This article has an erratum available at: http://dx.doi.org/10.21037/atm-2021-22 the article has been update on 2021-07-22 at here.
Delivery of vascular endothelial growth factor (VEGFC) via engineered exosomes improves lymphedema
Introduction
Lymphedema is a common postoperative complication of cancer or a result of a parasitic infection (1-3). Lymphedema is caused by the aberrant accumulation of interstitial fluid, which results in swelling and disability, mostly in the extremities (4). There are 140–250 million patients with limb lymphedema worldwide. Rebuilding the lymphatic duct is suggested to be crucial to treat lymphedema. Lymphatic venous anastomosis and vascularized lymph node transfer are considered the critical approaches for lymphedema (5). However, lymphatic venous anastomosis often results in lymphatic occlusion, and rebuilding of the communication between the new lymph node and the original lymphatic system remains a significant challenge (6-9). Therefore, novel strategies still must improve the therapy of lymphedema.
Mesenchymal stem cells (MSCs) are adult stem cells with multidirectional differentiation and self-renewal and have been widely used in the treatment of many diseases (10-12). It has been reported that adipose tissue-derived stem cells (ADSCs) play an essential role in lymphangiogenesis (13,14). However, treatment with MSCs often causes a series of cell-related side effects, including changes in disease phenotypes and uncontrolled implanted cells, thus limiting its clinical application. Exosomes are a group of small membrane-bound vesicles (30–120 nm in diameter) secreted by multiple cell types. Exosomes are necessary communication tools between cells, which transfer mRNAs, miRNAs, and proteins to recipient cells and thus modulate their activities (15,16). Some note that exosomes derived from MSCs that can mimic the biological functions of MSCs and have a series of therapeutic effects on tissue regeneration, immune regulation, and reduction of tissue degeneration (10-13,16). MSCs derived-exosomes have been applied to research on myocardial infarction, stroke, limb ischemia, hypoxic-ischemic brain injury, kidney injury, and cartilage injury (15-19). However, the effect of MSC exosomes on lymphedema is still unknown.
Vascular endothelial growth factor (VEGFC) plays a critical role in the growth of lymphatic endothelial cells and is proposed to be a potential target for lymphedema treatment (20-23). However, how to deliver VEGFC to target cells remains a major issue. Engineered exosomes as endogenous delivery carriers exhibit low immunogenicity, can protect the activity and stability of proteins or nucleic acid molecules, and prolong the half-life of drugs compared with other carriers (15-19). In this study, we developed a strategy to produce engineered ADSC-exos for VEGFC delivery. To improve the loading of VEGFC in exosomes, VEGFC was fused with CD63, and the fusion construct was transfected into ADSCs. Engineered exosomes were then isolated, and the effects of CD63-VEGFC-exos on the proliferation, migration, and tube formation of lymphatic endothelial cells and lymphedema were investigated.
We present the following article in accordance with the ARRIVE reporting checklist (available at http://dx.doi.org/10.21037/atm-20-6605).
Methods
Experiments were performed under a project license (SYXK-2020-0233) granted by The First Affiliated Hospital of Sun Yat-sen University, in compliance with Chinese national or institutional guidelines for the care and use of animals.
Isolation of ADSCs and cell culture
Human adipose tissue-derived stem cells were purchased from Guangzhou Cyagen Biology. ADSCs are cultured in DMEM-low glucose, holding 10% fetal bovine serum (FBS). Human dermal lymphatic endothelial cells (HDLECs) were obtained from the Cell Bank of the Chinese Academy of Sciences (Shanghai, China) and kept in DMEM medium, holding 10% fetal FBS. All cells are cultured at 37 °C with 5% CO2.
Extraction and characterization of ADSC exosomes
DMEM/F12 medium was centrifuged at 100,000 g for 16 hours to prepare the exosome-free medium. ADSCs were cultured in an exosome-free medium for 48 hours. After infection, with lentivirus expressing different constructs, the conditioned medium was collected for exosome extraction. The medium was centrifuged at 3,000 g for 15 minutes, 2,000 g for 30 minutes, and 100,000 g for 70 minutes. The pellet was washed once with PBS and centrifuged again at 100,000 g for another 70 minutes. After fixation with 3% glutaraldehyde for 2 hours, exosomes were stained with 2% uranyl acetate for 30 seconds. The morphology of the exosome was analyzed by the transmission electron microscopy (TEM, Zeiss, Jena, Germany).
