Accumulation of TNFR2-expressing regulatory T cells in malignant pleural effusion of lung cancer patients is associated with poor prognosis
Introduction
The central role of the immune system in killing human cancer cells is undebated (1). Compelling evidence has implicated that the adaptive immune system, mainly T cells immunity mediated by effector T cells (Teffs), plays crucial roles in tumor immunity (2,3). Teffs comprise different functional subtype and these cells can directly or indirectly kill tumors by synthesizing various biomolecules such as Interferon-γ (IFN-γ), tumor necrosis factor (TNF), perforin and/or granzymes (3,4). In contrast, regulatory T cells (Tregs), a subset of CD4+ T cells characterized by the expression of a key transcription factor forkhead box p3 (Foxp3) (5,6), are potent immunosuppressive cells that dampen anti-tumor immunity by suppressing Teffs activities and contribute to the development of the immunosuppressive tumor microenvironment, thus promoting immune evasion and cancer progression (3,7,8). Accumulation of Treg cells within tumor tissues and the resultant of high ratio of Treg to Teff, are associated with poor prognosis of cancer patients, including those with lung cancer (9,10), breast cancer (11), colorectal cancer (12), pancreatic cancer (13), and other malignancies (14). Hence, strategies to eliminating tumor-infiltrating Tregs to break immunosuppressive networks and re-establish anti-tumor immunity are urgently required in the cancer immunotherapy.
Malignant pleural effusions (MPEs) are a common, debilitating complication of the spread of metastatic cancer to serous cavities, with an estimated annual incidence in the United States of more than 200,000 cases (15,16). Patients suffering from MPE usually face a uniformly fatal prognosis and a life expectancy of only 3 to 12 months (16). Research on the immune landscape of MPE has shed light on the presence of potential antigen-presenting and effector cells, but host immunity has failed to contain the malignancy (15,17). This deadly outcome may be partly caused by the immunosuppressive activity in MPE (15).
Tregs may represent a major cellular mechanism in immune suppression by dampening the anti-tumor responses in MPE (15,17-22) . Previously, we observed an increased frequency of Tregs present in MPE compared to the corresponding blood or benign pleural effusions (9,19). They exhibit distinct phenotypic and functional profiles, upregulating markers associated with enhanced suppressive activity (9,20). These include immunosuppressive molecules, cytotoxic T-lymphocyte associated protein 4 (CTLA-4), glucocorticoid-induced TNFR family related gene (GITR), CD39; and inhibitory cytokines, including interleukin-10 (IL-10) and transforming growth factor-β (TGF-β) (19,23). In line with this, these functional Tregs present in MPE significantly inhibited the proliferation and cytokine production of co-cultured MPETeffs upon T cell receptor (TCR) stimulation (18,23). This process may occur in two ways, by cell-to-cell direct contact or by secretion of inhibitory cytokines according to the functional frofiles. Moreover, accumulation of Treg cells within MPE, and the high ratio of Tregs to Teffs in MPE was reported to be associated with an unfavorable prognosis (9,22). In this context, the question of which specific factors or signals present in MPE selectively modulate Treg frequency and activity is of considerable current interest. Moreover, identifying and targeting the most active Treg population within MPE is crucial to enhancing anti-tumor immunity.
Tumor-infiltrating Tregs persistently express higher levels of tumor necrosis factor type II receptor (TNFR2) than Teffs, and these TNFR2+Tregs are considered to be the maximally suppressive subset of Tregs (24-26). To date, the significance of TNFR2 expression on Tregs in MPE remains unclear. Furthermore, tumor necrosis factor (TNF), which is expressed by activated Teffs (27), plays a decisive role in the activation and expansion of Tregs via interaction with TNFR2 (28,29). We wonder whether Teffs in MPE abundantly express TNF, which could partly explain the “cold” function against tumor.
In this study, we have confirmed that TNFR2 can be considered as a reliable dentifying feature for functional Tregs in MPE, and has great clinical importance. TNF/TNFR2 signaling plays a decisive role in the suppressive function of MPE Tregs, and silencing of this pathway is an effective strategy for MPE immunotherapy. Furthermore, we have observed that T helper 1 (Th1) and T helper 17 (Th17) cells provide quite a large of TNF in MPE, which may expand the relationships between Tregs and Teffs in MPE. Taken together, our data expanded the immunosuppressive mechanism present in MPE induced by Tregs, and provides novel insight for the clinical diagnosis, disease evaluation, and treatment of MPE patients. We present the following article in accordance with the Materials Design Analysis Reporting (MDAR) reporting checklist (available at http://dx.doi.org/10.21037/atm-20-7181).
Methods
Ethical statement
The trial was conducted in accordance with the Declaration of Helsinki (as revised in 2013). The study protocol and clinical data of patients were approved by Ethics Committee of the Union Hospital, Tongji Medical College, Huazhong University of Science and Technology (2019-S809). Informed consent was obtained from all patients.
