediated by the Bcl-2 family member Bim, albeit at slightly higher SAHH inhibitor concentration than the present study. When CD4+ T cells respond to an allograft in vivo, in association with APCs, they become activated. Our results show that SAHH inhibitors decreased the activation of CD4+ T cells and induced apoptosis in CD4+ T cells. Apoptosis of alloreactive CD4+ T cells is important for immune homeostasis. The relationship between apoptosis and decreased activation of CD4+ T cells might interact as both cause and effect.
SAHH inhibitors’ toxicity may be an obstacle to their clinical application. Many studies have indicated cytotoxicity of SAHH inhibitors, especially irreversible inhibitors, whereas the reversible SAHH inhibitor DZ2002 is safer. Mice treated for 9 months with daily i.p. injections of DZ2002 (50 mg/kg/day) showed no signs of severe toxicity (18). Signaling defects caused by DZ2002 were restricted to CD4+ T cells. However, in our study, mice treated with AdOx (10 mg/kg/day) showed profound weight loss. High concentrations of AdOx led to a previously unknown form of cell death, which was characterized by distinct, caspase-independent alterations of the cell shape, including a marked protuberation of the nucleus, cytoplasmic extensions, actin aggregation, and incomplete chromatin condensation (19). Experimental evidence suggests that the reversible SAHH inhibitor (DZ2002) is much less toxic than irreversible SAHH inhibitors as the cytotoxicity of DZ2002 was 16- to 40-fold lower than irreversible SAHH inhibitor while conserving its potent immunosuppressive functions (5).
SAHH inhibitors inevitably block other transmethylation reactions in all cells, especially irreversible SAHH inhibitors (12,18). Theoretically, reversible SAHH inhibitors irreversibly reduce the enzymatic activity of SAHH. The toxicity and narrow therapeutic windows of SAHH inhibitors are also a barrier for their clinical application. Effective and toxic doses are unknown in SAHH inhibitors and need to be established before being used in the clinics. Type III SAHH inhibitors seem to be more promising due to their reversible binding to the open form of SAHH and their safety. Thus, future research efforts should focus on the development of safe, reversible SAHH inhibitors. AdOx was found to be more potent than DZ2002 as the inhibitory effect of AdOx on SAHH activity was maximal at 4 h and still apparent after 72 h of incubation (20). The apparent oral bioavailability of DZ2002 was 90–159%, and the mean terminal half-lives of DZ2002 and were 0.3–0.9 and 1.3–5.1 h (21). With improvements in the efficacy and specificity of existing agents, it is increasingly challenging to find new targets that meet patient’s needs. Reversible SAHH inhibitors present a promising immunosuppression therapy through the inhibition of cellular transmethylations.
In summary, the effects of reversible and irreversible SAHH inhibitors in a cardiac transplantation model were assessed. DZ2002, a reversible SAHH inhibitor, exhibits low toxicity and compelling immunosuppressive effects while irreversible SAHH inhibitor AdOx was also potent in immunosuppression but had relatively severe toxicity. Both inhibitors were effective in maintaining inflammatory factors, reducing CD4+ T cell activation, and inducing CD4+ T cell apoptosis. We believe that the reversible SAHH inhibitors represent a promising immunosuppression therapy that could serve as a possible complementary therapeutic agent in transplantation.
Acknowledgments
Funding: Supported by the National Natural Science Foundation of China (grant numbers 81671578).
Footnote
Reporting Checklist: The authors have completed the ARRIVE reporting checklist. Available at http://dx.doi.org/10.21037/atm-20-2899
Data Sharing Statement: Available at http://dx.doi.org/10.21037/atm-20-2899
Conflicts of Interest: All authors have completed the ICMJE uniform disclosure form (available at http://dx.doi.org/10.21037/atm-20-2899). The authors have no conflicts of interest to declare.
Ethical Statement: The authors are accountable for all aspects of the work in ensuring that questions related to the accuracy or integrity of any part of the work are appropriately investigated and resolved. Experiments were performed under a project license (IACUC Number: 2358) granted by the Tongji Medical College, HUST Institutional Animal Care and Use Committee, in compliance with institutional guidelines for the care and use of animals.
Open Access Statement: This is an Open Access article distributed in accordance with the Creative Commons Attribution-NonCommercial-NoDerivs 4.0 International License (CC BY-NC-ND 4.0), which permits the non-commercial replication and distribution of the article with the strict proviso that no changes or edits are made and the original work is properly cited (including links to both the formal publication through the relevant DOI and the license). See: https://creativecommons.org/licenses/by-nc-nd/4.0/.