The uptake of exosomes
The exosomes were labeled with PKH67 to assess the uptake of exosomes by HDLECs (Sigma, USA). Then, 2 µg of PKH67-stained exosomes were added to 6-well plates holding 1.5×105 HDLECs. Twenty-four hours later, cells were incubated with 4% paraformaldehyde for 20 minutes, and nuclei were stained with DAPI. The uptake of exosomes was visualized by a confocal microscope (Zeiss, Jena, Germany).
CCK-8 assay
The CCK-8 assay was used to determine the effect of exosomes on the proliferation of HDLECs. Cells were seeded in 96-well plates and culture overnight. Cells were treated with ADSC-exos (10 µg/mL). After culturing for 24, 48, and 72 hours, a CCK-8 reagent was added to the plates. Then, the OD value was measured at a wavelength of 450 nm on a microplate reader.
Migration assay
HDLECs in 100 µL medium were seeded in the upper chamber of 24-well Transwell plates to observe the effect of ADSC-exos on HDLEC migration (Corning, USA). Then, ADSC-exos (10 µg/mL) in 600 µL medium containing 1% FBS were added at the lower chamber. After incubation for 24 hours, cells on the top of the filter were swabbed off, and cells on the bottom were fixed in 4% paraformaldehyde and then stained with 0.05% crystal violet. Three random fields were selected, and the number of cells was counted.
Quantitative RT-PCR (qRT-PCR) analysis
HDLECs were treated with 10 µg/mL ADSC-exos for 48 hours, and then total RNA of HDLECs was extracted using Trizol reagent (Life science, USA). RNA was transcribed to be cDNA using the Thermo Scientific RevertAid, First Strand, cDNA Synthesis Kit (Thermo), and PCR was performed using SYBR Green (Tiangen Biotech, Beijing, China). The PCR conditions were: 95 °C for 30 seconds, 40 cycles of 95 °C for 15 seconds, and 60 °C for 30 seconds. Glyceraldehyde 3-phosphate dehydrogenase (GAPDH) serves as an internal control. The relative mRNA expression was calculated using the 2–ΔΔCT method.
Immunofluorescence assay
Cells were fixed with 4% PFA and permeabilized with 0.5% Triton X-100 diluted in PBS for 10 minutes. Then, the cells were stained with DAPI and observed under a fluorescence microscope (Zeiss, Jena, Germany).
Construction of ADSC-exos-alginate
Sodium alginate powder was dissolved in PBS to prepare a 2% (w/v) sodium alginate solution. Then 2% sodium alginate solution containing ADSC-exos (8 µg/mL) is mixed with 2% (w/v) calcium chloride solution and stirred gently to form ADSC-exos-alginate gels (control/exos-alginate, vector/exos-alginate, VEGFC-exos-alginate, and CD-63-VEGFC-exos-alginate).
The release of ADSC-exos from alginate hydrogel
The release ratio of ADSC-exos in alginate hydrogel was tested with the fluorescent signal, as described previously (24). Briefly, ADSC-exos were stained with ExoGlow-RNA EV Labeling kit (System Biosciences). Then, 100 µL alginate hydrogels with 80 µg ADSC-exos were plated in a 96-well plate holding 100 µL PBS. After incubation at 37 °C for 0, 1, 3, 6, 12, and 24 hours, the supernatant was collected, and the fluorescence of the supernatant was detected. A standard curve of exosome concentration was set up for the gradient concentration of labeled ADSC-exos from 0.15–10 µg in 100 µL PBS. Then the fluorescent signal determines the released exosome according to the standard curve.
Establishment of mouse tail lymphedema model
The mouse tail lymphedema model was established as described before (25). Intraperitoneal injection anesthetized the 6-week-old C57BL/6J female mouse with 8% chloral hydrate. After anesthesia, a circular incision was made at 1 cm from the base of the tail. Then, the skin of the tail was removed (~2 mm in deep). The major lymphatic trunks were found through a distal intradermal injection of 5 µL of methylene blue. Under an operating microscope, the blue-stained lymphatic vessels were removed, and the cut ends, and the skin of the wound margin was cauterized to prevent the lymphatic vessels from re-canalizing.