Patients
Malignant pleural fluid samples were collected from 41 patients with newly histologically-diagnosed lung adenocarcinoma with MPE. The MPE diagnosis was established by demonstration of malignant cells in pleural fluid, closed pleural biopsy, or both. Patients were excluded if they had undergone any invasive procedures directed into the pleural cavity or if they had suffered chest trauma within 3 months prior to hospitalization. At the time of sample collection, none of the patients had received any anti-cancer therapy, corticosteroids, or other non-steroidal anti-inflammatory drugs.
Tuberculous pleural effusion (TPE) samples were collected from 36 anti-HIV antibody (Ab) negative patients with tuberculous pleurisy, as evidenced by the presence of Mycobacterium tuberculosis (MTB) in pleural fluid or by demonstration of granulomatous pleurisy on pleural biopsy specimen in the absence of any evidence of other granulomatous diseases. After anti-tuberculosis chemotherapy, the resolution of TPE and clinical symptoms was observed in all of the patients. At the time of sample collection, none of the patients had received any anti-tuberculosis therapy, corticosteroids, or other non-steroidal anti-inflammatory drugs.
Clinical data collection
The clinical characteristics and laboratory findings of all 41 MPE patients were obtained from the electronic medical records of Wuhan Union Hospital (summarized in Table 1). The laboratory examinations included the following: routine laboratory tests, biochemical indicators, tumor markers of pleural effusion, and Epidermal Growth Factor Receptor (EGFR) mutations. The proportions of tumor cells in MPE were obtained from pathology reports recorded by pathologists. The maximum pleural effusion anteroposterior diameter (PEAD) was determined by ultrasonography. The Eastern Cooperative Oncology Group (ECOG) performance status (PS) scores are provided in the Supplementary Appendix.
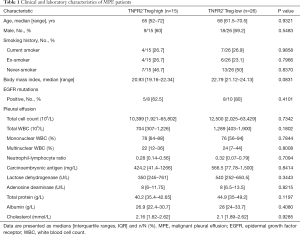
Full table
Sample collection and processing
The pleural fluid samples were collected in heparin-treated tubes from each patient using a standard thoracentesis technique within 24 h of hospitalization. Ten milliliters of peripheral blood (PB) was drawn simultaneously. MPE specimens were immediately put on ice and then centrifuged at 1,500 r for 6 min. The cell-free supernatants of MPE and serum were frozen at −80 °C immediately after centrifugation for later determining cytokine concentrations. The MPE cell pellets were resuspended in 1× phosphate buffered saline (PBS), and pleural effusion mononuclear cells (PEMCs) were isolated by Ficoll-Hypaque gradient centrifugation (StemCell Technologies, Vancouver, British Columbia, Canada).
Cell isolation and culture
Cluster of differentiation (CD)4+T cells were purified from PEMCs with a CD4+T cell isolation kit (Miltenyi Biotec, Bergisch Gladbach, Germany) according to the manufacturer’s instructions. The purity of the CD4+T cells was >97%, as measured by flow cytometry. Purified CD4+T cells (1×106 per well) or fresh PEMCs (1×106 per well) were cultured in a 48-well plate (Corning Costar, Corning, NY, USA) with 1 ml Roswell Park Memorial Institute (RPMI)-1640 medium (Solarbio, Beijing, China) containing 10% fetal bovine serum (FBS, Gibco, Invitrogen, Carlsbad, CA, USA) for 72 h with TNF (50 ng/mL) (PeproTech, Rocky Hill, NJ, USA), anti-TNFR2 (10 µg/mL), or rat immunoglobulin G (IgG) isotype (10 µg/mL) (Biolegend, San Diego, CA, USA) according to different requirements.
Flow cytometry and intracellular cytokine staining
The expression markers on T cells from MPE were identified by flow cytometry after surface or intracellular staining with anti-human-specific Abs conjugated with either phycoerythrin or fluorescein isothiocyanate (Table 2). These human Abs included anti-CD3, anti-CD4, anti-CD8, anti-CD25, anti-TNFR2, anti-programmed cell death-ligand 1 (PD-L1), anti-CTLA-4, anti-Ki-67, anti-forkhead box P3 (Foxp3), anti- interferon gamma (IFN-γ), anti-interleukin 17 (IL-17), and anti-TNF monoclonal antibodies (mAbs), which were purchased from BD Biosciences (San Diego, CA, USA), eBioscience (San Diego, CA, USA), or Biolegend (San Diego, CA, USA). Intracellular staining for IL-17, IFN-γ, and TNF was performed on T cells stimulated with phorbol myristate acetate (PMA) (50 ng/mL; Sigma-Aldrich, St. Louis, MO, USA) and ionomycin (1 µg/mL; Sigma-Aldrich) in the presence of Brefeldin A (1×; eBioscience) for 5 h. The intracellular IL-17, IFN-γ, or TNF was then stained with mAbs. Flow cytometry was performed on BD LSRFORTESSA X-20 (BD Biosciences) and the data were analyzed using FlowJo software version 10.0.7 (Tree Star Inc., Ashland, OR, USA). Fluorescence-activated cell sorting (FACS) analysis was performed on the live cells alone using Fixable Viability Stain purchased from BD Biosciences.
