A narrative review of central nervous system involvement in acute leukemias
Background on the central nervous systems (CNS) involvement in acute leukemias
Therapy for acute leukemias, both myeloid and lymphoblastic, has become increasingly effective in the past decade (1-7). This is explained by the increasing efforts of personalizing chemotherapy treatment and supportive care that continuously adapts to initial diagnostic and progression of the disease, and which has resulted in better quality of life and higher overall survival (OS) rate in leukemic patients (8-11). Despite, improvements made to the diagnosis and treatment of both forms of acute leukemia: lymphoblastic (ALL) and myeloid (AML), the CNS involvement is still limiting long-term treatment, remaining one of the most severe complication and the primary causes of mortality (12,13). CNS involvement is detected either at the initial diagnosis or it develops at relapse. This event happens in 30% of relapse cases and it represents the main hurdle that leads to treatment failure (14).
In acute lymphoblastic leukemia (ALL), CNS involvement is more common and about 5% of adults are presenting with CNS leukemia at initial diagnosis, having a shorter OS in comparison with patients, who did not develop CNS leukemia (15,16). There is still no consensus regarding the rate of development of CNS disease in ALL even through this is a major therapeutic obstacle. It is generally accepted that a third of the relapse cases involve CNS in pediatric ALL (17,18). The established way of assessing CNS leukemia is lumbar puncture (LP). However, practitioners have to pay attention to the fact that in 10–30% of LPs there is a danger of blood entering into CSF due to the trauma caused by the needle, this is defined as traumatic LP (19). Some studies concluded that traumatic LP increases the chances of disease recurrence in ALL (20), especially in high-risk patients (21). The choice of during an LP only if symptoms are present in ALL is also a strategy to decrease the risk of recurrence. If LP should be introduced as routine check-up in adult ALL, then the procedures from childhood ALL or AML should be introduced, such as intrathecal cytarabine given from the beginning (22).
It is generally considered that AML cells rarely reach the CNS and develop tumors, (23,24), whereas in pediatric patients (25) this is a common event. This pattern might not accurately reflect the reality, as many times CNS involvement in adults remains undiagnosed (12,23). The main reasons behind undiagnosed CNS leukemia in adult AML is related to the lack of routine diagnostic LP; this procedure being performed only when CNS signs or symptoms are manifest (26,27). This is why in the case of hyperleukocytosis at diagnosis, even in the absence of other symptoms, the performance of a LP evaluation is advised (17,18). Still, attention must be paid to the risks of traumatic LP. CNS leukemia is a frequent complication of acute leukemia having some specific cytogenetic anomalies. In AML, chromosome 16 inversion (resulting core binding factor beta-myosin 11 heavy chain fusion protein) and chromosome 11 abnormalities [trisomy with gene amplification, including myeloid lymphoid leukemia (MLL)] represent risk factors for CNS leukemia (28). Still there are some reports the inv(16)/t(16;16) is a favorable prognostic marker in AML (29). In preB-ALL, t(1;19) translocation is a risk factor for CNS relapse and these cells are found to express MER kinase (30). MER positive ALL-blasts enter G0/G1 phase when co-cultured with CNS derived cells (31). In ALL with t(1;19) translocation is found the highly encountered E2A-PBX1-rearrangement (30), with increased IL7R expression on the blasts surface and CNS leukemia incidence (32).
CNS involvement during AML treatment is uncommon, and the incidence of CNS leukemia has decreased since the incorporation of high dose cytarabine, that is, as mentioned above, used as a preventive measure in AML. This drug has beneficial effects due to its ability to penetrate the blood-brain barrier (33), however, this is also the main reason for severe CNS toxicity induced by this drug (34,35). Prior to the use of high dose cytarabine, meningeal disease was seen in up to 20% of children and 16% of adults with AML (33). In a review comprising information from 3261 adult patients enrolled in German clinical trials, CNS involvement was documented in 0.6% of adult patients at the time of initial presentation and in 2.9% relapse cases (23). However, in infants, potential CNS involvement was identified in 29% of cases (12).
In order to better understand the development of CNS involvement in acute leukemia (both acute myeloid leukemia (AML) and acute lymphoblastic leukemia (ALL) it is important to analyze the problem from two perspectives: the biology of the disease and the clinical characteristics of CNS involvement.
We present the following article in accordance with the Narrative Review reporting checklist (available at http://dx.doi.org/10.21037/atm-20-3140).
Method used for literature search
In the current narrative review, we looked at the literature data on the mechanisms behind CNS invasion by ALL and AML cells and the clinical significance of CNS leukemia. All of the included studies were written in English. The vast majority of researched literature was focused on AML and ALL.
Cell biology of CNS penetration by the leukemic blast cell
CNS involvement in acute leukemias can either be occult (23) or clinically manifested as leukemic meningitis (36), or myeloid sarcoma (37); the first one being most frequently encountered.
It can be present at the initial diagnosis, but also can develop at any time during the natural course of disease, even after years of complete remission, as isolated CNS relapse (38). There is great variability in the incidence of CNS involvement between various forms of acute leukemia, but in recent years the trend has continuously increase thus stimulating a more in-depth analysis over this kind of extramedullary disease (EMD).
The role of leukemia stem cells (LSCs) in the development of CNS leukemia
Acute leukemia is maintained by a pool of self-renewing cells denominated leukemic stem cells (LSCs), that express CD34+CD38− and increased aldehyde dehydrogenase activity (39) with properties similar to other cancer stem cells (CSC) (40-44). The LSCs can originate from hematopoietic stem cells (HSCs) or from committed cells that have an inner higher self-renewal potential (45). In ALL, there is a strong possibility that these stem cells originate from fully differentiated cells that during malignant transformation re-gain stem-like properties. The LSCs in pediatric ALL were discovered committed cells (46). In AML LSCs are responsible for the maintenance of minimal residual disease which is responsible for treatment failure and disease relapse in AML. The reason behind the observed results is that the LSCs remain in G0 state and have high self-renewal capacity. Two pro-malignancy events have to happen in order for a malignant LSC to develop. The LCSs then “mature” into leukemic progenitors, then leukemic blasts, which are the most often therapeutic targets. However, as long as the LSCs pool is maintained, the therapeutic death of leukemic blasts is insufficient (47).
The pathway of CNS entering of leukemic cells
The leukemic cells, that proliferate (48) and infiltrate various tissues in the body (49), have a lot of properties in common with normal hematopoietic progenitors, especially a population of cells, called LSCs (49,50) or mature cells (e.g., activated monocytes), that are further explored. In order to access CNS, leukemic cells can move along the walls of the vascular channels connecting the bone marrow of the skull bones and vertebrae with the pachymeninges, cross the vascular endothelium by transendothelial migration or endothelium destruction, diffusely infiltrate the arachnoid, migrate and intensively proliferate in CSF and consequently infiltrate perivascular spaces and brain parenchyma (Virchow-Robin space) (51). This is caused by increased hypoxia and angiogenesis in the bone marrow microenvironment. The newly formed blood vessels are incapable to supply enough oxygen and thus cause general hypoxia, which creates a feed-back flop to sustain continuous new blood formation and increased vascular permeability (52).
The role of adhesion molecules in CNS leukemia development
Adhesion molecules in AML
Leukemic cells express high levels of adhesion molecules, that are also expressed by normal myeloid or lymphoid lineage cells. Thus, these cells are considered “sticky cells” and have an increased ability to adhere to endothelial surfaces, a fact that can explain leukostasis in narrow blood vessels (42-45). In AML, cells can express all classes of adhesion molecules, more often identified being VLA-4 (53,54), VLA-5 (55), LFA-1 (56,57), MAC-1 (58), L-selectin (59,60), VCAM-1 (57), ICAM-1 (56), PECAM-1 (61), CD34 (62,63), CD44 (64,65) and CD56 (66).
New insides regarding blast cells extramedullary dissemination are given by the study of myeloblast endothelium interactions (67-70). Unlike normal myeloblasts or neutrophils, the AML leukemic blast cells can migrate through the endothelium when co-cultured with endothelial cells in a Transwell assay (71).
Numerous studies have demonstrated that blast cells secrete cytokines like transmembrane TNF-alpha (72) or IL-1beta (73) and induce an increase in E-selectin (74), P-selectin (75,76), ICAM-1 and VCAM-1 expression on endothelial cells (71), in a time dependent manner. Otherwise, they can also activate the migration of endothelial cells. In AML, an increased heterogeneity of adhesion molecules expression between FAB subtypes exist, that varies from patient to patient. Studies report a higher incidence of MAC-1 (CD11b/CD18) integrin expression in M4 and M5 subtypes (58), where CNS disease or other kinds of EMD are more frequent (77-80). MAC-1 has a broad binding specificity, mediating the interaction of macrophages with the extracellular matrix (81), granulocytes and collagen from extracellular matrix (82) and macrophage with other cells through ICAM-1, during activation (83). MAC1 plays major roles in transendothelial migration and tissue infiltration in the case of neutrophils (84).
Despite migration, adhesion molecules have a lot of other functions. LSCs enter a quiescent state in vascular niches in the bone marrow, but probably other niches too, and they do so by the expression of adhesion molecules like VLA-4 and CD44 (85). The integrin alpha4-beta1 (VLA-4) induces NF-kB signaling after binding to VCAM-1 molecules on the surface of stromal cells and increases the chemoresistance, while CD44 mediates the attachment of stromal cells to hyaluronic acid (86). VLA4 can also interact with other molecules as are osteopontin and fibronectin (87,88).
Adhesion molecules may also be involved in cell growth, proliferation, survival and migration (31,89,90). The most common AML adhesion molecules with pro-malignant effects are: ligand binding to integrins triggers SRC kinase (91), FAK (focal adhesion kinase) (92) and ILK (integrin-linked kinase) activation (93).
The role of adhesion molecules in ALL
Leukemic cells in ALL also express adhesion molecules, including beta-1 integrin (94), beta-2 integrin (CD18) (95) LFA-1 (96), LFA-3/CD58 (97,98), ICAM-1 (97), CD44 (99) and L-selectin (100). New research showed that ALL blasts express alpha 6-integrin and move along laminin in the microvessels connecting skull and vertebral bone marrow with the meninges (101). VE-cadherin and PECAM-1 co-expression in T-ALL cells enhances transendothelial migration (102).
Stefanidakis et al. showed that a supramolecular structure called invadosome is assembled at the contact points between leukemic blasts and endothelial or stromal cells. The invadosome contains proMMP-9 (gelatinase B) attached to alphaMbeta2 integrin and serves as an enzymatic machinery for tissue invasion that degrades extracellular matrix components (103).
B-cell ALL CNS leukemia is primarily a meningeal disease (104,105). The cerebrospinal fluid (CSF) of healthy humans contains mainly T cells (90%), as well as B cells (5%) and monocytes (5%) (106). ALL relapse can occur in bone marrow or extramedullary sites (less frequent), with higher incidence in ALL-blast sanctuaries and approximately 7.5–15% of relapses occur in the CNS (107). As follows, the clonal origin of CNS leukemia in ALL is called ‘neurotrophic subclone’ (108-110). The bone marrow of patients with CNS relapse may have contained at diagnosis small subpopulations of leukemic blasts with a CNS-prone profile, that translates into SCD (stearoyl-CoA desaturase) positiveness (111), or increased expression of SPP1 (secreted phosphoprotein 1), the gene encoding for osteopontin (112). ALL leukemic stem cells engraft in vascular niches (not in perivascular cells) expressing CXCL12 (113) and E-selectin (114).
The leukemic cells acquire irregular shapes in brain parenchyma. In CNS-derived leukemic cells, the genes related to cell cycle and oxidative phosphorylation are downregulated. They compared the transcriptome of CNS, BM and CSF-derived B-ALL leukemic cells and found that gene sets uniquely upregulated in CNS-derived leukemic cells included those associated with hypoxia and glycolysis (115). CNS-derived ALL leukemic cells are adapted to hypoxia, a mechanism that was rewarded with the 2019 Nobel Prize in Medicine or Physiology (116,117).
The acquired capacity of malignant transformed leukemic cells is related to the secretion of cytokines and overexpression of their receptors.
CXCL12/CXCR4 axis is central in physiological hematopoietic cells development (118), but it also seems to play a role in leukemic cells neurotropism. In vitro studies revealed that astrocytes (119), choroid plexus epithelial cells (120) and meningeal cells (121) secrete CXCL12 and B-ALL blasts move toward and adhere to them (120), CXCL12 appears to promote B-ALL blasts migration across brain-CSF barrier (120,122), but possibly also across BBB (120). CCR7/CCL19 is considered essential for CNS infiltration in Notch1-induced T-ALL, but not seem to be true for other ALL types (123). In preB-ALL, pediatric patients with IL7R overexpression have a high CNS leukemia incidence during disease evolution, especially in relapse (32). CXCR3 and PSGL-1 enhance BBB penetration as proven by in vitro studies. In ALL, the expression of these surface proteins is stimulated by IL-15 (124).