Immunohistochemistry
Sections were prepared from the injured distal tissues of mice, incubated with 3% hydrogen peroxide in methanol for 30 minutes to block the endogenous peroxidase, and boiled in a citrate buffer for antigen retrieval. After blocking with 10% goat serum, the sections were incubated with the primary antibody at 4 °C overnight. Then the sections were incubated with biotinylated goat-anti-rabbit secondary antibody for 30 minutes at room temperature. A horseradish peroxidase-streptavidin reagent (ImmunoCruz system, Santa Cruz Biotechnology) was used to develop the color. Then the sections were counter-stained with hematoxylin to visualize the nuclei.
Statistical analysis
All data were presented as mean ± SD. A comparison among distinct groups was performed using the one-way ANOVA. Statistical significance was considered the P value lower than 0.05.
Results
CD63 improves GFP expression on the membrane
To find the ideal elements that could improve the loading of target molecules, GFP, a green fluorescent protein, was the endogenous cargo target. Two membrane proteins (PB, Palm) and a transmembrane protein (CD63) were cloned into the lentiviral vector pLVX-GFP to produce fused proteins CD63-GFP, GFP-PB, and Palm-GFP (Figure 1A). 293T cells are transduced with lentivirus expressing different fused constructs. Immunofluorescence staining showed that the density of GFP on the membrane was highest in the CD63 group, followed by PB and Palm groups (Figure 1B). Then, exosomes were isolated from 293T cells transduced with different lentiviruses (Figure 1C,D). Western blot analysis showed that CD63/exos had the highest level of GFP, followed by PB/exos and Palm/exos (Figure 1E). Therefore, CD63 was selected as the element for further construction of exosomes.
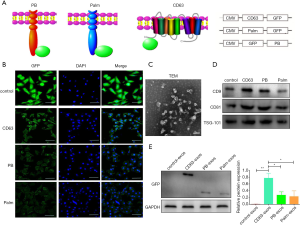
Engineered exosomes enhance the loading of VEGFC
VEGFC is a critical regulator in lymphedema (20-23). To efficiently deliver VEGFC to the target cells via exosomes, we fused VEGFC to the C-terminal of CD63 and then subcloned to the pLVX-GFP vector (Figure 2A). ADSCs stably expressing CD63-VEGFC were set up by transduction with lentivirus (Figure 2B). A CCK-8 assay showed that lentivirus infection did not affect the viability of ADSCs (Figure 2C). The morphology of exosomes extracted from ADSCs was verified by TEM (Figure 2D). Also, the expression of exosome markers (CD9, CD91, TSG-101) was confirmed by a Western blot test (Figure 2E). When examining the loading of VEGFC in exosomes, we found the protein level of VEGFC was significantly greater in CD63-VEGFC/exos compared with the control groups (control/exos, vector/exos, and VEGFC/exos) (Figure 2F). Together, these data showed that engineered exosomes could effectively increase the loading of VEGFC.
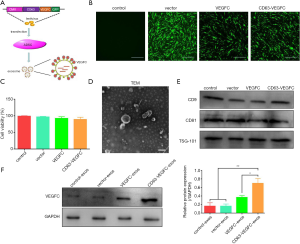
Engineered exosomes promote the proliferation, migration, and tube formation of lymphatic endothelial cells
First, we examined whether HDLECs could take up ADSC-exos. ADSC-exos were labeled with PKH67 dye and then incubated with HDLECs. Immunofluorescence staining showed that several green spots were observed in HDLECs (Figure 3A), suggesting uptake of ADSC-exos. Viability analysis revealed that treatment with CD63-VEGFC/exos resulted in a significant increase in the growth of HDLECs compared with the controls (control/exos, vector/exos, and VEGFC/exos) (Figure 3B). A Transwell migration assay also showed CD63-VEGFC/exos remarkably elevated the migration of HDLECs, which was followed by VEGFC/exos, vector/exos, and control/exos (Figure 3C). HDLECs treated with CD63-VEGFC/exos formed more tubes compared with the controls (control/exos, vector/exos, and VEGFC/exos) (Figure 3D). The mRNA and protein expressions of lymph tube markers (PROX1, LYEV-1, ERK and p-ERK) were greatly increased after treatment with CD63-VEGFC/exos compared with the controls (control/exos, vector/exos, and VEGFC/exos) (Figure 3E,F, Figure S1). Together, these results showed that CD63-VEGFC/exos have a positive effect on lymphatic endothelial cell proliferation, migration, and tube formation.