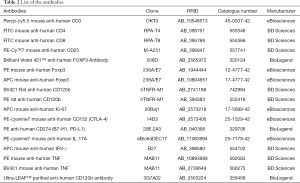
Full table
Measurement of cytokines
The concentrations of TNF in MPE and sera, as well as IFN-γ in culture supernatants, were measured using sandwich enzyme-linked immunosorbent assay (ELISA) kits (R&D Systems Inc, Minneapolis, MN, USA) according to the manufacturer’s protocols. All samples were assayed in duplicate.
Statistical analysis
The Student’s t-test, Wilcoxon sign-rank test, or Mann-Whitney test was used for comparison of two indicated groups. Chi-squared tests were applied to categorical variables. One-way analysis of variance (ANOVA) was used for the comparisons in multiple groups. Correlations between variables were determined by Spearman’s rank correlation coefficients. Survival was estimated by the Kaplan-Meier method and compared by the log-rank test. Significance was determined using P<0.05. All statistical analyses were performed using the SPSS 13.0 (SPSS Inc., Chicago, IL, USA).
Results
High TNFR2 expression is a characteristic of MPE Tregs in lung cancer patients
To characterize CD4+Foxp3+Tregs (Tregs) present in MPE, MPE was collected from 41 patients with stage IV lung cancer. As a comparison, Tregs in the PB were collected from the same patient and analyzed. As shown in Figure 1A,B, the proportion of Foxp3+ cells present in CD4+T cells present in MPE (18.14%) was approximately two times higher than that in the PB (10.17%, P<0.0001). Furthermore, Tregs present in the MPE appeared to express higher levels of Foxp3 on a per cell basis, as shown by the mean fluorescent intensity (MFI) of Foxp3 expression (P<0.0001, Figure 1C). As expected, the proportion of TNFR2+ cells in CD4+Foxp3+Tregs (Tregs) in MPE (72.66%±1.87%) was markedly higher than that expressed by CD4+Foxp3- effector T cells (Teffs) (34.45%±3.22%, P<0.0001, Figure 1D,E). Moreover, the MFI of TNFR2 expression by MPE Tregs was about two times higher compared to TNFR2 MFI expressed by MPE Teffs (P<0.0001, Figure 1F).
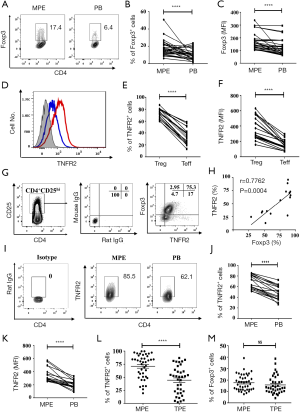
In CD4+T cells present in MPE, CD25hi could identify Tregs, as indicated by the high Foxp3 expression (18,21). We also found that TNFR2 and Foxp3 were co-expressed by the majority of CD4+CD25hi T cells in MPE (Figure 1G), and these two markers were positively correlated (Figure 1H). Furthermore, 85.5% of Foxp3-expressing MPE Tregs expressed TNFR2, which was notably higher than the TNFR2 expressed by PB Tregs (62.1%, P<0.0001, Figure 1I,J). Also, the intensity of TNFR2 expression on a per cell basis by MPE Tregs was approximately two times higher than PB Tregs (Figure 1K, P<0.0001). Thus, high expression of TNFR2 is a phenotypical characteristic of Tregs in patients with MPE, which is akin to Tregs present in the solid tumor environment or in the ascites of ovarian cancer patients (25,30).
Clinically, distinguishing MPE from TPE remains a challenge since they have similar clinical or laboratory manifestations, and sometimes lack pathological evidence. In this study, pleural effusion samples from a total of 41 patients with MPE and 36 patients with TPE were analyzed and compared. Surprisingly, the proportion of TNFR2+ cells present in MPE Tregs was considerably higher than that in TPE Tregs (79% vs. 51.05%, Figure 1L; P<0.0001). However, no differences were observed in the frequency of total Tregs in CD4+T cells (16.6% vs. 13.3%, Figure 1M; P>0.05). The data further supports the notion that TNFR2 is the characteristic marker of tumor-associated Tregs. Our findings also suggest a value of TNFR2 in the diagnosis of MPE.
Expression of TNFR2 on MPE Tregs has clinicopathological implications
Previous studies have reported that a high percentage of malignant cells in the pleural space are associated with the immunosuppressive milieu in MPE (31,32). Interestingly, the frequency of TNFR2-expressing Tregs was positively correlated with the frequency of tumor cells in MPE (r=0.458, P=0.019, n=26, Figure 2A), and the maximum PEAD was measured by ultrasound (r=0.56, P=0.001, n=30, Figure 2B). It is possible that TNFR2+ Tregs promote tumor metastasis, while metastatic tumors in MPE might induce the chemotaxis or proliferation of TNFR2+ Tregs. However, the exact mechanism needs to be further elucidated.