The role of hypoxia and VEGF secretion in the development of CNS leukemia
VEGF synthesis was identified in leukemic cells of both AML and ALL (125). In AML, high levels of VEGF-A are associated with disease diagnostic (126), and shorter OS (127). In patients with ALL, no correlation has been identified between plasma VEGF levels and CNS leukemia, but in CSF the VEGF level was significantly increased in patients with CNS involvement (128-131). VEGF expression is higher in leukemia cells already infiltrated in CNS, in comparison with cells from BM (132). Studies on CNS-derived leukemic cells found that VEGFA is one of the most upregulated hypoxia-induced genes. Despite promoting angiogenesis, VEGF can also increase endothelial permeability, including BBB disruption, by acting directly on endothelial cells (101). Indirectly, VEGF acts on its own receptors on ALL cells and stimulate MMP-2 and MMP-9 secretion (133). VEGF levels in ALL are increased in patients with relapse compared with newly diagnosed cases (134).
In vitro studies revealed that ALL-derived exosomes increase BBB permeability and VEGF secretion in astrocytes (135). When analyzing CNS-derived leukemic cells, reports show that this cell population has distinctive transcriptional signature. These cells, apart from bone marrow (BM)- and CSF-derived cells, downregulate genes involved in cell cycle and oxidative phosphorylation and increase the expression of genes related to hypoxia and glycolysis [VEGFA, hexokinase 2 (HK2), pyruvate dehydrogenase 1 (PDH1)] (115).
Brain parenchyma appears to be seeded by LSCs that adapt to hypoxic microenvironment of perivascular spaces (136). They modify the neural stem cell niches and maintain their stemness and quiescence there, also protected from chemotherapy (137). Brain microenvironment has major influence on leukemic cells transcriptome profiling.
Gaynes et al. found that CNS-derived leukemia cells have high PBX1 expression. Further in vitro assays showed that PBX1 is upregulated in a co-culture models of preB-ALL cells with BBB epithelial cells and choroid plexus epithelial cells. Moreover, these leukemic cells having increased cytarabine and methotrexate chemoresistance. Despite chemoresistance, inducing PBX1 overexpression leads to increased CNS infiltration in vivo (138). CNS is one of the ALL-blast sanctuaries and once LSCs get there, they are protected from chemotherapy (107) and the natural killer (NK) cells from the patient’s immune system thus LSCs can persist as MRD in CNS or can rapidly develop into CNS leukemia (139). Meninges also appear to have protective effects on leukemic cells. In vitro studies show that adherence of ALL blasts to primary meningeal cells leads to increased chemoresistance, decreased apoptosis and quiescence (31).
The essential role of CD56 in development of CNS leukemia
Cluster of differentiation 56 (CD56), also called neural cell adhesion molecule (NCAM) is physiologically expressed by NK cells and a subset of mature T cells in the immune system (140-143). NCAM is the first adhesion molecule identified to have essential roles in both in utero and adult neurogenesis, synaptic plasticity and learning (144-147). When cells undergo malignant transformation, they express at a higher level CD56 and show an aggressive behavior (148). Apart from carcinomas with neuroendocrine phenotype (149) or tumors of neuroectodermal origin (150), well known for CD56 positivity, hematological malignancies can also express CD56 in various proportions. NCAM is expressed by malignant cells of NK leukemias (151), T-cell leukemias/lymphomas (152) and AML (153,154).
Through alternative splicing, the NCAM1 gene codes the synthesis of three isoforms noted as NCAM-120, NCAM-140 and NCAM-180, according to their molecular weight. All NCAM isoforms contain an N-terminal extracellular domain with five immunoglobulin‐like (Ig) modules and two fibronectin type III (F3) modules (155). Except for NCAM-120, which is GAP-anchored on the cell surface, the extracellular domains of NCAM-140 and NCAM-180 are followed by a short transmembrane segment and a C-terminal cytosolic domain, which is longer in the NCAM-180 isoform. In the immune cells, NCAM-140 is the dominant isoform, but in the neural tissue, they are differentially distributed: NCAM-120 isoform only in glia, NCAM-180 only in neurons and NCAM-140 isoform is expressed in both neurons and glia. It is the first adhesion molecule identified, but information about NCAM signaling still remains scarce, especially in immune cells. NCAM proteins form cis dimers on the cell surface but can also form tetramers after trans-interaction with other NCAM dimers on adjacent cells (156-158).
A link between NCAM and FGFR signaling was reported and it appears that the tetrameric form of NCAM triggers the activation of FGFR by lateral associations through the membrane. If NCAM ligands are present, spectrin mediates the colocalization of NCAM proteins with other molecules in lipid raft areas, leading to Fyn kinase activation, that in turn activates FAK (159-161).
Some of the pathways identified as being activated downstream from NCAM are Ras/MAPK (162), PLC/DAG/AA (155,163) and PI3K/Akt pathways (164), as inhibition of these key enzymes impairs the neurite outgrowth. Studies also noted the activation of PKC and PKA with consequent increase in intracellular calcium (165) and cAMP concentrations (166), following NCAM activation. The neurite outgrowth appears to depend on CREB (167,168), c-Fos (168) and NF-kB activation (169). These transcription factors are final targets in NCAM signaling.
CD56 is expressed on various cells from the immune system, not only by NK cells, but also by some populations of γδ T cells, CD8+ T cells, monocytes and monocyte-derived IL-15 dendritic cells (146). Little is known about NCAM function in the immune system, but it appears that, in NK cells, NCAM expression confers a high mobility potential (170), mediates NK cells adhesion to CD56 positive tumor cells thus amplifying the cytotoxic effects of NK cells (146) and it can also function as a pathogen recognition receptor (PRR) (171). In that context, NCAM expression on T cells positively correlates with the expression of CD16, NKG2A/D, NKp44/46, CD122, and DNAM-1 and with high intracytoplasmic perforin and granzyme B content (146). The intestinal T cell population has a high expression of CD56 and it has a stimulatory function on proliferation in CD56− T cells from peripheral blood (172).
CD56-bright NK cells only, and not the CD56dim subset, display CD117 (c-Kit) expression, high affinity receptor for IL-2 and CCR7 on their surface and express a higher level of CXCR3 (173).
The CD56 bright NK cells are more potent producers of the following cytokines: IFN gamma, TNF alpha, GM-CSF, IL10, IL13. As follows, CD56 serves as a diagnostic marker for NK-cell malignancies, but subpopulations of cells in any other hematological malignancy can also be positive for CD56. It is not completely understood whether, CD56 expression is inherited from the original normal cell, is a results of oncogenic mutations in malignant cells or the tumor microenvironment induces CD56 expression on leukemic cells (174).
Fischer et al. reported TRC [transfer RNA cysteine 4 (anticodon GCA)] gene rearrangements in almost all cases of CD56+ T-ALL and concluded that CD56+ leukemias could emerge from a bispecific T/NK progenitor (152).
Not known for leukemias, IL-15 serves as a powerful cytokine to boost CD56 expression in NK cells (175) and other immune cells, such as CD8+ T cells (176), the downstream signals being carried by Ras/MAPK, PI3K/Akt and JAK/STAT pathways (177). Fuhrmann et al. analyzed CD56 expression in a cohort of T-ALL patients. The genetic mutations found in the CD56+ group, that carry the mutations NOTCH1, PTEN and FBXW7, appear to have a similar distribution as for CD56− group such that they cannot link that immunophenotype to any genetic mutation. Also, the clinical progression of ALL showed significant differences only when patients are stratified according to CD56 expression profile (178).
Because AML show CD56 positivity in about 20% of cases and RUNX1 mutations are so frequent, Gattenloehner et al. also concluded that these mutations increase CD56 expression and disease aggressiveness (179). When RUNX1-coded wild-type p48 isoform is overexpressed, it exerts a potent stimulatory effect on NCAM gene promoter and, together, the two proteins increase BCL-2 expression, thus protecting the AML cell from apoptosis. New RUNX1 isoforms were characterized (p38a, p30, and p24) and they caused the underexpression of NCAM gene (179).
CD56 is expressed on a small subset of T-ALL cell population of non-thymic phenotype and frequent T receptor rearrangement. Although the patients did not show any differences at initial diagnosis, a smaller proportion of them entered into complete remission in comparison with CD56− T-ALL patients and developed resistance more often (152).
For instance, Alegretti et al. report a mean OS of only 4.0 months in CD56+ patients when compared with 14.5 months in CD56- patients with AML (66). CD56 expression has with a higher frequency in subtypes of AML with aggressive behaviors, such M4 and M5 types (66), or AML with t(8;21) translocation (180).
In acute promyelocytic leukemia (APL), even if it is rarely expressed, CD56 was correlated with high WBC count, lower platelet count, severe intravascular coagulation, expression of CD2, CD7, CD34 and CD117, shorter event-free survival (EFS) and increased relapse incidence (181,182). CD56 positivity in acute leukemias was also found to increase the tendency to develop EMD or relapse (183). EMD show high frequency in M4 and M5 types of AML (184) and in AML with t(8;21) translocation (185).
Many studies associated CD56 positivity with increased incidence of CNS leukemia at diagnosis, but also at any time during disease, both in myeloid (183), and lymphoblastic acute leukemias (140,186).
CD56 could serve as a predictive marker for CNS leukemia. ALL patients with CD56+ cells developed ALL more rapidly and was more severe, considering that the OS of ALL patients with CNS involvement was lower for CD56+ cases (140). CD56 presence was associated with CNS leukemia at initial presentation with 19% of cases in CD56+ ALL patients versus 4% in CD56- ALL patients. Even in the case of treatment with 8 consecutive cycles of cyclophosphamide the CNS leukemia developed more often in CD56+ ALL cases. However it should be considered that there were only 16 CD56+ cases versus 184 CD56− case (186). A study analyzing the role of CD56+ in T-ALL concluded that there was no difference in CNS leukemia between CD56+ or CD56− T-ALL cases (152). A case presentation also reported the expression of CD56, along with CD34, CD19, CD79s in B cell precursor ALL (BCP-ALL) who had KMT2A-AFF1 gene rearrangement (187).
In AML, as stated above, there are less frequent cases of CNS involvement and the role of CD56 status is still debatable. However, CD56 positivity in AML leukemic blasts was associated with higher incidence of extramedullary involvement, including CNS infiltration (183). CNS relapse rate, and overall relapse rate too, are higher in CD56+ AML patients (180), especially second remission (188) including APL, especially if associated with high levels of WBC (189). According to a case report, in APL immunophenotype after CNS relapse, the cells express CD34 and CD56. Thorough a reanalysis of the bone marrow aspirate at the initial diagnosis a small subpopulation of CD34 and CD56 positive blasts was discovered (190). A careful analysis of CD56 expression at diagnosis could serve as a predictive marker to assess CNS relapse risk after complete molecular response. This is caused by CD56 expression on leukemic blasts that signals for the production of cytokine receptors that interact with cytokines secreted by astrocytes or other cells in the brain. Through this chemoattraction, leukemic cells infiltrate into the perivascular tissue and migrate in the neural tissue by CD56 homophilic interactions. These blasts also manipulate the microenvironment and establish neural stem cell niches. Their maintenance in such leukemic niches in a quiescent state could theoretically explain the high incidence of isolated CNS relapses, where cells show CD56 positivity (191).
Diagnosis and management of CNS disease in acute leukemias
Clinical presentation of CNS leukemia
As stated in the introduction, CNS involvement in acute leukemias remains under-diagnosed. The reason behind this is that the particularities of many cases are still overlooked, and current diagnostic tools are many times insufficient to detect the beginning of this complication. Symptomatic manifestations of CNS infiltration, although rare, are often subtle and attributed to other causes, as hyperleukocytosis, treatment related neuropathy, infections or brain parenchymal metastases (192). These symptoms depend of the leukemic infiltration and the number of sites involved.
In most cases, CNS involvement in acute leukemia is asymptomatic. Symptoms and signs on a neurological examination are present in half of patients with ≥5 leukocytes in the CSF. Moreover, 80% of patients with leukocytes in CSF below this number do not have any symptoms (193-195). The neurological symptoms depend on the localization of blasts and are many times atypical, such as headache, loss of balance, fainting, mood swings, seizures, nausea/vomiting and papilledema (12,196). Cranial nerves involvement is less common and can cause double vision (197), facial numbness, deafness, blindness, and swallowing difficulties (192). Another rare symptom, often overlooked, is the sudden onset of obesity, which can be caused by hypothalamic localization of leukemic cells (198).
Symptoms of spinal cord involvement are even more difficult to identify. Similar to CNS leukemia, different locations give different symptoms from back pain, legs or arms weakness, numbness or pain, and loss of bladder or bowel control (199,200).
Seldom, distinct tumors formed of infiltrated leukemic cells can be detected throughout the CNS (201).
CNS leukemia-diagnostic tools
At the MRI analysis, CNS leukemia can be seen as dispersed lesions in the brain with round edges and local edema that give an equal T1 signal and a long T2 signal. Typically, a contrast agent is used (202,203). However, many times it is hard to differentiate between these lesions and CNS infections or neurodegenerative disorders, which are also common in patients with acute leukemia (204,205). These imaging challenges, associated with the difficulties faced by the LP analysis oftentimes delay the diagnostic and the beginning of treatment in CNS leukemia patients, much to the disadvantage in the quality of life and survival of these patients (206,207).