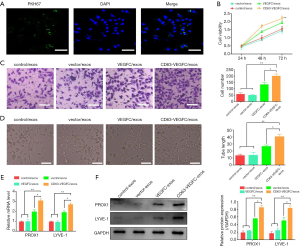
Engineered exosomes improve lymphedema in a mouse model
To immobilize the engineered exosomes to the injury area, we first embedded the CD63-VEGFC/exos into the alginate hydrogel (Figure 4A). CD63-VEGFC/exos were labeled with the ExoGlow RNA probe, and the number of exosomes was determined by fluorescence density. The result showed that the incubating time increased, CD63-VEGFC/exos gradually released from the alginate hydrogel (Figure 4B).
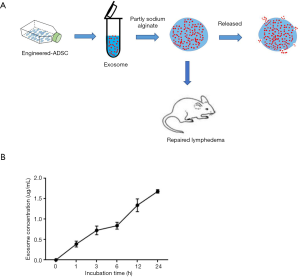
We then investigated whether CD63-VEGFC/exos embedded in alginate hydrogel had a beneficial effect on lymphedema. An acquired mouse tail lymphedema model was set up via microsurgical ablation. Engineered exosomes embedded in alginate hydrogel were injected into the subcutaneous edema area of the mouse tail. Forty-two days after the operation, the diameter of the mouse tail was measured. The result showed that treatment with CD63-VEGFC/exos-alginate markedly reduced the diameter of the mouse tail compared with the controls (Figure 5A). The mRNA and protein levels of lymph tube markers (PROX1 and LYEV-1) were also significantly increased in the CD63-VEGFC/exos-alginate group compared with the control groups, as showed by qRT-PCR, immunohistochemistry, and Western blot (Figure 5B,C,D). Collectively, these data showed that engineered CD63-VEGFC/exos could improve lymphedema in vivo.
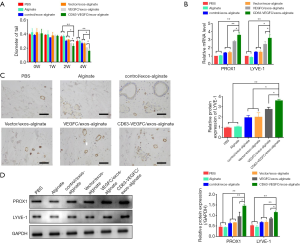
Discussion
Reconstruction of the structure and function of the endogenous lymphatic system is vital for the effective treatment of lymphedema. However, strategies that can improve the reconstruction are still lacking. In the present study, we developed a strategy to produce engineered exosomes, which simultaneously deliver VEGFC to improve lymphatic reconstruction and thus enhance the treatment of lymphedema.
Lymphatic vessel formation is a complicated process that involves the proliferation, migration, and tube formation of lymphatic endothelial cells. Several chemokines, growth factors, adhesion molecules, and extracellular matrix regulators are implicated in this process. Among them, VEGFC is considered the primary modulator. It has been shown that VEGFC promotes the differentiation of lymphatic endothelial cells and thus the formation of lymphatic vessels (20-23). Animal studies have also shown that lentivirus or adenovirus-mediated overexpression of VEGFC improves lymphedema (26). However, the instability/degradation of VEGFC, low delivery efficiency, and the short residence rate, limiting the efficacy. Therefore, developing an efficient delivery strategy to transport VEGFC to target cells is significant in lymphatic vessel regeneration.