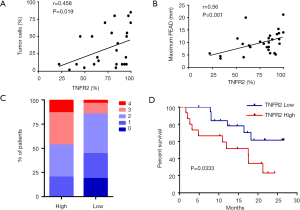
Next, we investigated the relationships between TNFR2 expression levels on Tregs and the clinical parameters in patients with MPE. Based on the percentages of TNFR2 in Tregs determined by flow cytometry, patients with a value equal to or more than 85% were described as “high TNFR2+Tregs (n=15)”, and those below as “low TNFR2+Tregs (n=26)”. The demographics, clinical characteristics, and laboratory parameters between these two groups are shown in Table 1. Poor ECOG PS is a negative prognostic factor for lung cancer patients (33). We noted that a poor ECGO PS (≥3) at admission was more frequent in patients with high TNFR2+Tregs (47% vs. 15%, P=0.029, Figure 2C). Moreover, patients with low TNFR2+Tregs had significantly longer overall survival (median, 8.0 months; Figure 2D) than patients with high TNFR2+Tregs (median, 4.8 months). Individuals in the high TNFR2+Tregs group experienced a 2.8-fold higher risk of death compared with those in the low TNFR2+Tregs group [95% confidence interval (CI), 1.1–8.3]. Therefore, our data supports the idea that an increase in the frequency of TNFR2+ Tregs in MPE is a predictor of increased risk of death and reduced survival in patients with MPE.
TNFR2-expressing Tregs in MPE exhibit a highly proliferative and functional phenotype
TNFR2 acts as a costimulatory molecule that considerably enhances the activation of lymphocytes by TCR signals (34,35). It has been shown previously that TNFR2 is expressed in highly proliferative Tregs (24). Hererin, we analyzed the functionally phenotypic characteristics of TNFR2+Tregs present in MPE (Figure 3). Indeed, the proportion of Ki-67+ cells in MPE TNFR2hi Tregs (69.3%) was significantly higher than that in TNFR2low Tregs (27.9%, P<0.0001) and TNFR2neg Tregs (10.9%, P<0.0001, Figure 3A,B,C). Cytotoxic T lymphocyte-associated antigen (CTLA)-4-mediated subversion of antigen-presenting cell function and PD-L1-mediated contact inhibition reportedly participates in the suppressive activity of Tregs in the tumor microenvironment (23,36-38). Interestingly, the proportions of CTLA-4+ cells (49.3%±6.73%) and PD-L1+ cells (40.93%±10.16%) present in TNFR2+ Tregs were notably higher compared with those in TNFR2- Tregs (27.82%±7.06%, 17.23%±6.144%, respectively; all P<0.001; Figure 3E,F). The expression of TNFR2 and Ki-67 (r=0.885, P<0.0001; Figure 3D), as well as CTLA-4 and PD-L1 (r=0.905 for CTLA-4; 0.952 for PD-L1; all P<0.0001; Figure 3G) by MPE Tregs were positively correlated, indicating that TNFR2-expressing Tregs in MPE are highly proliferative with potent suppressive activity.
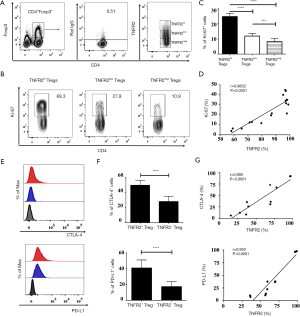
TNF-TNFR2 interaction enhances immunosuppressive function of Tregs in MPE
Previous studies have demonstrated that TNFR2 not only serves as a surface marker for the identification of Tregs but also promotes Treg function (29,35,39). Thus, we investigated the effects of TNF-TNFR2 interaction on Tregs in MPE. Purified CD4+T cells from MPE were treated with TNF for 72 hours. The result showed that the proportions of both CTLA-4+ cells and PD-L1+ cells present in TNFR2+ Tregs were significantly increased after TNF treatment (from 24.5% to 34.6% for CTLA-4; from 69.8% to 85.2% for PD-L1; all P<0.05; Figure 4). Notably, the TNF-induced up-regulation of CTLA-4 and PD-L1 expression on TNFR2+ Tregs were completely abrogated by anti-TNFR2 Abs treatment (Figure 4, all P<0.01), indicating that the TNF-TNFR2 interaction contributes to the immunosuppressive function of Tregs in MPE.
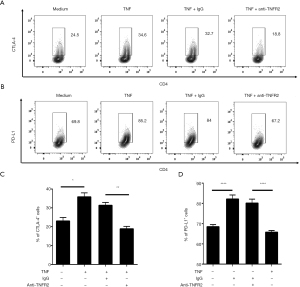
Th1 and Th17 in MPE are major TNF producers
Since TNFR2 mediates the effects of TNF on the functions of Tregs in MPE, and the concentrations of TNF in MPE were three times higher than the corresponding plasma (Figure 5A; P<0.0001), we determined the cell origins of TNF in MPE using flow cytometry (Figure 5B). The data showed that CD4+T cells comprised up to 67% of the total TNF+ cells in MPE, while CD8+T cells accounted for 18%, and CD3- cells (presumably myeloid cells) accounted for 15% (Figure 5C), which is likely attributable to the fact that CD4+T cells are a dominant population in MPE (Figure S1). In addition, we also noted that the percentage of TNF+ cells in CD4+ T cells (65%±5.7%) was considerably higher compared to CD8+T cells (56%±5.6%; P<0.05) and CD3- cells (47%±6.3%; P<0.001; Figure 5D).