The obstacles faced by pathological assessment are related to the risks of an LP analysis, especially traumatic LPs. However, nowadays new techniques are developed that may help minimize the risk, such as stereotactic biopsy (208,209). If this technique would be learned by more specialists, CNS leukemia would be diagnosed faster and more accurate (205).
Development of CNS leukemia involves a series of clinical characteristics at initial presentation or development of these characteristics over the course of the disease, in both ALL and AML. In AML, a high initial leukocyte count has been reported to be predictive of a CNS involvement (210). Sixty-eight of patients with elevated initial WBC count developed CNS leukemia, therefore screening LPs should be routinely obtained during induction therapy in these patients. Leukemia cells in the CSF at diagnosis seems to also be predictive for CNS infiltration, along with extramedullary AML without CNS localization. Men more frequently developed CNS dissemination than women at a three to one ratio. Another detected risk factor for CNS involvement is the presence of internal tandem duplication (ITD) in FLT3 gene associated with NPM1 gene mutations in leukemic cells (23,211).
It is essential to point out that risk factors and associations, although useful can still not replace the use of an LP and subsequent CSF pathological analysis. This remains the golden standard, which must be performed at the slightest suspicion of CNS involvement and whose technical application should be continuously improved (192). The difficulties raised by the technique start from the performance of LP, which can show high opening pressure. This is continued during sample processing, during which several indicators of CNS leukemia can be observed, but these are still not enough to put a diagnostic more than 5/mm3 white blood cell count, less than 60 mg/dL glucose and more than 50 mg/dL protein level. The diagnostic is formulated only after leukemic cells are detected on the analyzed slides of CSF (192). Still, many times the test give false negative results (212), due to the fact that there infiltrated leukemic cells are in very small number and they all circulate in a large volume of CFS from which only a small volume is taken; as follows it is very easy to sample CSF without malignant cells. In addition, the pathologies must be very accustomed with the morphology of leukemic cells, since these can be easily confused with normal white blood cells. In order to increase the sensitivity of CSF analysis, repeated LPs are recommended. The possibility of malignant cells coming from a solid tumor that has metastasized to the brain should also be eliminated (213).
In regard to the imaging techniques the most commonly used are computed tomography (CT) and gadolinium MRI (214-216). MRI is often preferred to CT (217). During initial MRI scanning, a T1, T2 weighted sequences with fat suppression are used to scan the entire CNS. Still, it was found that this imaging analysis can only detect 44% of B-cell CNS leukemia (218).
Flow cytometry immunophenotyping (FCI) is a very reliable alternative to cytomorphology and the combination between the two can significantly increase the chances of CNS detection. This is because FCI can detect leukemic blasts even in small number (219). Flow cytometry was used successfully in the detection of CNS involvement in ALL and can successfully distinct between low risk and high risk patients (220). This technique is very useful in detecting minimal residual disease thus proving to be a powerful tool in the monitorization of leukemic patients, by being able to detect 1 abnormal cell from 10,000 events (221-223), however much attention must be paid to sampling, storage and processing (224,225). First the CSF must be collected in tubes that do not contain anti-coagulants (such as EDTA, heparin treated tubes). Secondly, analysis must be performed in maximum 72 h and prior to analysis CSF must be centrifuged and only the pellet or the bottom phase should be inserted into the flow cytometer (226-228). Besides these, it is recommended to use a combination of 6–9 antibodies (229). Due to the ability to concentrate the sample, and high sensitivity, flow cytometry is sometimes considered to be superior cu the commonly used CSF pathology (226). FCI of the CSF increased the detection rate of CNS involvement of ALL approximately two times compared to cytomorphology. FCI of CSF is a very reliable method to determine CNS leukemia in cases of low cellularity. The status of “indeterminate cases” is low, meaning 8.3% and it includes technical flaws such as very low number of cells, inappropriate stanning, insufficient normal population of cells for appropriate comparison (230).
Histology is also predictive of a CNS involvement, with significantly higher incidence found among monocytic M4 and M5a. Among risk factors, inversion of chromosome 16, chromosome 11 abnormality, trisomy 8 (28), serum lysozyme (<30 IU) (231) and high lactate dehydrogenase activity (>25,000/mm3) (232) was also correlated with CNS leukemia.
In ALL, the same risk factors are valid: younger age (233), presence of leukemic cells in CSF (234) or elevated lactate dehydrogenase (232). In addition, mature B-ALL and T cell phenotype is correlated with increased incidence of CNS infiltration. The presence of cytogenetic abnormalities, may it be t(9;22) or t(4;11), are also high-risk markers (235).
Therapeutic option for CNS leukemia
CNS-targeted therapy significantly increased overall and disease-free survival in hematological malignancies (236). Usually, these therapies refer to intrathecal chemotherapy (IT) or CNS targeted radiotherapy (237). Nowadays, most protocols restrict radiotherapy to high-risk patients. In addition the dose are continuously adapted to the progression of the disease, with the scope of regaining normal hematological physiology in the patient’s organism as soon as possible. Elimination of MRD and monitorization of immune privileged sites, such as CNS are also incorporated.
As stated above, protection of immune privileged sites, such as CNS need careful monitorization. Because of the limited penetration of cytostatic drugs for the treatment of leukemia across the BBB, the CNS is like a protective environment for the leukemic cells. Different cell types of the neurovascular unit including vascular cells (endothelium and mural cells), glia (astrocytes, microglia), and neurons contribute to regulation of BBB permeability. The insufficient CNS accumulation of the drugs explains why CNS relapse is reported in 30% of adult patients with ALL (238) thus early measures must be taken to prevent new tumor formations in the brain (239), such as: IT, high‐dose systemic chemotherapy and craniospinal irradiation. The role of cranial craniospinal radiotherapy has become controversial because of its neurologic adverse events. However, recent studies state that IT and high-dose chemotherapy are enough to prevent CNS leukemia (240-243).
When assessing initial intrathecal therapy (ITT) followed by cranial irradiation compared with further IT, there is no significant differences between the groups in the overall event rate, the annual event rate, OS at 10 years. There were fewer isolated CNS relapses in the cranial irradiation group (4.9%) than in the ITT (7.1%) (P=0.03) (242,243). When comparing the addition of intravenous methotrexate to long-term ITT (up to 12 doses) or radiotherapy with ITT (up to 9 doses), there was a significant reduction of 17% (P=0.003) in the annual event rate with intravenous methotrexate. EFS at 10 years was higher in the group given intravenous methotrexate (68.1% versus 61.9%). The OS rates at 10 years did not differ significantly. The annual rates of non-CNS relapses were 17% lower in the methotrexate group (P=0.02), while the CNS and isolated CNS rates were both non significantly lower in this group (244-249).
A randomized control study concluded that the triple ITT, in comparison with intrathecal methotrexate (IT MTX) reduces the risk of isolated CNS leukemia, but the OS of patients remains lower than in the case of IT MTX. This is reasoned by the fact that leukemia inducible tumors can also form in other sites of the body and these are often overlooked. A combination of ITT and high doses of systemic chemotherapy are recommended. When comparing cranial irradiation combined with short-term ITT versus intravenous methotrexate combined with short-term ITT, neither the 10-year survival, nor EFS was significantly different between the groups. The CNS relapse rates were 62% lower in the cranial irradiation group (P=0.00005). When comparing different doses of radiotherapy (24 versus 18 or 21 Gy) combined with short-term ITT, there were no significant differences between the doses on any measure. Still, when comparing radiotherapy combined with short-term ITT with intravenous methotrexate combined with long-term ITT, there were no significant differences between the groups on any measure (250,251). Thus, radiotherapy can be replaced by long-term ITT and intravenous methotrexate as it reduces non-CNS relapses.
It is highly important for the drugs used in CNS preventive/treatment chemotherapy to be able to cross the BBB and distribute uniformly in CNS. The best known options until now are cytarabine and methotrexate (238,252). Steroids are also frequently used in addition to chemotherapeutic treatment. In this case dexamethasone is preferred to prednisone, because of a higher stability (253,254) and reported ability to lower CNS recurrence to 2% (255). Adequate concentration in CSF can be reached with etoposide and 6-mercaptopurine systemic administration, as well as L-asparaginase (256-258). Although the single use of chemotherapy is enough to prevent CNS relapse, this is often avoided, due to the severed side effects given by this treatment choice.
Treating a B-cell ALL with CNS disease—an example
As a proof-of-concept, of the theoretical background presented, we depict the case of a 24-year-old male presented in June 2013 for bilateral laterocervical adenopathy, associated with slight fever, dysphagia, cough, and unexplainable weight-loss. No other indications of a disease were present. Physical examination certified adenopathy, of approximately 2 cm in the laterocervical, submandibular, axillary and inguinal areas and grade II splenomegaly. Leucopenia (3.14×103/µL) with 22% blasts was detected upon blood analysis and the bone marrow aspirate found hypocellularity with maturation in granulocytic and erythroblastic lineages, but 60% blast cell infiltrate. The immunophenotyping analysis revealed a common Philadelphia chromosome negative (Ph-) preB-ALL (positive for: CD19, CD22, CD10, CD45, HLA-DR, CD34, CD33).
The HyperCVAD protocol was started, and the first complete remission (CR1) was obtained after the first cycle, however with MRD positivity. Clinicians decided to treat the MRD+ state with blinatumomab and the patient successfully achieved complete molecular response after 4 cycles. POMP (6-mercaptopurine, vincristine, methotrexate, prednisone) maintenance therapy was continued until December 2016, but the disease relapsed in July 2017, with 90% blasts in the bone marrow, anemia (hemoglobin =9.6 g/dL) and mild thrombocytopenia (103×103/µL). Chemotherapy was started again, following the same HyperCVAD protocol. However, this time a complete hematological response was not achieved, the patient remaining refractory with a low, but persistent level of blasts in the peripheral blood. Consequently, the family was tested for HLA compatibility, but were not compatible; as a consequence, he was put on the waiting-list for matched unrelated allogeneic HSCT. The Romanian National Drug Agency (ANM) approved in December 2017 the initiation of a second blinatumomab treatment, the patient being by this time in a second complete remission (CR2) after 2 cycles. Blinatumomab therapy was well tolerated, with only mild adverse effects (fever spikes, grade 1 neurotoxicity), successfully controlled with corticotherapy. A severe polyclonal hypogammaglobulinemia was present even 4 years post blinatumomab treatment (IgG =123 mg/dL, normal value 700–1,600 mg/dL), but with no severe infectious complications. Soon after CR2, the patient proceeded to an allogeneic stem cell transplantation (allo-SCT). Conditioning chemotherapy regimen consisted of busulfan and cyclophosphamide and post-transplant immunosuppression was done using methotrexate and tacrolimus (259-262). The patient rapidly recovered but developed grade 1 hepatic GVHD (graft versus host disease) that was treated with tacrolimus and methylprednisolone. He achieved complete post-transplant chimerism with no residual disease.
About one year after the allo-SCT, in July 2019, the patient presented in emergency with an episode of generalized seizures. He also associated dyspnea, sore throat and some involuntary movements of the left side of the face, but no adenomegaly or hepatosplenomegaly. The CT-scan was normal, and the CSF fluid was clean. Immunology assays and CSF virology tests for VZV, HHV-1, HHV-2, CMV and BKV were all negative, possible differential diagnoses remaining blinatumomab neurotoxicity and CNS relapse (262). A brain MRI was performed showing several millimetric demyelinating lesions scattered through the white matter, but also one bigger lesion in each precentral gyrus. The one in right precentral gyrus had 18/6 millimeters and his exact position was the superior facial area of the motor homunculus, possibly explaining the left facial involuntary movements. Both emit high signal in T2-weighted scan and are also seen in FLAIR scan, suggesting irreversible ischemic lesions. The MRI images are found in Figure 1. The patient state got worse, even with steroid and antiepileptic therapy, and a PET-CT performed in Vienna certified the diagnosis of isolated CNS relapse with extensive lesions. The positron emission tomography (PET-CT) images are found in Figure 2. He died soon thereafter, in September 2019.
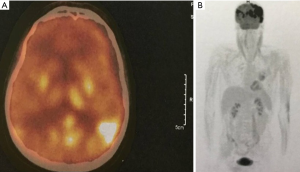
Conclusions
CNS leukemia is a severe effect, that is the leading cause of acute leukemia-related deaths and that is encountered often during the progression of this disease. The leukemic cells stimulate VEGF and de novo angiogenesis, roll through the bone marrow endothelial cells through adhesion molecules (the most important being CD56) and enter into the CNS through the blood vessels connecting bone marrow to the CNS. In the brain parenchyma, these malignant cells can travel to different locations thus they give a high variety of neurological symptoms.