Exosomes derived from MSCs are suggested to be a valuable tool for target delivery and display therapeutic potential in many diseases. For example, it has been reported that ADSC-secreted exosomes with polypyrrole titanium significantly enhance osteogenesis in vivo (27). In a diabetic model, the delivery of SPION-conjugated BAY55-9837 by exosomes significantly increases the secretion of insulin and improves the treatment of type 2 diabetes mellitus (28). These results show that exosomes have potential applications as transport carriers for targeted therapies. However, cargo loading is still a significant challenge for exosome-based delivery. There are two significant ways of loading drugs/targets to exosomes: electroporation/ultrasound and exosome formation (29). Exosomes from different cell types have drug/target selectivity. Thus, only overexpressing specific molecules may not improve the loading. Many studies have revealed that exosome membranes are originated from cell membranes. Thus, co-overexpression of membrane proteins, including CD9, CD63, Lamp2, could positively enrich RNA and proteins in exosomes and improve the delivery efficiency (30-32). Consistently, we showed that fusing VEGFC with CD63 could significantly increase the expression of VEGFC in ADSC-exos. CD63-VEGFC-exos could significantly enhance the proliferation, migration, and tube formation of lymphatic endothelial cells.
Although exosomes play a vital role in drug delivery, low retention rates and short half-life remain significant challenges. Incorporation of exosomes in hydrogel appears as a promising strategy against these problems. Alginate is a type of natural linear polysaccharide extracted from brown algae and has been widely used in drug delivery and tissue engineering because of its biocompatibility and similarity with the extracellular matrix (33,34). For instance, the delivery of MSC-derived small extracellular vesicles (sEVs) embedded in alginate hydrogel highly improves cardiac function (35). Sequential delivery of VEGF-A (165), followed by PDGF-BB with alginate hydrogels, improves angiogenic effect (36). In agreement with these studies, we found that incorporating CD63-VEGFC/exos in sodium alginate hydrogel reduced mouse lymphedema substantially. Therefore, incorporation of exosomes carrying VEGFC in alginate hydrogel might offer a novel strategy for the therapy of lymphedema. However, the stability and safety of CD63-VEGFC/exos are still needed to be clarified in future studies before further applications.
Conclusions
In this study, we reported, for the first time, the effect of engineered ADSC-exos in the treatment of lymphedema. Our data showed that CD63-VEGFC/exos remarkably promoted the proliferation, migration, and tube formation of lymphatic endothelial cells. Incorporation of CD63-VEGFC/exos in sodium alginate hydrogel significantly improved lymphedema in mice. Our findings supply a novel ADSC-exo-based strategy for treating lymphedema.
Acknowledgments
We thank all of the members in the lab. We are grateful to the financial support of the National Natural Science Foundation.
Funding: This work was financially supported by the National Natural Science Foundation of China (8187020594).
Footnote
Reporting Checklist: The authors have completed the ARRIVE reporting checklist. Available at http://dx.doi.org/10.21037/atm-20-6605
Data Sharing Statement: Available at http://dx.doi.org/10.21037/atm-20-6605
Conflicts of Interest: All authors have completed the ICMJE uniform disclosure form (available at http://dx.doi.org/10.21037/atm-20-6605). The authors have no conflicts of interest to declare.
Ethical Statement: The authors are accountable for all aspects of the work in ensuring that questions related to the accuracy or integrity of any part of the work are appropriately investigated and resolved. The procedure was proved by the Research Ethics Committee of the First Affiliated Hospital of Sun Yat-sen University. All the volunteers provided informed consent. Experiments were performed under a project license (SYXK-2020-0233) granted by The First Affiliated Hospital of Sun Yat-sen University, in compliance with Chinese national or institutional guidelines for the care and use of animals.
Open Access Statement: This is an Open Access article distributed in accordance with the Creative Commons Attribution-NonCommercial-NoDerivs 4.0 International License (CC BY-NC-ND 4.0), which permits the non-commercial replication and distribution of the article with the strict proviso that no changes or edits are made and the original work is properly cited (including links to both the formal publication through the relevant DOI and the license). See: https://creativecommons.org/licenses/by-nc-nd/4.0/.
References
- Dessources K, Aviki E, Leitao MM Jr. Lower extremity lymphedema in patients with gynecologic malignancies. Int J Gynecol Cancer 2020;30:252-60. [Crossref] [PubMed]
- Gillespie TC, Sayegh HE, Brunelle CL, et al. Breast cancer-related lymphedema: risk factors, precautionary measures, and treatments. Gland Surg 2018;7:379-403. [Crossref] [PubMed]
- Wu R, Huang X, Dong X, et al. Obese patients have higher risk of breast cancer-related lymphedema than overweight patients after breast cancer: a meta-analysis. Ann Transl Med. 2019;7:172. [Crossref] [PubMed]
- Nimir M, Abdelrahim M, Abdelrahim M, et al. In silico analysis of single nucleotide polymorphisms (SNPs) in human FOXC2 gene. F1000Res 2017;6:243. [Crossref] [PubMed]
- Li P, He B, Yang Y, et al. Short-term outcome of vascularized supraclavicular lymph nodes flap transplantation to treat the lower extremity lymphedema. Chinese Journal of Microsurgery 2017;40:218.