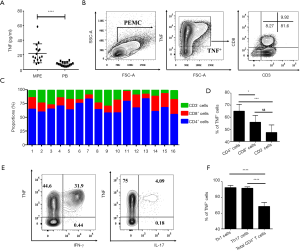
Previous studies have reported that the accumulation of IFN-γ-producing CD4+T (Th1) cells and IL-17-producing CD4+T (Th17) cells frequently occurs in MPE (21,23). Herein, we confirmed these reports and found that ~32% of MPE CD4+T cells were INF γ-producing cells and ~4.2% of MPE CD4+T cells were IL-17-producing cells (Figure 5E). Interestingly, ~95% of INF γ+ cells and IL-17+ cells co-expressed TNF (Figure 5E,F). Therefore, Th1 and Th17 cells in MPE appear to be the major source of TNF and may account for the activation and function of Tregs in MPE.
To our previous knowledge, Teffs play central roles in orchestrating adaptive immune responses to tumor antigens, which can be suppressed by Tregs in tumor microenvironment. In this context, the functions of Teffs and Tregs were mainly considered to be reciprocal inhibition and antagonism. However, our findings indicate that Teffs may support the suppressive activity of Tregs via TNF/TNFR2 pathway, which provide new insights in the relationships between Tregs and Teffs. Furthermore, these data also indicated that Teffs present in MPE may possess dual function as both immunity and immunosuppression. If blindly increasing the number or enhancing the function of Teffs in MPE, the immunosuppressive activity of Tregs may be also enhanced as a side effect. Therefore, further understanding of the complex interactions between these two functional distinct cells is crucial for devising effective and safe treatment of immunotherapy of cancer.
Blockade of TNFR2 increases IFN-γ-expressing CD8+CTLs in MPE
As we identified that blocking TNFR2 impaired the immunosuppressive function of Tregs in MPE (Figure 4), we further tested the consequent effects on the activation of CD8+cytotoxic T lymphocytes (CD8+CTL). To this end, PEMCs were isolated from MPE and treated with neutralizing anti-TNFR2 Abs or an isotype control. As shown in Figure 6, after 72 hours of treatment, the proportion of IFN-γ+ cells in CD8+ CTL was increased from 49.8% to 62.7% (Figure 6A,B; P=0.0006). Consistently, the concentrations of IFN-γ in the supernatant was elevated by approximately two times following TNFR2 blockade (Figure 6C, P=0.0077). Thus, blocking TNFR2 in PEMCs markedly enhanced the anti-tumor activity of CD8+CTLs. These data indicated that targeting TNFR2 may offer an exciting treatment strategy to enhance anti-tumor immunity within MPE and may help improve the prognosis for lung cancer patients in the end stage. Further clinical trials should be carried out to provide more compelling evidences in the future.
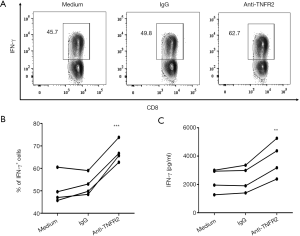
Discussion
Our data presented in this study showed that TNFR2-expressing Tregs aberrantly accumulated in MPE with highly replicating property and potent immunosuppressive function, which was contributed to maintaining the immunosuppressive activity of MPE. This idea was supported by the observation that blockade of TNFR2 potently enhanced the anti-tumor activity of CD8+CTLs in MPE. Moreover, retrospectively analysed clinical data of patients showed that higher frequencies of TNFR2+Tregs in MPE were associated with more tumor cells present in MPE and larger volume of MPE, indicating TNFR2+Tregs might promote tumor cells invasion and metastasis to pleural cavity and contribute to the development and progression of MPE. As expected, patients with higher frequencies of TNFR2+Tregs in MPE are more likely to have poor prognosis.
In this study, we observed that significantly higher levels of TNFR2 were expressed by MPE Tregs compared with MPE Teffs or by Tregs in PB. Our results are consistent with previous studies that show that Tregs present in ascites in patients with ovarian cancer expressed high levels of TNFR2 (25,30). Our data suggested that TNF-TNFR2 interaction likely plays an important role in the activation and expansion of Tregs in MPE. Thus, our data suggests that targeting of TNFR2 may present a novel strategy to selectively eliminate MPE Tregs, while having no or only minor effects on regular Tregs in the periphery, which are required for the maintenance of immunological homeostasis. Presumably, targeting of TNFR2+ Tregs might mitigate the possibility of developing systemic autoimmune inflammatory responses, which cause severe collateral damage to normal tissues evident after treatment with cancer immunotherapeutic regimens (40).