Early diagnostic through a more common application of CSF analysis, improved MRI techniques and flow cytometry is the first step to an early detection of the disease. Practitioners should improve their techniques of LP and keep up-to date this the latest developments that would prevent complications. At the same time, the role of prophylaxis and early treatment is still underestimated. The best types of treatment are: targeted chemo-or radiation therapy to the CNS and high doses of systemic chemotherapy.
We have decided to show the case presented as a proof-of-concept. All the modern diagnostic protocols were used but physicians still failed to properly establish whether the immunocompromised patient has a CNS relapse or infection. Thus, a better, more targeted early diagnosis is crucial, for instance a combined use of flow cytometry and cytomorphology analysis of CSF.
Acknowledgments
The authors also gratefully acknowledge the insightful support of professor Berindan-Neagoe.
Funding: Dalma Deak was funded by an internal grant of the Iuliu Hatieganu University-School of Doctoral Studies (PCD 2019-2021). Ciprian Tomuleasa was also supported by two national research grants of the Romanian Government: the first one awarded for Frontiers Research Projects 2018-2022 (grant number PN-III-P4-ID-PCCF-2016-112), awarded to the Babes Bolyai University in collaboration with the Ion Chiricuta Oncology Institute Cluj Napoca; as well as a second one, awarded for Young Research Teams 2020-2022 (grant number PN-III-P1-1.1-TE-2019-0271) awarded to the Iuliu Hatieganu University of Medicine and Pharmacy Cluj Napoca. Ciprian Tomuleasa, Delia Dima, Alina Tanase and Olafur Sigurjonsson were also financed by an international collaborative grant of the European Economic Space between Romania and Iceland 2020–2022 (grant number 19-COP-0031).
Footnote
Reporting Checklist: The authors have completed the Narrative Review reporting checklist. Available at http://dx.doi.org/10.21037/atm-20-3140
Peer Review File: Available at http://dx.doi.org/10.21037/atm-20-3140
Conflicts of Interest: All authors have completed the ICMJE uniform disclosure form (available at http://dx.doi.org/10.21037/atm-20-3140). CT serves as an unpaid editorial board member of Annals of Translational Medicine from Nov 2019 to Oct 2021. The other authors have no conflicts of interest to declare.
Ethical Statement: The authors are accountable for all aspects of the work in ensuring that questions related to the accuracy or integrity of any part of the work are appropriately investigated and resolved.
Open Access Statement: This is an Open Access article distributed in accordance with the Creative Commons Attribution-NonCommercial-NoDerivs 4.0 International License (CC BY-NC-ND 4.0), which permits the non-commercial replication and distribution of the article with the strict proviso that no changes or edits are made and the original work is properly cited (including links to both the formal publication through the relevant DOI and the license). See: https://creativecommons.org/licenses/by-nc-nd/4.0/.
References
- Tatar AS, Jurj A, Tomuleasa C, et al. CD19-targeted, Raman tagged gold nanourchins as theranostic agents against acute lymphoblastic leukemia. Colloids Surf B Biointerfaces 2019;184:110478. [Crossref] [PubMed]
- Sas V, Blag C, Zaharie G, et al. Transient leukemia of Down syndrome. Crit Rev Clin Lab Sci 2019;56:247-59. [Crossref] [PubMed]
- Frey NV. Chimeric antigen receptor T cells for acute lymphoblastic leukemia. Am J Hematol 2019;94:S24-7. [Crossref] [PubMed]
- Dima D, Oprita L, Rosu AM, et al. Adult acute megakaryoblastic leukemia: rare association with cytopenias of undetermined significance and p210 and p190 BCR-ABL transcripts. Onco Targets Ther 2017;10:5047-51. [Crossref] [PubMed]
- Sas V, Moisoiu V, Teodorescu P, et al. Approach to the Adult Acute Lymphoblastic Leukemia Patient. J Clin Med 2019;8:1175. [Crossref] [PubMed]
- Gafencu GA, Tomuleasa CI, Ghiaur G. PARP inhibitors in acute myeloid leukaemia therapy: How a synthetic lethality approach can be a valid therapeutic alternative. Med Hypotheses 2017;104:30-4. [Crossref] [PubMed]
- Tatar AS, Nagy-Simon T, Tomuleasa C, et al. Nanomedicine approaches in acute lymphoblastic leukemia. J Control Release 2016;238:123-38. [Crossref] [PubMed]
- Munker R, Labopin M, Esteve J, et al. Mixed phenotype acute leukemia: outcomes with allogeneic stem cell transplantation. A retrospective study from the Acute Leukemia Working Party of the EBMT. Haematologica 2017;102:2134-40. [Crossref] [PubMed]
- Xie S, Hossain MJ. Survival differences in childhood and young adult acute myeloid leukemia: A cross-national study using US and England data. Cancer Epidemiol 2018;54:19-24. [Crossref] [PubMed]
- Ellison LF. Increasing survival from leukemia among adolescents and adults in Canada: A closer look. Health Rep 2016;27:19-26. [PubMed]
- Bailey C, Richardson LC, Allemani C, et al. Adult leukemia survival trends in the United States by subtype: A population-based registry study of 370,994 patients diagnosed during 1995-2009. Cancer 2018;124:3856-67. [Crossref] [PubMed]
- Johnston DL, Alonzo TA, Gerbing RB, et al. Central nervous system disease in pediatric acute myeloid leukemia: A report from the Children's Oncology Group. Pediatr Blood Cancer 2017. [Crossref] [PubMed]
- Constantinescu C, Bodolea C, Pasca S, et al. Clinical Approach to the Patient in Critical State Following Immunotherapy and/or Stem Cell Transplantation: Guideline for the On-Call Physician. J Clin Med 2019;8:884. [Crossref] [PubMed]
- Baytan B, Evim MS, Güler S, et al. Acute Central Nervous System Complications in Pediatric Acute Lymphoblastic Leukemia. Pediatr Neurol 2015;53:312-8. [Crossref] [PubMed]
- Lazarus HM, Richards SM, Chopra R, et al. Central nervous system involvement in adult acute lymphoblastic leukemia at diagnosis: results from the international ALL trial MRC UKALL XII/ECOG E2993. Blood 2006;108:465-72. [Crossref] [PubMed]
- Jabbour E, Thomas D, Cortes J, et al. Central nervous system prophylaxis in adults with acute lymphoblastic leukemia: current and emerging therapies. Cancer 2010;116:2290-300. [Crossref] [PubMed]
- Surapaneni UR, Cortes JE, Thomas D, et al. Central nervous system relapse in adults with acute lymphoblastic leukemia. Cancer 2002;94:773-9. [Crossref] [PubMed]
- Reman O, Pigneux A, Huguet F, et al. Central nervous system involvement in adult acute lymphoblastic leukemia at diagnosis and/or at first relapse: results from the GET-LALA group. Leuk Res 2008;32:1741-50. [Crossref] [PubMed]
- Gorchynski J, Oman J, Newton T. Interpretation of traumatic lumbar punctures in the setting of possible subarachnoid hemorrhage: who can be safely discharged? Cal J Emerg Med 2007;8:3-7. [PubMed]
- Barranco-Lampón G, Rozen-Fuller E, Olarte-Carrilo I, et al. Association between traumatic lumbar puncture and the risk of central nervous system relapse in adults with acute lymphoblastic leukaemia. Revista Médica del Hospital General de México.
- Rech A, de Carvalho GP, Meneses CF, et al. The influence of traumatic lumbar puncture and timing of intrathecal therapy on outcome of pediatric acute lymphoblastic leukemia. Pediatr Hematol Oncol 2005;22:483-8. [Crossref] [PubMed]
- Johnston DL, Alonzo TA, Gerbing RB, et al. The Effect of Traumatic Diagnostic Lumbar Puncture in De Novo Pediatric Acute Myeloid Leukemia - a Report from the Children's Oncology Group. Blood 2016;128:4016. [Crossref]
- Alakel N, Stölzel F, Mohr B, et al. Symptomatic central nervous system involvement in adult patients with acute myeloid leukemia. Cancer Manag Res 2017;9:97-102. [Crossref] [PubMed]
- Cheng CL, Li CC, Hou HA, et al. Risk factors and clinical outcomes of acute myeloid leukaemia with central nervous system involvement in adults. BMC Cancer 2015;15:344. [Crossref] [PubMed]
- Kaspers G, Zimmermann M, Reinhardt D, et al. Central Nervous System (CNS) Involvement In Pediatric Relapsed Acute Myeloid Leukemia: Results and Lessons From Study Relapsed AML 2001/01. Blood 2010;116:184. [Crossref]
- Döhner H, Estey EH, Amadori S, et al. Diagnosis and management of acute myeloid leukemia in adults: recommendations from an international expert panel, on behalf of the European LeukemiaNet. Blood 2010;115:453-74. [Crossref] [PubMed]
- Döhner H, Estey E, Grimwade D, et al. Diagnosis and management of AML in adults: 2017 ELN recommendations from an international expert panel. Blood 2017;129:424-47. [Crossref] [PubMed]
- Shihadeh F, Reed V, Faderl S, et al. Cytogenetic profile of patients with acute myeloid leukemia and central nervous system disease. Cancer 2012;118:112-7. [Crossref] [PubMed]
- Delaunay J, Vey N, Leblanc T, et al. Prognosis of inv(16)/t(16;16) acute myeloid leukemia (AML): a survey of 110 cases from the French AML Intergroup. Blood 2003;102:462-9. [Crossref] [PubMed]
- Linger RM, Lee-Sherick AB, DeRyckere D, et al. Mer receptor tyrosine kinase is a therapeutic target in pre-B-cell acute lymphoblastic leukemia. Blood 2013;122:1599-609. [Crossref] [PubMed]
- Jonart LM, Ebadi M, Basile P, et al. Disrupting the leukemia niche in the central nervous system attenuates leukemia chemoresistance. Haematologica 2020;105:2130-40. [Crossref] [PubMed]
- Alsadeq A, Lenk L, Vadakumchery A, et al. IL7R is associated with CNS infiltration and relapse in pediatric B-cell precursor acute lymphoblastic leukemia. Blood 2018;132:1614-7. [Crossref] [PubMed]
- Peterson BA, Brunning RD, Bloomfield CD, et al. Central nervous system involvement in acute nonlymphocytic leukemia: A prospective study of adults in remission. Am J Med 1987;83:464-70. [Crossref] [PubMed]
- Cole N, Gibson BE. High-dose cytosine arabinoside in the treatment of acute myeloid leukaemia. Blood Rev 1997;11:39-45. [Crossref] [PubMed]
- Reese ND, Schiller GJ. High-Dose Cytarabine (HD araC) in the Treatment of Leukemias: a Review. Curr Hematol Malig Rep 2013;8:141-8. [Crossref] [PubMed]
- Chamberlain MC, Nolan C, Abrey LE. Leukemic and lymphomatous meningitis: incidence, prognosis and treatment. J Neurooncol 2005;75:71-83. [Crossref] [PubMed]
- Cervantes GM, Cayci Z. Intracranial CNS Manifestations of Myeloid Sarcoma in Patients with Acute Myeloid Leukemia: Review of the Literature and Three Case Reports from the Author's Institution. J Clin Med 2015;4:1102-12. [Crossref] [PubMed]
- Patkowska E, Szczepaniak A, Barańska M, et al. Primary and Secondary Central Nervous System Involvement in Acute Myeloid Leukemia. J Leuk 2019. [Crossref]
- Yanagisawa B, Ghiaur G, Smith BD, et al. Translating leukemia stem cells into the clinical setting: Harmonizing the heterogeneity. Exp Hematol 2016;44:1130-7. [Crossref] [PubMed]
- Aldea MD, Petrushev B, Soritau O, et al. Metformin plus sorafenib highly impacts temozolomide resistant glioblastoma stem-like cells. J buon 2014;19:502-11. [PubMed]
- Frinc I, Muresan MS, Zaharie F, et al. Cancer stem-like cells: the dark knights of clinical hematology and oncology. J BUON 2014;19:328-35. [PubMed]
- Rani B, Cao Y, Malfettone A, et al. Role of the tissue microenvironment as a therapeutic target in hepatocellular carcinoma. World J Gastroenterol 2014;20:4128-40. [Crossref] [PubMed]
- Ghiaur G, Gerber J, Jones RJ. Concise review: Cancer stem cells and minimal residual disease. Stem cells (Dayton, Ohio) 2012;30:89-93. [Crossref] [PubMed]
- Ghiaur G, Gerber JM, Matsui W, et al. Cancer stem cells: relevance to clinical transplantation. Curr Opin Oncol 2012;24:170-5. [Crossref] [PubMed]
- Chopra M, Bohlander SK. The cell of origin and the leukemia stem cell in acute myeloid leukemia. Genes Chromosomes Cancer 2019;58:850-8. [Crossref] [PubMed]
- Bernt KM, Armstrong SA. Leukemia stem cells and human acute lymphoblastic leukemia. Semin Hematol 2009;46:33-8. [Crossref] [PubMed]
- Roboz GJ, Guzman M. Acute myeloid leukemia stem cells: seek and destroy. Expert Rev Hematol 2009;2:663-72. [Crossref] [PubMed]
- Wang X, Huang S, Chen JL. Understanding of leukemic stem cells and their clinical implications. Mol Cancer 2017;16:2. [Crossref] [PubMed]
- Fukuda S, Onishi C, Pelus LM. Trafficking of Acute Leukemia Cells – Chemokine Receptor Pathways that Modulate Leukemia Cell Dissemination. In: Antica M, editor. Acute Leukemia.The Scientist's Perspective and Challenge. Intechopen; 2010.