- Garza RM, Chang DW. Lymphovenous bypass for the treatment of lymphedema. J Surg Oncol 2018;118:743-9. [Crossref] [PubMed]
- Mazerolle P, Meresse T, Gangloff D, et al. Vascularized lymph node transfer with submental free flap. Eur Ann Otorhinolaryngol Head Neck Dis 2020;137:73-7. [Crossref] [PubMed]
- Yang Y, Chen XH, Li FG, et al. In vitro induction of human adipose-derived stem cells into lymphatic endothelial-like cells. Cell Reprogram 2015;17:69-76. [Crossref] [PubMed]
- Yang Y, Yang JT, Chen XH, et al. Construction of tissue-engineered lymphatic vessel using human adipose derived stem cells differentiated lymphatic endothelial like cells and decellularized arterial scaffold: A preliminary study. Biotechnol Appl Biochem 2018;65:428-34. [Crossref] [PubMed]
- Zhou Y, Xu W, Shao A. Application Prospect of Mesenchymal Stem Cells in the Treatment of Sepsis. Crit Care Med 2020;48:e634. [Crossref] [PubMed]
- Jia Y, Shu X, Yang X, et al. Enhanced therapeutic effects of umbilical cord mesenchymal stem cells after prolonged treatment for HBV-related liver failure and liver cirrhosis. Stem Cell Res Ther 2020;11:277. [Crossref] [PubMed]
- Sabry D, Marzouk S, Zakaria R, et al. The effect of exosomes derived from mesenchymal stem cells in the treatment of induced type 1 diabetes mellitus in rats. Biotechnol Lett 2020;42:1597-610. [Crossref] [PubMed]
- Ahmadzadeh N, Robering JW, Kengelbach-Weigand A, et al. Human adipose-derived stem cells support lymphangiogenesis in vitro by secretion of lymphangiogenic factors. Exp Cell Res 2020;388:111816. [Crossref] [PubMed]
- Takeda K, Sowa Y, Nishino K, et al. Adipose-derived stem cells promote proliferation, migration, and tube formation of lymphatic endothelial cells in vitro by secreting lymphangiogenic factors. Ann Plast Surg 2015;74:728-36. [Crossref] [PubMed]
- Liu L, Jin X, Hu CF, et al. Exosomes Derived from Mesenchymal Stem Cells Rescue Myocardial Ischaemia/Reperfusion Injury by Inducing Cardiomyocyte Autophagy Via AMPK and Akt Pathways. Cell Physiol Biochem 2017;43:52-68. [Crossref] [PubMed]
- Lai RC, Arslan F, Lee MM, et al. Exosome secreted by MSC reduces myocardial ischemia/reperfusion injury. Stem Cell Res 2010;4:214-22. [Crossref] [PubMed]
- Goetzl L, Merabova N, Darbinian N, et al. Diagnostic Potential of Neural Exosome Cargo as Biomarkers for Acute Brain Injury. Ann Clin Transl Neurol 2017;5:4-10. [Crossref] [PubMed]
- Jin J, Shi Y, Gong J, et al. Exosome secreted from adipose-derived stem cells attenuates diabetic nephropathy by promoting autophagy flux and inhibiting apoptosis in podocyte. Stem Cell Res Ther 2019;10:95. [Crossref] [PubMed]
- Toh WS, Lai RC, Hui JHP, et al. MSC exosome as a cell-free MSC therapy for cartilage regeneration: Implications for osteoarthritis treatment. Semin Cell Dev Biol 2017;67:56-64. [Crossref] [PubMed]
- Foster RR, Armstrong L, Baker S, et al. Glycosaminoglycan regulation by VEGFA and VEGFC of the glomerular microvascular endothelial cell glycocalyx in vitro. Am J Pathol 2013;183:604-16. [Crossref] [PubMed]
- Dufies M, Giuliano S, Ambrosetti D, et al. Sunitinib Stimulates Expression of VEGFC by Tumor Cells and Promotes Lymphangiogenesis in Clear Cell Renal Cell Carcinomas. Cancer Res 2017;77:1212-26. [Crossref] [PubMed]