References
- Belužić L, Grbeša I, Belužić R, et al. Knock-down of AHCY and depletion of adenosine induces DNA damage and cell cycle arrest. Sci Rep 2018;8:14012. [Crossref] [PubMed]
- Luo M. Chemical and Biochemical Perspectives of Protein Lysine Methylation. Chem Rev 2018;118:6656-705. [Crossref] [PubMed]
- Yuan CS, Saso Y, Lazarides E, et al. Recent advances in S-adenosyl-L-homocysteine hydrolase inhibitors and their potential clinical applications. Expert Opinion on Therapeutic Patents 1999;9:1197-206.
- Borchardt RT, Patel UG, Bartel RL. Adenosine dialdehyde: a potent inhibitor of S-adenosylhomocysteine hydrolase. Biochemistry of S-adenosylmethionine and related compounds. Springer, 1982:645-52.
- Wu QL, Fu YF, Zhou WL, et al. Inhibition of S-adenosyl-L-homocysteine hydrolase induces immunosuppression. J Pharmacol Exp Ther 2005;313:705-11. [Crossref] [PubMed]
- Ding X, Chen J, Wu C, et al. Nucleotide-Binding Oligomerization Domain-Like Receptor Protein 3 Deficiency in Vascular Smooth Muscle Cells Prevents Arteriovenous Fistula Failure Despite Chronic Kidney Disease. J Am Heart Assoc 2019;8:e011211. [Crossref] [PubMed]
- German DC, Bloch CA, Kredich NM. Measurements of S-adenosylmethionine and L-homocysteine metabolism in cultured human lymphoid cells. J Biol Chem 1983;258:10997-1003. [PubMed]
- Xiao Y, Xia J, Cheng J, et al. Inhibition of S-Adenosylhomocysteine Hydrolase Induces Endothelial Dysfunction via Epigenetic Regulation of p66shc-Mediated Oxidative Stress Pathway. Circulation 2019;139:2260-77. [Crossref] [PubMed]
- He SJ, Lin ZM, Wu YW, et al. Therapeutic effects of DZ2002, a reversible SAHH inhibitor, on lupus-prone NZB×NZW F1 mice via interference with TLR-mediated APC response. Acta Pharmacol Sin 2014;35:219-29. [Crossref] [PubMed]
- Dasgupta A, Jung KJ, Jeong SJ, et al. Inhibition of methyltransferases results in induction of g2/m checkpoint and programmed cell death in human T-lymphotropic virus type 1-transformed cells. J Virol 2008;82:49-59. [Crossref] [PubMed]
- Madhavan GV, McGee DP, Rydzewski RM, et al. Synthesis and antiviral evaluation of 6'-substituted aristeromycins: potential mechanism-based inhibitors of S-adenosylhomocysteine hydrolase. J Med Chem 1988;31:1798-804. [Crossref] [PubMed]
- LaMere SA, Thompson RC, Meng X, et al. H3K27 Methylation Dynamics during CD4 T Cell Activation: Regulation of JAK/STAT and IL12RB2 Expression by JMJD3. J Immunol 2017;199:3158-75. [Crossref] [PubMed]
- Antignano F, Zaph C. Regulation of CD4 T-cell differentiation and inflammation by repressive histone methylation. Immunol Cell Biol 2015;93:245-52. [Crossref] [PubMed]
- Campbell KJ, Tait SWG. Targeting BCL-2 regulated apoptosis in cancer. Open Biol 2018;8:180002. [Crossref] [PubMed]
- O'Connor L, Strasser A, O'Reilly LA, et al. Bim: a novel member of the Bcl-2 family that promotes apoptosis. EMBO J 1998;17:384-95. [Crossref] [PubMed]
- Labi V, Bertele D, Woess C, et al. Haematopoietic stem cell survival and transplantation efficacy is limited by the BH3-only proteins Bim and Bmf. EMBO Mol Med 2013;5:122-36. [Crossref] [PubMed]
- Hildeman DA, Zhu Y, Mitchell TC, et al. Activated T cell death in vivo mediated by proapoptotic bcl-2 family member bim. Immunity 2002;16:759-67. [Crossref] [PubMed]
- Lawson BR, Manenkova Y, Ahamed J, et al. Inhibition of transmethylation down-regulates CD4 T cell activation and curtails development of autoimmunity in a model system. J Immunol 2007;178:5366-74. [Crossref] [PubMed]
- Schwerk C, Schulze-Osthoff K. Methyltransferase inhibition induces p53-dependent apoptosis and a novel form of cell death. Oncogene 2005;24:7002-11. [Crossref] [PubMed]
- O'Dea RF, Mirkin BL, Hogenkamp HP, et al. Effect of adenosine analogues on protein carboxylmethyltransferase, S-adenosylhomocysteine hydrolase, and ribonucleotide reductase activity in murine neuroblastoma cells. Cancer Res 1987;47:3656-61. [PubMed]
- Jia W, Li J, Du F, et al. Assay development for determination of DZ2002, a new reversible SAHH inhibitor, and its acid metabolite DZA in blood and application to rat pharmacokinetic study. J Pharm Anal 2019;9:25-33. [Crossref] [PubMed]