- Hanekamp D, Cloos J, Schuurhuis GJ. Leukemic stem cells: identification and clinical application. Int J Hematol 2017;105:549-57. [Crossref] [PubMed]
- Lenk L, Alsadeq A, Schewe DM. Involvement of the central nervous system in acute lymphoblastic leukemia: opinions on molecular mechanisms and clinical implications based on recent data. Cancer Metastasis Rev 2020;39:173-87. [Crossref] [PubMed]
- Passaro D, Di Tullio A, Abarrategi A, et al. Increased Vascular Permeability in the Bone Marrow Microenvironment Contributes to Disease Progression and Drug Response in Acute Myeloid Leukemia. Cancer Cell 2017;32:324-41.e6. [Crossref] [PubMed]
- Shalapour S, Hof J, Kirschner-Schwabe R, et al. High VLA-4 expression is associated with adverse outcome and distinct gene expression changes in childhood B-cell precursor acute lymphoblastic leukemia at first relapse. Haematologica 2011;96:1627-35. [Crossref] [PubMed]
- Becker PS, Kopecky KJ, Wilks AN, et al. Very late antigen-4 function of myeloblasts correlates with improved overall survival for patients with acute myeloid leukemia. Blood 2009;113:866-74. [Crossref] [PubMed]
- Bendall LJ, Makrynikola V, Hutchinson A, et al. Stem cell factor enhances the adhesion of AML cells to fibronectin and augments fibronectin-mediated anti-apoptotic and proliferative signals. Leukemia 1998;12:1375-82. [Crossref] [PubMed]
- Zhang W, Zhang X, Fan X, et al. Effect of ICAM-1 and LFA-1 in hyperleukocytic acute myeloid leukaemia. Clin Lab Haematol 2006;28:177-82. [Crossref] [PubMed]
- Gruszka AM, Valli D, Restelli C, et al. Adhesion Deregulation in Acute Myeloid Leukaemia. Cells 2019;8:66. [Crossref] [PubMed]
- Graf M, Reif S, Kröll T, et al. Expression of MAC-1 (CD11b) in acute myeloid leukemia (AML) is associated with an unfavorable prognosis. Am J Hematol 2006;81:227-35. [Crossref] [PubMed]
- Aref S, Salama O, Al-Tonbary Y, et al. L and E selectins in acute myeloid leukemia: expression, clinical relevance and relation to patient outcome. Hematology 2002;7:83-7. [Crossref] [PubMed]
- Graf M, Reif S, Hecht K, et al. Low L-selectin (CD62L) expression in acute myeloid leukemia correlates with a bad cytogenetic risk. Eur J Haematol 2003;71:366-76. [Crossref] [PubMed]
- Herault O, Gallay N, Domenech J, et al. The Extramedullar Dissemination of Acute Myeloid Leukemic Cells Depends on CD31 (PECAM-1) and CD38 Coexpression Level. Blood 2006;108:1896. [Crossref]
- Oyan AM, Bø TH, Jonassen I, et al. CD34 expression in native human acute myelogenous leukemia blasts: differences in CD34 membrane molecule expression are associated with different gene expression profiles. Cytometry B Clin Cytom 2005;64:18-27. [Crossref] [PubMed]
- Mattes K, Gerritsen M, Folkerts H, et al. CD34 + acute myeloid leukemia cells with low levels of reactive oxygen species show increased expression of stemness genes and can be targeted by the BCL2 inhibitor venetoclax. Haematologica 2020;105:e399-e403. [Crossref] [PubMed]
- Quéré R, Andradottir S, Brun AC, et al. High levels of the adhesion molecule CD44 on leukemic cells generate acute myeloid leukemia relapse after withdrawal of the initial transforming event. Leukemia 2011;25:515-26. [Crossref] [PubMed]
- Chen P, Huang H, Wu J, et al. Bone marrow stromal cells protect acute myeloid leukemia cells from anti-CD44 therapy partly through regulating PI3K/Akt–p27Kip1 axis. Mol Carcinog 2015;54:1678-85. [Crossref] [PubMed]
- Alegretti AP, Bittar CM, Bittencourt R, et al. The expression of CD56 antigen is associated with poor prognosis in patients with acute myeloid leukemia. Rev Bras Hematol Hemoter 2011;33:202-6. [Crossref] [PubMed]
- Yoo HJ, Lee JS, Kim JE, et al. Extracellular Histone Released from Leukemic Cells Increases Their Adhesion to Endothelium and Protects them from Spontaneous and Chemotherapy-Induced Leukemic Cell Death. PLoS One 2016;11:e0163982. [Crossref] [PubMed]
- Kasaai B, Caolo V, Peacock HM, et al. Erythro-myeloid progenitors can differentiate from endothelial cells and modulate embryonic vascular remodeling. Sci Rep 2017;7:43817. [Crossref] [PubMed]
- Gomez Perdiguero E, Klapproth K, Schulz C, et al. Tissue-resident macrophages originate from yolk-sac-derived erythro-myeloid progenitors. Nature 2015;518:547-51. [Crossref] [PubMed]
- Plein A, Fantin A, Denti L, et al. Erythro-myeloid progenitors contribute endothelial cells to blood vessels. Nature 2018;562:223-8. [Crossref] [PubMed]
- Pezeshkian B, Donnelly C, Tamburo K, et al. Leukemia Mediated Endothelial Cell Activation Modulates Leukemia Cell Susceptibility to Chemotherapy through a Positive Feedback Loop Mechanism. PLoS One 2013;8:e60823. [Crossref] [PubMed]
- Zhou X, Zhou S, Li B, et al. Transmembrane TNF-α preferentially expressed by leukemia stem cells and blasts is a potent target for antibody therapy. Blood 2015;126:1433-42. [Crossref] [PubMed]
- Kornblau SM, McCue D, Singh N, et al. Recurrent expression signatures of cytokines and chemokines are present and are independently prognostic in acute myelogenous leukemia and myelodysplasia. Blood 2010;116:4251-61. [Crossref] [PubMed]
- Barbier V, Erbani J, Fiveash C, et al. Endothelial E-selectin inhibition improves acute myeloid leukaemia therapy by disrupting vascular niche-mediated chemoresistance. Nat Commun 2020;11:2042. [Crossref] [PubMed]
- Bruserud Ø, Foss B, Ulvestad E, et al. Effects of acute myelogenous leukemia blasts on platelet release of soluble P-selectin and platelet-derived growth factor. Platelets 1998;9:352-8. [Crossref] [PubMed]
- Spertini C, Baïsse B, Bellone M, et al. Acute Myeloid and Lymphoblastic Leukemia Cell Interactions with Endothelial Selectins: Critical Role of PSGL-1, CD44 and CD43. Cancers (Basel) 2019;11:1253. [Crossref] [PubMed]
- Yamada Y, Takakura N. Physiological pathway of differentiation of hematopoietic stem cell population into mural cells. J Exp Med 2006;203:1055-65. [Crossref] [PubMed]
- Stucki A, Rivier AS, Gikic M, et al. Endothelial cell activation by myeloblasts: molecular mechanisms of leukostasis and leukemic cell dissemination. Blood 2001;97:2121-9. [Crossref] [PubMed]
- Bendall LJ, Kortlepel K, Gottlieb DJ. Bone marrow fibroblast exposure to the inflammatory cytokines tumor necrosis factor-alpha and interferon-gamma increases adhesion of acute myeloid leukemia cells and alters the adhesive mechanism. Exp Hematol 1997;25:132-9. [PubMed]
- Morceau F, Dicato M, Diederich M. Pro-inflammatory cytokine-mediated anemia: regarding molecular mechanisms of erythropoiesis. Mediators Inflamm 2009;2009:405016. [Crossref] [PubMed]
- Podolnikova NP, Kushchayeva YS, Wu Y, et al. The Role of Integrins αMβ2 (Mac-1, CD11b/CD18) and αDβ2 (CD11d/CD18) in Macrophage Fusion. Am J Pathol 2016;186:2105-16. [Crossref] [PubMed]
- Walzog B, Schuppan D, Heimpel C, et al. The leukocyte integrin Mac-1 (CD11b/CD18) contributes to binding of human granulocytes to collagen. Exp Cell Res 1995;218:28-38. [Crossref] [PubMed]
- Bernatchez SF, Atkinson MR, Parks PJ. Expression of intercellular adhesion molecule-1 on macrophages in vitro as a marker of activation. Biomaterials 1997;18:1371-8. [Crossref] [PubMed]
- Hyun Y-M, Choe YH, Park SA, et al. LFA-1 (CD11a/CD18) and Mac-1 (CD11b/CD18) distinctly regulate neutrophil extravasation through hotspots I and II. Exp Mol Med 2019;51:1-13. [Crossref] [PubMed]
- Jin L, Hope KJ, Zhai Q, et al. Targeting of CD44 eradicates human acute myeloid leukemic stem cells. Nat Med 2006;12:1167-74. [Crossref] [PubMed]
- Krause DS, Lazarides K, Lewis JB, et al. Selectins and their ligands are required for homing and engraftment of BCR-ABL1+ leukemic stem cells in the bone marrow niche. Blood 2014;123:1361-71. [Crossref] [PubMed]
- Mahlknecht U, Schönbein C. Histone deacetylase inhibitor treatment downregulates VLA-4 adhesion in hematopoietic stem cells and acute myeloid leukemia blast cells. Haematologica 2008;93:443-6. [Crossref] [PubMed]
- Konopleva M, Tabe Y, Zeng Z, et al. Therapeutic targeting of microenvironmental interactions in leukemia: mechanisms and approaches. Drug Resist Updat 2009;12:103-13. [Crossref] [PubMed]
- Forte D, Krause DS, Andreeff M, et al. Updates on the hematologic tumor microenvironment and its therapeutic targeting. Haematologica 2019;104:1928-34. [Crossref] [PubMed]
- Kumar B, Garcia M, Weng L, et al. Acute myeloid leukemia transforms the bone marrow niche into a leukemia-permissive microenvironment through exosome secretion. Leukemia 2018;32:575-87. [Crossref] [PubMed]
- Arias-Salgado EG, Lizano S, Sarkar S, et al. Src kinase activation by direct interaction with the integrin beta cytoplasmic domain. Proc Natl Acad Sci U S A 2003;100:13298-302. [Crossref] [PubMed]
- Guan JL. Integrin signaling through FAK in the regulation of mammary stem cells and breast cancer. IUBMB Life 2010;62:268-76. [Crossref] [PubMed]
- Yen CF, Wang HS, Lee CL, et al. Roles of integrin-linked kinase in cell signaling and its perspectives as a therapeutic target. Gynecol Minim Invasive Ther 2014;3:67-72. [Crossref]
- Cacciola RR, Stagno F, Impera S, et al. Beta-1-integrin expression in adult acute lymphoblastic leukemia: possible relationship with the stem cell antigen CD34. Acta Haematol 1997;97:63-6. [Crossref] [PubMed]
- Scharff BF, Modvig S, Marquart HV, et al. Integrin-Mediated Adhesion and Chemoresistance of Acute Lymphoblastic Leukemia Cells Residing in the Bone Marrow or the Central Nervous System. Front Oncol 2020;10:775. [Crossref] [PubMed]
- Chittasupho C, Manikwar P, Krise JP, et al. cIBR effectively targets nanoparticles to LFA-1 on acute lymphoblastic T cells. Mol Pharm 2010;7:146-55. [Crossref] [PubMed]
- Mielcarek M, Sperling C, Schrappe M, et al. Expression of intercellular adhesion molecule 1 (ICAM-1) in childhood acute lymphoblastic leukaemia: correlation with clinical features and outcome. Br J Haematol 1997;96:301-7. [Crossref] [PubMed]
- Lee RV, Braylan RC, Rimsza LM. CD58 Expression Decreases as Nonmalignant B Cells Mature in Bone Marrow and Is Frequently Overexpressed in Adult and Pediatric Precursor B-Cell Acute Lymphoblastic Leukemia. Am J Clin Pathol 2005;123:119-24. [Crossref] [PubMed]
- Marques LVC, Noronha EP, Andrade FG, et al. CD44 Expression Profile Varies According to Maturational Subtypes and Molecular Profiles of Pediatric T-Cell Lymphoblastic Leukemia. Front Oncol 2018;8:488. [Crossref] [PubMed]
- Spertini O, Callegari P, Cordey A, et al. High levels of the shed form of L-selectin are present in patients with acute leukemia and inhibit blast cell adhesion to activated endothelium. Blood 1994;84:1249-56. [Crossref] [PubMed]
- Yao H, Price TT, Cantelli G, et al. Leukaemia hijacks a neural mechanism to invade the central nervous system. Nature 2018;560:55-60. [Crossref] [PubMed]
- Akers SM, O'Leary HA, Minnear FL, et al. VE-cadherin and PECAM-1 enhance ALL migration across brain microvascular endothelial cell monolayers. Exp Hematol 2010;38:733-43. [Crossref] [PubMed]