Our experimental results indicate that the frequencies of TNFR2-expressing Tregs were positively correlated with the number of tumor cells in MPE. It is well known that tumor cells evolve multiple sophisticated mechanisms to escape immune surveillance, including the creation of an immune tolerogenic microenvironment by recruiting immune suppressive cells (41). Thus, it is possible that TNFR2+ Tregs and the tumor form positive feedback loops to reciprocally promote each other, resulting in an increasingly immunosuppressive environment, as well as rapid tumor growth and persistent progression of MPE. In line with this, we observed that the high level of TNFR2 expression on Tregs positively correlated with poor prognosis. Further investigation of the mutual interaction between TNFR2+ Tregs and tumor cells should be carried out in the future.
TNF is a pleiotropic cytokine involved in diverse physiological and pathological processes, including tumor progression and metastasis (42). In fact, the concept that TNF is an important effector of MPE formation and progression was proposed a decade ago (43). This is consistent with our findings that TNF-TNFR2 interactions contribute to the activation and immunosuppressive function of Tregs in MPE, which play decisive roles in maintaining an immunosuppressive environment. Previously, it was well documented that TNFR2+Tregs were considered to be the maximally suppressive subset of Tregs (26,44). In the ascites of ovarian cancer patients, TNFR2+Tregs expressed high levels of the immunosuppressive molecules CD73 and CD39, and produced more immunosuppressive cytokines, including IL-10 and transforming growth factor-beta (TGF-β) (45). In this study, we observed that TNFR2+Tregs present in MPE of lung cancer patients expressed higher levels of the immunosuppressive molecules PD-L1 and CTLA-4, and replicating marker Ki-67, which is akin to TNFR2+Tregs from acute myeloid leukemia (AML) patients (25). Thus, targeting the TNF-TNFR2 pathway may provide an effective strategy to enhance anti-tumor immunity, as it could potentially disrupt a range of regulatory circuits that ensure dampening of the immune response. This may explain why the proportions of IFN-γ-producing CTLs were significantly increased following TNFR2 blockade. Interestingly, we found that the Th1 and Th17 cells are the major source of TNF in MPE. Thus, MPE Teffs may paradoxically promote tumor growth by boosting MPE Treg activity via the TNF/TNFR2 pathway. Further understanding the complex interplay between Teffs and Tregs in MPE may help to formulate safer and more effective immunotherapy for MPE.
Taken together, our data demonstrates that TNFR2 is preferentially expressed by MPE Tregs with highly replicating and highly suppressive properties, and identifies that targeting of TNFR2 can considerably boost the anti-tumor immune responses in MPE. Moreover, patients with high frequencies of TNFR2+Tregs are associated with a poor prognosis, and the discrepancies between TNFR2+Tregs frequencies are prevalent between malignant and tuberculosis pleural effusions, suggesting the potential value of TNFR2+Tregs in diagnosis and prognosis. Therefore, this study provides novel insights for the clinical diagnosis, prognosis evaluation, and immunotherapy of lung cancer patients with MPE.
Acknowledgments
Funding: This work was supported by grants from the National Natural Science Foundation of China (No. 81973990; No. 81900096; No. 81770090).
Footnote
Reporting Checklist: The authors have completed the MDAR reporting checklist. Available at http://dx.doi.org/10.21037/atm-20-7181
Data Sharing Statement: Available at http://dx.doi.org/10.21037/atm-20-7181
Conflicts of Interest: All authors have completed the ICMJE uniform disclosure form (available at http://dx.doi.org/10.21037/atm-20-7181). The authors have no conflicts of interest to declare.
Ethical Statement: The authors are accountable for all aspects of the work in ensuring that questions related to the accuracy or integrity of any part of the work are appropriately investigated and resolved. The trial was conducted in accordance with the Declaration of Helsinki (as revised in 2013). The study protocol and clinical data of patients were approved by Ethics Committee of the Union Hospital, Tongji Medical College, Huazhong University of Science and Technology (2019-S809). Informed consent was obtained from all patients.
Open Access Statement: This is an Open Access article distributed in accordance with the Creative Commons Attribution-NonCommercial-NoDerivs 4.0 International License (CC BY-NC-ND 4.0), which permits the non-commercial replication and distribution of the article with the strict proviso that no changes or edits are made and the original work is properly cited (including links to both the formal publication through the relevant DOI and the license). See: https://creativecommons.org/licenses/by-nc-nd/4.0/.