- Zhou J, He Z, Guo L, et al. MiR-128-3p directly targets VEGFC/VEGFR3 to modulate the proliferation of lymphatic endothelial cells through Ca(2+) signaling. Int J Biochem Cell Biol 2018;102:51-8. [Crossref] [PubMed]
- Gauvrit S, Villasenor A, Strilic B, et al. HHEX is a transcriptional regulator of the VEGFC/FLT4/PROX1 signaling axis during vascular development. Nat Commun 2018;9:2704. [Crossref] [PubMed]
- Zhang K, Zhao X, Chen X, et al. Enhanced Therapeutic Effects of Mesenchymal Stem Cell-Derived Exosomes with an Injectable Hydrogel for Hindlimb Ischemia Treatment. ACS Appl Mater Interfaces 2018;10:30081-91. [Crossref] [PubMed]
- Jang DH, Song DH, Chang EJ, et al. Anti-inflammatory and lymphangiogenetic effects of low-level laser therapy on lymphedema in an experimental mouse tail model. Lasers Med Sci 2016;31:289-96. [Crossref] [PubMed]
- Kim JD, Jin SW. A tale of two models: mouse and zebrafish as complementary models for lymphatic studies. Mol Cells 2014;37:503-10. [Crossref] [PubMed]
- Chen L, Mou S, Li F, et al. Self-Assembled Human Adipose-Derived Stem Cell-Derived Extracellular Vesicle-Functionalized Biotin-Doped Polypyrrole Titanium with Long-Term Stability and Potential Osteoinductive Ability. ACS Appl Mater Interfaces 2019;11:46183-96. [Crossref] [PubMed]
- Zhuang M, Du D, Pu L, et al. SPION-Decorated Exosome Delivered BAY55-9837 Targeting the Pancreas through Magnetism to Improve the Blood GLC Response. Small 2019;15:e1903135. [Crossref] [PubMed]
- Armstrong JPK, Stevens MM. Strategic design of extracellular vesicle drug delivery systems. Adv Drug Deliv Rev 2018;130:12-6. [Crossref] [PubMed]
- Wang Q, Yu J, Kadungure T, et al. ARMMs as a versatile platform for intracellular delivery of macromolecules. Nat Commun 2018;9:960. [Crossref] [PubMed]
- Liang G, Zhu Y, Ali DJ, et al. Engineered exosomes for targeted co-delivery of miR-21 inhibitor and chemotherapeutics to reverse drug resistance in colon cancer. J Nanobiotechnology 2020;18:10. [Crossref] [PubMed]
- Kojima R, Bojar D, Rizzi G, et al. Designer exosomes produced by implanted cells intracerebrally deliver therapeutic cargo for Parkinson's disease treatment. Nat Commun 2018;9:1305. [Crossref] [PubMed]
- Wang P, Song Y, Weir MD, et al. A self-setting iPSMSC-alginate-calcium phosphate paste for bone tissue engineering. Dent Mater 2016;32:252-63. [Crossref] [PubMed]
- Gonzalez-Fernandez T, Tierney EG, Cunniffe GM, et al. Gene Delivery of TGF-beta3 and BMP2 in an MSC-Laden Alginate Hydrogel for Articular Cartilage and Endochondral Bone Tissue Engineering. Tissue Eng Part A 2016;22:776-87. [Crossref] [PubMed]
- Lv K, Li Q, Zhang L, et al. Incorporation of small extracellular vesicles in sodium alginate hydrogel as a novel therapeutic strategy for myocardial infarction. Theranostics 2019;9:7403-16. [Crossref] [PubMed]
- Hao X, Silva EA, Mansson-Broberg A, et al. Angiogenic effects of sequential release of VEGF-A165 and PDGF-BB with alginate hydrogels after myocardial infarction. Cardiovasc Res 2007;75:178-85. [Crossref] [PubMed]