- Stefanidakis M, Karjalainen K, Jaalouk DE, et al. Role of leukemia cell invadosome in extramedullary infiltration. Blood 2009;114:3008-17. [Crossref] [PubMed]
- Stamatoullas A, Buchonnet G, Lepretre S, et al. De novo acute B cell leukemia/lymphoma with t(14;18). Leukemia 2000;14:1960-6. [Crossref] [PubMed]
- Fisher PG, Chiello C. Meningeal leukemia with cerebrospinal fluid block. Med Pediatr Oncol 2000;34:281-3. [Crossref] [PubMed]
- Delacroix R, Morel SNA, Hervé L, et al. Cerebrospinal fluid lens-free microscopy: a new tool for the laboratory diagnosis of meningitis. Sci. Rep 2017;7:39893. [Crossref] [PubMed]
- Gaudichon J, Jakobczyk H, Debaize L, et al. Mechanisms of extramedullary relapse in acute lymphoblastic leukemia: Reconciling biological concepts and clinical issues. Blood Reviews 2019;36:40-56. [Crossref] [PubMed]
- Bartram J, Goulden N, Wright G, et al. High throughput sequencing in acute lymphoblastic leukemia reveals clonal architecture of central nervous system and bone marrow compartments. Haematologica 2018;103:e110-4. [Crossref] [PubMed]
- Sinclair PB, Blair HH, Ryan SL, et al. Dynamic clonal progression in xenografts of acute lymphoblastic leukemia with intrachromosomal amplification of chromosome 21. Haematologica 2018;103:634-44. [Crossref] [PubMed]
- Chen YY, Huang CE, Chou HJ, et al. Mutant DNMT3A clone evading chemotherapy and infiltrating central nervous system in a patient with molecularly good-risk acute myeloid leukemia. Ann Hematol 2014;93:1441-2. [Crossref] [PubMed]
- Savino AM, Olivares O, Barel S, et al. Stearoyl-CoA Desaturase (SCD) Enhances Central Nervous System Leukemia. Blood 2018;132:389. [Crossref]
- Karpinsky G, Fatyga A, Krawczyk MA, et al. Osteopontin: its potential role in cancer of children and young adults. Biomark Med 2017;11:389-402. [Crossref] [PubMed]
- Pitt LA, Tikhonova AN, Hu H, et al. CXCL12-Producing Vascular Endothelial Niches Control Acute T Cell Leukemia Maintenance. Cancer Cell 2015;27:755-68. [Crossref] [PubMed]
- Winter SS, Sweatman JJ, Lawrence MB, et al. Enhanced T-lineage acute lymphoblastic leukaemia cell survival on bone marrow stroma requires involvement of LFA-1 and ICAM-1. Br J Haematol 2001;115:862-71. [Crossref] [PubMed]
- Kato I, Nishinaka Y, Nakamura M, et al. Hypoxic adaptation of leukemic cells infiltrating the CNS affords a therapeutic strategy targeting VEGFA. Blood 2017;129:3126-9. [Crossref] [PubMed]
- Semenza GL. Dynamic regulation of stem cell specification and maintenance by hypoxia-inducible factors. Mol Aspects Med 2016;47-48:15-23. [Crossref] [PubMed]
- Chiarini F, Lonetti A, Evangelisti C, et al. Advances in understanding the acute lymphoblastic leukemia bone marrow microenvironment: From biology to therapeutic targeting. Biochim Biophys Acta 2016;1863:449-63. [Crossref] [PubMed]
- Sugiyama T, Kohara H, Noda M, et al. Maintenance of the Hematopoietic Stem Cell Pool by CXCL12-CXCR4 Chemokine Signaling in Bone Marrow Stromal Cell Niches. Immunity 2006;25:977-88. [Crossref] [PubMed]
- Luo X, Tai WL, Sun L, et al. Crosstalk between astrocytic CXCL12 and microglial CXCR4 contributes to the development of neuropathic pain. Mol Pain 2016;12:1744806916636385. [Crossref] [PubMed]
- März M, Meyer S, Erb U, et al. Pediatric acute lymphoblastic leukemia-Conquering the CNS across the choroid plexus. Leuk Res 2018;71:47-54. [Crossref] [PubMed]
- Li M, Ransohoff RM. Multiple roles of chemokine CXCL12 in the central nervous system: a migration from immunology to neurobiology. Prog Neurobiol 2008;84:116-31. [Crossref] [PubMed]
- Velázquez-Avila M, Balandrán JC, Ramírez-Ramírez D, et al. High cortactin expression in B-cell acute lymphoblastic leukemia is associated with increased transendothelial migration and bone marrow relapse. Leukemia 2019;33:1337-48. [Crossref] [PubMed]
- Ma S, Shi Y, Pang Y, et al. Notch1-induced T cell leukemia can be potentiated by microenvironmental cues in the spleen. J Hematol Oncol 2014;7:71. [Crossref] [PubMed]
- Williams MTS, Yousafzai Y, Cox C, et al. Interleukin-15 enhances cellular proliferation and upregulates CNS homing molecules in pre-B acute lymphoblastic leukemia. Blood 2014;123:3116-27. [Crossref] [PubMed]
- Song G, Li Y, Jiang G. Role of VEGF/VEGFR in the pathogenesis of leukemias and as treatment targets Oncol Rep 2012;28:1935-44. (Review). [Crossref] [PubMed]
- Song M, Wang H, Ye Q. Increased circulating vascular endothelial growth factor in acute myeloid leukemia patients: a systematic review and meta-analysis. Syst Rev 2020;9:103. [Crossref] [PubMed]
- Dias S, Hattori K, Zhu Z, et al. Autocrine stimulation of VEGFR-2 activates human leukemic cell growth and migration. J Clin Invest 2000;106:511-21. [Crossref] [PubMed]
- Redondo-Muñoz J, García-Pardo A, Teixidó J. Molecular Players in Hematologic Tumor Cell Trafficking. Front Immunol 2019;10:156. [Crossref] [PubMed]
- Wen Y, Yang H, Liu Y, et al. Evaluation of BMMSCs-EPCs sheets for repairing alveolar bone defects in ovariectomized rats. Sci Rep 2017;7:16568. [Crossref] [PubMed]
- Erb U, Schwerk C, Schroten H, et al. Review of functional in vitro models of the blood-cerebrospinal fluid barrier in leukaemia research. J Neurosci Methods 2020;329:108478. [Crossref] [PubMed]
- Lazarevic I, Engelhardt B. Modeling immune functions of the mouse blood-cerebrospinal fluid barrier in vitro: primary rather than immortalized mouse choroid plexus epithelial cells are suited to study immune cell migration across this brain barrier. Fluids Barriers CNS 2016;13:2. [Crossref] [PubMed]
- Muench V, Trentin L, Herzig J, et al. Migration of Acute Lymphoblastic Leukemia Cells into the Central Nervous System Is Regulated By VEGF. Blood 2015;126:2634. [Crossref]
- Poyer F, Coquerel B, Pegahi R, et al. Secretion of MMP-2 and MMP-9 induced by VEGF autocrine loop correlates with clinical features in childhood acute lymphoblastic leukemia. Leuk Res 2009;33:407-17. [Crossref] [PubMed]
- Lyu CJ, Rha SY, Won SC. Clinical role of bone marrow angiogenesis in childhood acute lymphocytic leukemia. Yonsei Med J 2007;48:171-5. [Crossref] [PubMed]
- Kinjyo I, Bragin D, Grattan R, et al. Leukemia-derived exosomes and cytokines pave the way for entry into the brain. J Leukoc Biol 2019;105:741-53. [Crossref] [PubMed]
- Cheloni G, Poteti M, Bono S, et al. The Leukemic Stem Cell Niche: Adaptation to "Hypoxia" versus Oncogene Addiction. Stem Cells Int 2017;2017:4979474. [Crossref] [PubMed]
- Decimo I, Bifari F, Krampera M, et al. Neural stem cell niches in health and diseases. Curr Pharm Des 2012;18:1755-83. [Crossref] [PubMed]
- Gaynes JS, Jonart LM, Zamora EA, et al. The Central Nervous System Microenvironment Influences the Leukemia Transcriptome and Enhances Leukemia Chemo-Resistance. Haematologica 2017;102:e136-e139. [Crossref] [PubMed]
- Frishman-Levy L, Izraeli S. Advances in understanding the pathogenesis of CNS acute lymphoblastic leukaemia and potential for therapy. Br J Haematol 2017;176:157-67. [Crossref] [PubMed]
- Hu W, Wang X, Yang R, et al. Expression of CD56 is a risk factor for acute lymphocytic leukemia with central nervous system involvement in adults. Hematology 2017;22:81-7. [Crossref] [PubMed]
- Aref S, Azmy E, El-Bakry K, et al. Prognostic impact of CD200 and CD56 expression in pediatric B-cell acute lymphoblastic leukemia patients. Pediatr Hematol Oncol 2017;34:275-85. [Crossref] [PubMed]
- Theodorakos I, Paterakis G, Papadakis V, et al. Interference of bone marrow CD56+ mesenchymal stromal cells in minimal residual disease investigation of neuroblastoma and other CD45−/CD56+ pediatric malignancies using flow cytometry. Pediatr Blood Cancer 2019;66:e27799. [Crossref] [PubMed]
- Jang JE, Hwang DY, Chung H, et al. Early Cytomegalovirus Reactivation and Expansion of CD56brightCD16dim/−DNAM1+ Natural Killer Cells Are Associated with Antileukemia Effect after Haploidentical Stem Cell Transplantation in Acute Leukemia. Biol. Blood Marrow Transplant 2019;25:2070-8. [Crossref] [PubMed]
- Leshchyns'ka I, Liew HT, Shepherd C, et al. Aβ-dependent reduction of NCAM2-mediated synaptic adhesion contributes to synapse loss in Alzheimer's disease. Nat Commun 2015;6:8836. [Crossref] [PubMed]
- Suzuki M, Angata K, Nakayama J, et al. Polysialic acid and mucin type o-glycans on the neural cell adhesion molecule differentially regulate myoblast fusion. J Biol Chem 2003;278:49459-68. [Crossref] [PubMed]
- Van Acker HH, Capsomidis A, Smits EL, et al. CD56 in the Immune System: More Than a Marker for Cytotoxicity? Front Immunol 2017;8:892. [Crossref] [PubMed]
- Jensen M, Berthold F. Targeting the neural cell adhesion molecule in cancer. Cancer Lett 2007;258:9-21. [Crossref] [PubMed]
- Montero I, Rios E, Parody R, et al. CD56 in T-cell acute lymphoblastic leukaemia: a malignant transformation of an early myeloid-lymphoid progenitor? Haematologica 2003;88:ELT26. [PubMed]
- Farinola MA, Weir EG, Ali SZ. CD56 expression of neuroendocrine neoplasms on immunophenotyping by flow cytometry: a novel diagnostic approach to fine-needle aspiration biopsy. Cancer 2003;99:240-6. [Crossref] [PubMed]
- Gardner LJ, Polski JM, Fallon R, et al. Identification of CD56 and CD57 by flow cytometry in Ewing's sarcoma or primitive neuroectodermal tumor. Virchows Arch 1998;433:35-40. [Crossref] [PubMed]
- Sedick Q, Alotaibi S, Alshieban S, et al. Natural Killer Cell Lymphoblastic Leukaemia/Lymphoma: Case Report and Review of the Recent Literature. Case Rep Oncol 2017;10:588-95. [Crossref] [PubMed]
- Fischer L, Gökbuget N, Schwartz S, et al. CD56 expression in T-cell acute lymphoblastic leukemia is associated with non-thymic phenotype and resistance to induction therapy but no inferior survival after risk-adapted therapy. Haematologica 2009;94:224-9. [Crossref] [PubMed]
- Raspadori D, Damiani D, Lenoci M, et al. CD56 antigenic expression in acute myeloid leukemia identifies patients with poor clinical prognosis. Leukemia 2001;15:1161-4. [Crossref] [PubMed]
- Pardo LM, Voigt AP, Alonzo TA, et al. Deciphering the Significance of CD56 Expression in Pediatric Acute Myeloid Leukemia: A Report from the Children's Oncology Group. Cytometry B Clin Cytom 2020;98:52-6. [Crossref] [PubMed]
- Gattenlöhner S, Stühmer T, Leich E, et al. Specific detection of CD56 (NCAM) isoforms for the identification of aggressive malignant neoplasms with progressive development. Am J Pathol 2009;174:1160-71. [Crossref] [PubMed]
- Valgardsdottir R, Capitanio C, Texido G, et al. Direct involvement of CD56 in cytokine-induced killer-mediated lysis of CD56+ hematopoietic target cells. Exp Hematol 2014;42:1013-21.e1. [Crossref] [PubMed]
- Guan F, Wang X, He F. Promotion of cell migration by neural cell adhesion molecule (NCAM) is enhanced by PSA in a polysialyltransferase-specific manner. PLoS One 2015;10:e0124237. [Crossref] [PubMed]
- Tur MK, Etschmann B, Benz A, et al. The 140-kD isoform of CD56 (NCAM1) directs the molecular pathogenesis of ischemic cardiomyopathy. Am J Pathol 2013;182:1205-18. [Crossref] [PubMed]