References
- Schreiber RD, Old LJ, Smyth MJ. Cancer immunoediting: integrating immunity's roles in cancer suppression and promotion. Science 2011;331:1565-70. [Crossref] [PubMed]
- Schirrmacher V, Feuerer M, Fournier P, et al. T-cell priming in bone marrow: the potential for long-lasting protective anti-tumor immunity. Trends Mol Med 2003;9:526-34. [Crossref] [PubMed]
- Yin Z, Bai L, Li W, et al. Targeting T cell metabolism in the tumor microenvironment: an anti-cancer therapeutic strategy. J Exp Clin Cancer Res 2019;38:403. [Crossref] [PubMed]
- Horn T, Grab J, Schusdziarra J, et al. Antitumor T cell responses in bladder cancer are directed against a limited set of antigens and are modulated by regulatory T cells and routine treatment approaches. Int J Cancer 2013;133:2145-56. [Crossref] [PubMed]
- Campbell DJ, Ziegler SF. FOXP3 modifies the phenotypic and functional properties of regulatory T cells. Nat Rev Immunol 2007;7:305-10. [Crossref] [PubMed]
- Hori S, Nomura T, Sakaguchi S. Control of regulatory T cell development by the transcription factor Foxp3. Science 2003;299:1057-61. [Crossref] [PubMed]
- Najafi M, Farhood B, Mortezaee K. Contribution of regulatory T cells to cancer: A review. J Cell Physiol 2019;234:7983-93. [Crossref] [PubMed]
- Chaudhary B, Elkord E., Regulatory T. Cells in the Tumor Microenvironment and Cancer Progression: Role and Therapeutic Targeting. Vaccines (Basel) 2016;4:28. [Crossref] [PubMed]
- Budna J, Spychalski Ł, Kaczmarek M, et al. Regulatory T cells in malignant pleural effusions subsequent to lung carcinoma and their impact on the course of the disease. Immunobiology 2017;222:499-505. [Crossref] [PubMed]
- Tao H, Mimura Y, Aoe K, et al. Prognostic potential of FOXP3 expression in non-small cell lung cancer cells combined with tumor-infiltrating regulatory T cells. Lung Cancer 2012;75:95-101. [Crossref] [PubMed]
- Shou J, Zhang Z, Lai Y, et al. Worse outcome in breast cancer with higher tumor-infiltrating FOXP3+ Tregs: a systematic review and meta-analysis. BMC Cancer 2016;16:687. [Crossref] [PubMed]
- Lin YC, Mahalingam J, Chiang JM, et al. Activated but not resting regulatory T cells accumulated in tumor microenvironment and correlated with tumor progression in patients with colorectal cancer. Int J Cancer 2013;132:1341-50. [Crossref] [PubMed]
- Liu L, Zhao G, Wu W, et al. Low intratumoral regulatory T cells and high peritumoral CD8(+) T cells relate to long-term survival in patients with pancreatic ductal adenocarcinoma after pancreatectomy . Cancer Immunol Immunother 2016;65:73-82. [Crossref] [PubMed]
- Mougiakakos D, Johansson CC, Trocme E, et al. Intratumoral forkhead box P3-positive regulatory T cells predict poor survival in cyclooxygenase-2-positive uveal melanoma. Cancer 2010;116:2224-33. [Crossref] [PubMed]
- Murthy P, Ekeke CN, Russell KL, et al. Making cold malignant pleural effusions hot: driving novel immunotherapies. Oncoimmunology 2019;8:e1554969. [Crossref] [PubMed]
- Donnenberg AD, Luketich JD, Dhupar R, et al. Treatment of malignant pleural effusions: the case for localized immunotherapy. J Immunother Cancer 2019;7:110. [Crossref] [PubMed]
- Steinfort DP, Kranz S, Dowers A, et al. Sensitive molecular testing methods can demonstrate NSCLC driver mutations in malignant pleural effusion despite non-malignant cytology. Transl Lung Cancer Res 2019;8:513-8. [Crossref] [PubMed]
- Ye ZJ, Zhou Q, Zhang JC, et al. CD39+ regulatory T cells suppress generation and differentiation of Th17 cells in human malignant pleural effusion via a LAP-dependent mechanism. Respir Res 2011;12:77. [Crossref] [PubMed]
- Budna J, Kaczmarek M, Kolecka-Bednarczyk A, et al. Enhanced Suppressive Activity of Regulatory T Cells in the Microenvironment of Malignant Pleural Effusions. J Immunol Res 2018;2018:9876014. [Crossref] [PubMed]
- Lv M, Xu Y, Tang R, et al. miR141-CXCL1-CXCR2 signaling-induced Treg recruitment regulates metastases and survival of non-small cell lung cancer. Mol Cancer Ther 2014;13:3152-62. [Crossref] [PubMed]
- Yang WB, Ye ZJ, Xiang F, et al. Th17/Treg imbalance in malignant pleural effusion. J Huazhong Univ Sci Technolog Med Sci 2013;33:27-32. [Crossref] [PubMed]
- Yang G, Li H, Yao Y, et al. Treg/Th17 imbalance in malignant pleural effusion partially predicts poor prognosis. Oncol Rep 2015;33:478-84. [Crossref] [PubMed]