- Životić M, Tampe B, Müller G, et al. Modulation of NCAM/FGFR1 signaling suppresses EMT program in human proximal tubular epithelial cells. PloS one 2018;13:e0206786. [Crossref] [PubMed]
- Shi Y, Xia YY, Wang L, et al. Neural cell adhesion molecule modulates mesenchymal stromal cell migration via activation of MAPK/ERK signaling. Exp Cell Res 2012;318:2257-67. [Crossref] [PubMed]
- Christensen C, Berezin V, Bock E. Neural cell adhesion molecule differentially interacts with isoforms of the fibroblast growth factor receptor. Neuroreport 2011;22:727-32. [Crossref] [PubMed]
- Schmid RS, Graff RD, Schaller MD, et al. NCAM stimulates the Ras-MAPK pathway and CREB phosphorylation in neuronal cells. J Neurobiol 1999;38:542-58. [Crossref] [PubMed]
- Walmod PS, Kolkova K, Berezin V, et al. Zippers make signals: NCAM-mediated molecular interactions and signal transduction. Neurochem Res 2004;29:2015-35. [Crossref] [PubMed]
- Ditlevsen DK, Køhler LB, Pedersen MV, et al. The role of phosphatidylinositol 3-kinase in neural cell adhesion molecule-mediated neuronal differentiation and survival. J Neurochem 2003;84:546-56. [Crossref] [PubMed]
- Kolkova K, Novitskaya V, Pedersen N, et al. Neural cell adhesion molecule-stimulated neurite outgrowth depends on activation of protein kinase C and the Ras-mitogen-activated protein kinase pathway. J Neurosci 2000;20:2238-46. [Crossref] [PubMed]
- Kolkova K, Stensman H, Berezin V, et al. Distinct roles of PKC isoforms in NCAM-mediated neurite outgrowth. J Neurochem 2005;92:886-94. [Crossref] [PubMed]
- Ma TC, Barco A, Ratan RR, et al. cAMP-responsive element-binding protein (CREB) and cAMP co-regulate activator protein 1 (AP1)-dependent regeneration-associated gene expression and neurite growth. J Biol Chem 2014;289:32914-25. [Crossref] [PubMed]
- Jessen U, Novitskaya V, Pedersen N, et al. The transcription factors CREB and c-Fos play key roles in NCAM-mediated neuritogenesis in PC12-E2 cells. J Neurochem 2001;79:1149-60. [Crossref] [PubMed]
- Simpson CS, Morris BJ. Regulation of neuronal cell adhesion molecule expression by NF-kappa B. J Biol Chem 2000;275:16879-84. [Crossref] [PubMed]
- Mace EM, Gunesch JT, Dixon A, et al. Human NK cell development requires CD56-mediated motility and formation of the developmental synapse. Nat Commun 2016;7:12171. [Crossref] [PubMed]
- Ziegler S, Weiss E, Schmitt AL, et al. CD56 Is a Pathogen Recognition Receptor on Human Natural Killer Cells. Sci Rep 2017;7:6138. [Crossref] [PubMed]
- Cohavy O, Targan SR. CD56 marks an effector T cell subset in the human intestine. J Immunol 2007;178:5524-32. [Crossref] [PubMed]
- Lu H, Jin LP, Huang HL, et al. Trophoblast-derived CXCL12 promotes CD56 bright CD82 - CD29 + NK cell enrichment in the decidua. Am J Reprod Immunol 2020. [Crossref] [PubMed]
- Jung HR, Kim MJ, Wee YM, et al. CD56+CD57+ infiltrates as the most predominant subset of intragraft natural killer cells in renal transplant biopsies with antibody-mediated rejection. Sci Rep 2019;9:16606. [Crossref] [PubMed]
- Wagner JA, Rosario M, Romee R, et al. CD56bright NK cells exhibit potent antitumor responses following IL-15 priming. J Clin Investig 2017;127:4042-58. [Crossref] [PubMed]
- Correia MP, Costa AV, Uhrberg M, et al. IL-15 induces CD8+ T cells to acquire functional NK receptors capable of modulating cytotoxicity and cytokine secretion. Immunobiology 2011;216:604-12. [Crossref] [PubMed]
- Mishra A, Sullivan L, Caligiuri MA. Molecular pathways: interleukin-15 signaling in health and in cancer. Clin Cancer Res 2014;20:2044-50. [Crossref] [PubMed]
- Fuhrmann S, Schabath R, Möricke A, et al. Expression of CD56 defines a distinct subgroup in childhood T-ALL with inferior outcome. Results of the ALL-BFM 2000 trial. Br J Haematol 2018;183:96-103. [Crossref] [PubMed]
- Gattenloehner S, Chuvpilo S, Langebrake C, et al. Novel RUNX1 isoforms determine the fate of acute myeloid leukemia cells by controlling CD56 expression. Blood 2007;110:2027-33. [Crossref] [PubMed]
- Iriyama N, Hatta Y, Takeuchi J, et al. CD56 expression is an independent prognostic factor for relapse in acute myeloid leukemia with t(8;21). Leuk Res 2013;37:1021-6. [Crossref] [PubMed]
- Testa U, Lo-Coco F. Prognostic factors in acute promyelocytic leukemia: strategies to define high-risk patients. Ann Hematol 2016;95:673-80. [Crossref] [PubMed]
- Sobas M, Montesinos P, Boluda B, et al. An analysis of the impact of CD56 expression in de novo acute promyelocytic leukemia patients treated with upfront all-trans retinoic acid and anthracycline-based regimens. Leuk. Lymphoma 2019;60:1030-5. [Crossref] [PubMed]
- Chang H, Brandwein J, Yi QL, et al. Extramedullary infiltrates of AML are associated with CD56 expression, 11q23 abnormalities and inferior clinical outcome. Leuk. Res. 2004;28:1007-11. [Crossref] [PubMed]
- Harris AC, Kitko CL, Couriel DR, et al. Extramedullary relapse of acute myeloid leukemia following allogeneic hematopoietic stem cell transplantation: incidence, risk factors and outcomes. Haematologica 2013;98:179-84. [Crossref] [PubMed]
- Ganzel C, Manola J, Douer D, et al. Extramedullary Disease in Adult Acute Myeloid Leukemia Is Common but Lacks Independent Significance: Analysis of Patients in ECOG-ACRIN Cancer Research Group Trials, 1980-2008. J Clin Oncol 2016;34:3544-53. [Crossref] [PubMed]
- Ravandi F, Cortes J, Estrov Z, et al. CD56 expression predicts occurrence of CNS disease in acute lymphoblastic leukemia. Leuk Res 2002;26:643-9. [Crossref] [PubMed]
- Nagoshi H, Toishigawa K, Inoue T, et al. CD56-positive B cell precursor acute lymphoblastic leukemia harboring KMT2A-AFF1 rearrangement developed in a pregnant woman successfully treated with allogeneic hematopoietic cell transplantation. J Hematop 2020;13:47-9. [Crossref]
- Chaudhri NA, Almhareb F, Walter CU, et al. Expression of CD56 in Acute Myeloid Leukemia (AML) Is Associated with Poor Outcome When Patients Treated with Stem Cell Transplant in Second Remission but Not in the First Remission. Blood 2011;118:4880. [Crossref]
- Ono T, Takeshita A, Kishimoto Y, et al. Expression of CD56 is an unfavorable prognostic factor for acute promyelocytic leukemia with higher initial white blood cell counts. Cancer Sci 2014;105:97-104. [Crossref] [PubMed]
- Maenhout TM, Moreau E, Van Haute I, et al. Minimal Coexpression of CD34+/CD56+ in Acute Promyelocytic Leukemia Is Associated With Relapse. Am J Clin Pathol 2015;144:347-51. [Crossref] [PubMed]
- Skog MS, Nystedt J, Korhonen M, et al. Expression of neural cell adhesion molecule and polysialic acid in human bone marrow-derived mesenchymal stromal cells. Stem Cell Res Ther 2016;7:113. [Crossref] [PubMed]
- Del Principe MI, Maurillo L, Buccisano F, et al. Central nervous system involvement in adult acute lymphoblastic leukemia: diagnostic tools, prophylaxis, and therapy. Mediterr J Hematol Infect Dis 2014;6:e2014075. [Crossref] [PubMed]
- Gajendra S, Das RR, Sharma R. Isolated Central Nervous System (CNS) Relapse in Paediatric Acute Promyelocytic Leukaemia: A Systematic Review. J Clin Diagn Res 2017;11:XE05-XE08. [Crossref] [PubMed]
- Bürger B, Zimmermann M, Mann G, et al. Diagnostic cerebrospinal fluid examination in children with acute lymphoblastic leukemia: significance of low leukocyte counts with blasts or traumatic lumbar puncture. J Clin Oncol 2003;21:184-8. [Crossref] [PubMed]
- te Loo DM, Kamps WA, van der Does-van den Berg A, et al. Prognostic significance of blasts in the cerebrospinal fluid without pleiocytosis or a traumatic lumbar puncture in children with acute lymphoblastic leukemia: experience of the Dutch Childhood Oncology Group. J Clin Oncol 2006;24:2332-6. [Crossref] [PubMed]
- Pui CH, Robison LL, Look AT. Acute lymphoblastic leukaemia. Lancet 2008;371:1030-43. [Crossref] [PubMed]
- Fozza C, Dore F, Isoni MA, et al. Strabismus and diplopia in a patient with acute myeloid leukemia. Am J Case Rep 2014;15:288-90. [Crossref] [PubMed]
- Zhang LD, Li YH, Ke ZY, et al. Obesity as the initial manifestation of central nervous system relapse of acute lymphoblastic leukemia: case report and literature review. J Cancer Res Ther 2012;8:151-3. [Crossref] [PubMed]
- Koh HJ, Baek J, Lee MS, et al. Epidural chloroma and spinal cord compression. Chin Med J (Engl) 2019;132:853-5. [Crossref] [PubMed]
- Grisold W. Leukemia and the Peripheral Nervous System: A review. J Leuk 2014. [Crossref]
- Lusher JM. Chloroma as a Presenting Feature of Acute Leukemia: A Report of Two Cases in Children. Am J Dis Child 1964;108:62-6. [Crossref] [PubMed]
- Chen CY, Zimmerman RA, Faro S, et al. Childhood leukemia: central nervous system abnormalities during and after treatment. AJNR Am J Neuroradiol 1996;17:295-310. [PubMed]
- Porto L, Kieslich M, Schwabe D, et al. Central nervous system imaging in childhood leukaemia. Eur J Cancer 2004;40:2082-90. [Crossref] [PubMed]
- Faraci M, Lanino E, Dini G, et al. Severe neurologic complications after hematopoietic stem cell transplantation in children. Neurology 2002;59:1895-904. [Crossref] [PubMed]
- Liu J, Wang Y, Sun X, et al. Lesions of the central nervous system in leukemia: Pathological and magnetic resonance imaging features at presentation in 14 patients. Oncol Lett 2017;14:8162-70. [Crossref] [PubMed]
- Kumar R, Nijalingappa S, Grainger J, et al. Acute disseminated encephalomyelitis mimicking late CNS relapse of acute lymphoblastic leukaemia: case report. J Med Case Rep 2007;1:4. [Crossref] [PubMed]
- Thastrup M, Marquart HV, Levinsen M, et al. Flow cytometric detection of leukemic blasts in cerebrospinal fluid predicts risk of relapse in childhood acute lymphoblastic leukemia: a Nordic Society of Pediatric Hematology and Oncology study. Leukemia 2020;34:336-46. [Crossref] [PubMed]
- Malikova H, Liscak R, Latnerova I, et al. Complications of MRI-guided stereotactic biopsy of brain lymphoma. Neuro Endocrinol Lett 2014;35:613-8. [PubMed]
- Iijima K, Hirato M, Miyagishima T, et al. Microrecording and image-guided stereotactic biopsy of deep-seated brain tumors. J Neurosurg 2015;123:978-88. [Crossref] [PubMed]
- Schuurhuis GJ, Heuser M, Freeman S, et al. Minimal/measurable residual disease in AML: a consensus document from the European LeukemiaNet MRD Working Party. Blood 2018;131:1275-91. [Crossref] [PubMed]
- Ostronoff F, Othus M, Lazenby M, et al. Prognostic significance of NPM1 mutations in the absence of FLT3-internal tandem duplication in older patients with acute myeloid leukemia: a SWOG and UK National Cancer Research Institute/Medical Research Council report. J Clin Oncol 2015;33:1157-64. [Crossref] [PubMed]
- Del Principe MI, Buccisano F, Soddu S, et al. Involvement of central nervous system in adult patients with acute myeloid leukemia: Incidence and impact on outcome. Semin Hematol 2018;55:209-14. [Crossref] [PubMed]
- Rozovski U, Ohanian M, Ravandi F, et al. Incidence of and risk factors for involvement of the central nervous system in acute myeloid leukemia. Leuk Lymphoma 2015;56:1392-7. [Crossref] [PubMed]
- Ranta S, Palomäki M, Levinsen M, et al. Presenting features and imaging in childhood acute myeloid leukemia with central nervous system involvement. Pediatr Blood Cancer 2017. [Crossref] [PubMed]
- Ranta S, Palomäki M, Levinsen M, et al. Role of neuroimaging in children with acute lymphoblastic leukemia and central nervous system involvement at diagnosis. Pediatr Blood Cancer 2017;64:64-70. [Crossref] [PubMed]
- Leite da Silveira P, Gonçalves Silva V, Rizzato Paschoal J, et al. Bilateral peripheral facial palsy and mastoid infiltration as symptoms of relapsed acute myeloid leukemia. Eur Ann Otorhinolaryngol Head Neck Dis 2015;132:41-3. [Crossref] [PubMed]
- Grossman SA, Krabak MJ. Leptomeningeal carcinomatosis. Cancer Treat Rev 1999;25:103-19. [Crossref] [PubMed]
- Chamberlain MC, Sandy AD, Press GA. Leptomeningeal metastasis: a comparison of gadolinium-enhanced MR and contrast-enhanced CT of the brain. Neurology 1990;40:435-8. [Crossref] [PubMed]
- Ranta S, Nilsson F, Harila-Saari A, et al. Detection of central nervous system involvement in childhood acute lymphoblastic leukemia by cytomorphology and flow cytometry of the cerebrospinal fluid. Pediatr Blood Cancer 2015;62:951-6. [Crossref] [PubMed]
- Thastrup M, Marquart HV, Levinsen M, et al. Central Nervous System Involvement Detected By Flow Cytometry Is a Risk Factor for Relapse in Childhood Acute Lymphoblastic Leukemia. Blood 2018;132:657. [Crossref]
- Craig FE, Foon KA. Flow cytometric immunophenotyping for hematologic neoplasms. Blood 2008;111:3941-67. [Crossref] [PubMed]
- Sorigué M, Juncà J, Sancho JM, et al. Flow cytometry for detection of central nervous system disease in acute myeloid leukemia. Leuk Lymphoma 2015;56:2190-2. [Crossref] [PubMed]
- Crespo-Solis E, López-Karpovitch X, Higuera J, et al. Diagnosis of acute leukemia in cerebrospinal fluid (CSF-acute leukemia). Curr Oncol Rep 2012;14:369-78. [Crossref] [PubMed]
- Grewal RK, Chetty M, Abayomi EA, et al. Use of flow cytometry in the phenotypic diagnosis of hodgkin's lymphoma. Cytometry B Clin Cytom 2019;96:116-27. [Crossref] [PubMed]
- Tomuleasa C, Selicean C, Cismas S, et al. Minimal residual disease in chronic lymphocytic leukemia: A consensus paper that presents the clinical impact of the presently available laboratory approaches. Crit Rev Clin Lab Sci 2018;55:329-45. [Crossref] [PubMed]
- de Graaf MT, de Jongste AH, Kraan J, et al. Flow cytometric characterization of cerebrospinal fluid cells. Cytometry B Clin Cytom 2011;80:271-81. [Crossref] [PubMed]
- Aune MW, Becker JL, Brugnara C, et al. Automated flow cytometric analysis of blood cells in cerebrospinal fluid: analytic performance. Am J Clin Pathol 2004;121:690-700. [Crossref] [PubMed]
- Kleine TO, Nebe CT, Löwer C, et al. Cell analysis in cerebrospinal fluid (CSF) using Sysmex® hematology analyzers XT-4000i and XE-5000: evaluation with CSF controls of the Joint German Society for Clinical Chemistry and Laboratory Medicine (DGKL). Cytometry A 2012;81:255-64. [Crossref] [PubMed]
- Buccisano F, Maurillo L, Del Principe MI, et al. Prognostic and therapeutic implications of minimal residual disease detection in acute myeloid leukemia. Blood 2012;119:332-41. [Crossref] [PubMed]
- Craig FE, Ohori NP, Gorrill TS, et al. Flow Cytometric Immunophenotyping of Cerebrospinal Fluid Specimens. Am J Clin Pathol 2011;135:22-34. [Crossref] [PubMed]
- Martínez-Cuadrón D, Montesinos P, Pérez-Sirvent M, et al. Central nervous system involvement at first relapse in patients with acute myeloid leukemia. Haematologica 2011;96:1375-9. [Crossref] [PubMed]
- Stewart DJ, Keating MJ, McCredie KB, et al. Natural history of central nervous system acute leukemia in adults. Cancer 1981;47:184-96. [Crossref] [PubMed]
- Pavlovsky S, Eppinger-Helft M, Sackmann Muriel F. Factors that influence the appearance of central nervous system leukemia. Blood 1973;42:935-8. [Crossref] [PubMed]
- Mastrangelo R, Poplack D, Bleyer A, et al. Report and recommendations of the Rome workshop concerning poor-prognosis acute lymphoblastic leukemia in children: biologic bases for staging, stratification, and treatment. Med Pediatr Oncol 1986;14:191-4. [Crossref] [PubMed]
- Sancho JM, Ribera JM, Oriol A, et al. Central nervous system recurrence in adult patients with acute lymphoblastic leukemia: frequency and prognosis in 467 patients without cranial irradiation for prophylaxis. Cancer 2006;106:2540-6. [Crossref] [PubMed]
- Qian L, Tomuleasa C, Florian IA, et al. Advances in the treatment of newly diagnosed primary central nervous system lymphomas. Blood Res 2017;52:159-66. [Crossref] [PubMed]
- Pieters R, Carroll WL. Biology and treatment of acute lymphoblastic leukemia. Pediatr Clin North Am 2008;55:1-20. ix. [Crossref] [PubMed]
- Larson RA. Managing CNS disease in adults with acute lymphoblastic leukemia. Leuk Lymphoma 2018;59:3-13. [Crossref] [PubMed]
- Faderl S, Jeha S, Kantarjian HM. The biology and therapy of adult acute lymphoblastic leukemia. Cancer 2003;98:1337-54. [Crossref] [PubMed]
- Barredo JC, Devidas M, Lauer SJ, et al. Isolated CNS relapse of acute lymphoblastic leukemia treated with intensive systemic chemotherapy and delayed CNS radiation: a pediatric oncology group study. J Clin Oncol 2006;24:3142-9. [Crossref] [PubMed]
- Gunther JR, Rahman AR, Dong W, et al. Craniospinal irradiation prior to stem cell transplant for hematologic malignancies with CNS involvement: Effectiveness and toxicity after photon or proton treatment. Pract Radiat Oncol 2017;7:e401-8. [Crossref] [PubMed]
- Hiniker SM, Agarwal R, Modlin LA, et al. Survival and neurocognitive outcomes after cranial or craniospinal irradiation plus total-body irradiation before stem cell transplantation in pediatric leukemia patients with central nervous system involvement. Int J Radiat Oncol Biol Phys 2014;89:67-74. [Crossref] [PubMed]
- Kumar P, Mulhern RK, Regine WF, et al. A prospective neurocognitive evaluation of children treated with additional chemotherapy and craniospinal irradiation following isolated central nervous system relapse in acute lymphoblastic leukemia. Int J Radiat Oncol Biol Phys 1995;31:561-6. [Crossref] [PubMed]
- Unal S, Yetgin S, Cetin M, et al. The prognosis and survival of childhood acute lymphoblastic leukemia with central nervous system relapse. Pediatr Hematol Oncol 2004;21:279-89. [Crossref] [PubMed]
- Gao RW, Dusenbery KE, Cao Q, et al. Augmenting Total Body Irradiation with a Cranial Boost before Stem Cell Transplantation Protects Against Post-Transplant Central Nervous System Relapse in Acute Lymphoblastic Leukemia. Biol Blood Marrow Transplant 2018;24:501-6. [Crossref] [PubMed]
- Hamidieh AA, Monzavi SM, Kaboutari M, et al. Outcome Analysis of Pediatric Patients with Acute Lymphoblastic Leukemia Treated with Total Body Irradiation-Free Allogeneic Hematopoietic Stem Cell Transplantation: Comparison of Patients with and Without Central Nervous System Involvement. Biol. Blood Marrow Transplant 2017;23:2110-7. [Crossref] [PubMed]
- Famoso JM, Grow JL, Laughlin B, et al. The Impact of Low-Dose Cranial Boost on the Long-Term Outcomes of Adult Patients with High-Risk Acute Lymphoblastic Leukemia Undergoing Total Body Irradiation and Allogeneic Hematopoietic Stem Cell Transplantation. Pract Radiat Oncol 2019;9:e283-9. [Crossref] [PubMed]
- Yeh TC, Liang DC, Hou JY, et al. Treatment of childhood acute lymphoblastic leukemia with delayed first intrathecal therapy and omission of prophylactic cranial irradiation: Results of the TPOG-ALL-2002 study. Cancer 2018;124:4538-47. [Crossref] [PubMed]
- Liu HC, Yeh TC, Hou JY, et al. Triple intrathecal therapy alone with omission of cranial radiation in children with acute lymphoblastic leukemia. J Clin Oncol 2014;32:1825-9. [Crossref] [PubMed]
- Hutchinson L. Paediatric oncology: triple therapy avoids need for radiation in children with ALL. Nat Rev Clin Oncol 2014;11:378. [Crossref] [PubMed]
- Wilejto M, Di Giuseppe G, Hitzler J, et al. Treatment of young children with CNS-positive acute lymphoblastic leukemia without cranial radiotherapy. Pediatr Blood Cancer 2015;62:1881-5. [Crossref] [PubMed]
- Lee EJ, Petroni GR, Schiffer CA, et al. Brief-duration high-intensity chemotherapy for patients with small noncleaved-cell lymphoma or FAB L3 acute lymphocytic leukemia: results of cancer and leukemia group B study 9251. J Clin Oncol 2001;19:4014-22. [Crossref] [PubMed]
- Bostrom BC, Sensel MR, Sather HN, et al. Dexamethasone versus prednisone and daily oral versus weekly intravenous mercaptopurine for patients with standard-risk acute lymphoblastic leukemia: a report from the Children's Cancer Group. Blood 2003;101:3809-17. [Crossref] [PubMed]
- Jones B, Freeman AI, Shuster JJ, et al. Lower incidence of meningeal leukemia when prednisone is replaced by dexamethasone in the treatment of acute lymphocytic leukemia. Med Pediatr Oncol 1991;19:269-75. [Crossref] [PubMed]
- Annino L, Vegna ML, Camera A, et al. Treatment of adult acute lymphoblastic leukemia (ALL): long-term follow-up of the GIMEMA ALL 0288 randomized study. Blood 2002;99:863-71. [Crossref] [PubMed]
- Relling MV, Mahmoud HH, Pui CH, et al. Etoposide achieves potentially cytotoxic concentrations in CSF of children with acute lymphoblastic leukemia. J Clin Oncol 1996;14:399-404. [Crossref] [PubMed]
- Zimm S, Ettinger LJ, Holcenberg JS, et al. Phase I and Clinical Pharmacological Study of Mercaptopurine Administered as a Prolonged Intravenous Infusion. Cancer Res 1985;45:1869-73. [PubMed]
- Riccardi R, Holcenberg JS, Glaubiger DL, et al. L-asparaginase pharmacokinetics and asparagine levels in cerebrospinal fluid of rhesus monkeys and humans. Cancer Res 1981;41:4554-8. [PubMed]
- Colita A, Colita A, Bumbea H, et al. LEAM vs. BEAM vs. CLV Conditioning Regimen for Autologous Stem Cell Transplantation in Malignant Lymphomas. Retrospective Comparison of Toxicity and Efficacy on 222 Patients in the First 100 Days After Transplant, On Behalf of the Romanian Society for Bone Marrow Transplantation. Front Oncol 2019;9:892. [Crossref] [PubMed]
- Gaballa S, Ge I, El Fakih R, et al. Results of a 2-arm, phase 2 clinical trial using post-transplantation cyclophosphamide for the prevention of graft-versus-host disease in haploidentical donor and mismatched unrelated donor hematopoietic stem cell transplantation. Cancer 2016;122:3316-26. [Crossref] [PubMed]
- Tanase A, Tomuleasa C, Marculescu A, et al. Haploidentical Donors: Can Faster Transplantation Be Life-Saving for Patients with Advanced Disease? Acta Haematologica 2016;135:211-6. [Crossref] [PubMed]
- Brammer JE, Khouri I, Gaballa S, et al. Outcomes of Haploidentical Stem Cell Transplantation for Lymphoma with Melphalan-Based Conditioning. Biol Blood Marrow Transplant 2016;22:493-8. [Crossref] [PubMed]