- Chen YQ, Shi HZ, Qin XJ, et al. CD4+CD25+ regulatory T lymphocytes in malignant pleural effusion. Am J Respir Crit Care Med 2005;172:1434-9. [Crossref] [PubMed]
- Chen X, Hamano R, Subleski JJ, et al. Expression of costimulatory TNFR2 induces resistance of CD4+FoxP3- conventional T cells to suppression by CD4+FoxP3+ regulatory T cells. J Immunol 2010;185:174-82. [Crossref] [PubMed]
- Govindaraj C, Scalzo-Inguanti K, Madondo M, et al. Impaired Th1 immunity in ovarian cancer patients is mediated by TNFR2+ Tregs within the tumor microenvironment. Clin Immunol 2013;149:97-110. [Crossref] [PubMed]
- Chen X, Subleski JJ, Kopf H, et al. Cutting edge: expression of TNFR2 defines a maximally suppressive subset of mouse CD4+CD25+FoxP3+ T regulatory cells: applicability to tumor-infiltrating T regulatory cells. J Immunol 2008;180:6467-71. [Crossref] [PubMed]
- Zhou Q, Hu Y, Howard OM, et al. In vitro generated Th17 cells support the expansion and phenotypic stability of CD4(+)Foxp3(+) regulatory T cells in vivo. Cytokine 2014;65:56-64. [Crossref] [PubMed]
- Biton J, Semerano L, Delavallee L, et al. Interplay between TNF and regulatory T cells in a TNF-driven murine model of arthritis. J Immunol 2011;186:3899-910. [Crossref] [PubMed]
- Chen X, Baumel M, Mannel DN, et al. Interaction of TNF with TNF receptor type 2 promotes expansion and function of mouse CD4+CD25+ T regulatory cells. J Immunol 2007;179:154-61. [Crossref] [PubMed]
- Torrey H, Butterworth J, Mera T, et al. Targeting TNFR2 with antagonistic anti-bodies inhibits proliferation of ovarian cancer cells and tumor-associated Tregs. Sci Signal 2017;10:eaaf8608. [Crossref] [PubMed]
- Stathopoulos GT, Kollintza A, Moschos C, et al. Tumor necrosis factor-alpha promotes malignant pleural effusion. Cancer Res 2007;67:9825-34. [Crossref] [PubMed]
- Roberts ME, Neville E, Berrisford RG, et al. Management of a malignant pleural effusion: British Thoracic Society Pleural Disease Guideline 2010. Thorax 2010;65 Suppl 2:i32-40. [Crossref] [PubMed]
- Lilenbaum RC, Cashy J, Hensing TA, et al. Prevalence of poor performance status in lung cancer patients: implications for research. J Thorac Oncol 2008;3:125-9. [Crossref] [PubMed]
- Kim EY, Priatel JJ, Teh SJ, et al. TNF receptor type 2 (p75) functions as a costimulator for antigen-driven T cell responses in vivo. J Immunol 2006;176:1026-35. [Crossref] [PubMed]
- Kim EY, Teh HS. TNF type 2 receptor (p75) lowers the threshold of T cell activation. J Immunol 2001;167:6812-20. [Crossref] [PubMed]
- Keir ME, Butte MJ, Freeman GJ, et al. PD-1 and its ligands in tolerance and immunity. Annu Rev Immunol 2008;26:677-704. [Crossref] [PubMed]
- Auchincloss H, Turka LA. CTLA-4: not all costimulation is stimulatory. J Immunol 2011;187:3457-8. [Crossref] [PubMed]
- Ohue Y, Nishikawa H., Regulatory T. Treg) cells in cancer: Can Treg cells be a new therapeutic target?. Cancer Sci 2019;110:2080-9. [Crossref] [PubMed]
- Hamano R, Huang J, Yoshimura T, et al. TNF optimally activatives regulatory T cells by inducing TNF receptor superfamily members TNFR2, 4-1BB and OX40. Eur J Immunol 2011;41:2010-20. [Crossref] [PubMed]
- Chen X, Oppenheim J J. Targeting TNFR2, an immune checkpoint stimulator and oncoprotein, is a promising treatment for cancer. Sci Signal 2017;10:eaal2328. [Crossref] [PubMed]
- Deng G. Tumor-infiltrating regulatory T cells: origins and features. Am J Clin Exp Immunol 2018;7:81-7. [PubMed]
- He J, Li R, Chen Y, et al. TNFR2-expressing CD4(+)Foxp3(+) regulatory T cells in cancer immunology and immunotherapy. Prog Mol Biol Transl Sci 2019;164:101-17. [Crossref] [PubMed]
- Stathopoulos GT, Kollintza A, Moschos C, et al. Tumor necrosis factor-alpha promotes malignant pleural effusion. Cancer Res 2007;67:9825-34. [Crossref] [PubMed]
- Chen X, Subleski JJ, Hamano R, et al. Co-expression of TNFR2 and CD25 identifies more of the functional CD4+FOXP3+ regulatory T cells in human peripheral blood. Eur J Immunol 2010;40:1099-106. [Crossref] [PubMed]
- Govindaraj C, Tan P, Walker P, et al. Reducing TNF receptor 2+ regulatory T cells via the combined action of azacitidine and the HDAC inhibitor, panobinostat for clinical benefit in acute myeloid leukemia patients. Clin Cancer Res 2014;20:724-35. [Crossref] [PubMed]
(English Language Editor: A. Kassem)