The effects of TGF-β1 on staphylococcus epidermidis biofilm formation in a tree shrew biomaterial-centered infection model
Introduction
The occurrence and development of human diseases can be well simulated in the primate model, although large-scale application of the traditional primate model is limited due to high feeding costs, low fertility, and operational challenges. A variety of small mammal, tree shrews are taxonomically classified as mammalia, placentalia, and scandentia, an independent category between insectivora and primate considered to be an intermediate type linking ancient primates and modern primates. The physiological anatomy, neurodevelopment, viral infection characteristics, and psychological stress mode of tree shrews are highly similar to those of primates. Moreover, tree shrews bear more similarities with humans than commonly-used laboratory animals such as rats and mice, their feeding and reproductive costs are far lower than those of higher primates, such as monkeys, and they are also characterized by the small individual, simple operation and good adaptability to the environment. It is for these reasons that tree shrews have been widely used in medical research.
In recent years, the increase in the application of biomaterials, particularly in patients with malignant tumors, has seen a significant rise in the morbidity rate of biomaterial-centered infection (BCI) (1,2). The initiating factor for BCI is bacterial biofilm adhering to the surface of the material. Of the pathogenic bacteria of biofilm-related infections, Staphylococcus epidermidis is the one most frequently detected. Biofilm formation and immunocompromise in tumor patients are the key difficulties in treating infections (3,4). Therefore, exploring the relationships between the immune function of tumor patients and bacterial colonization and bacterial biofilm formation is of great significance to the clinical prevention and treatment of BCI.
Transforming growth factor-β1 (TGF-β1), a potent immunosuppressor, is highly expressed in a variety of tumors (5). TGF-β1 can inhibit the proliferation and function of lymphocytes and is one of the major reasons behind immune dysfunction in patients with malignant tumors (6).
In this study, the tree shrew model of BCI was established, and the effects of TGF-β1 on cellular immune status of tree shrews and whether it further affects Staphylococcus epidermidis biofilm formation were explored.
We present the following article in accordance with the MDAR reporting checklist (available at http://dx.doi.org/10.21037/atm-20-4526).
Methods
Experimental materials and instruments
The study was performed according to the international, national and institutional rules considering animal experiments, clinical studies and biodiversity rights. The study protocol was approved by the Ethics Committee of Yunnan Cancer Hospital.
Tupaia belangeri of both genders (aged about 6 months old and weighing 140–180 g) purchased from Kunming Institute of Zoology, Chinese Academy of Sciences was used as the animal model and fed in a specific pathogen-free laboratory. ATCC12228 and ATCC35984 standard strains of Staphylococcus epidermidis were sourced from the National Institute for Control of Pharmaceutical and Biological Products. Central venous catheters (CVC, CS-14502, 5F-13CM) were obtained from ARROW. We also purchased FACSCalibur flow cytometer from Becton Dikinson, and a scanning electron microscope (S-100) from HITACHI.
Experimental methods
The effects of TGF-β1 at different concentrations on the levels of T helper (Th)1 and Th2 cytokines in tree shrews
Forty tree shrews were divided at random into four groups: S1 group (n=10, control group, no intravenous injection of TGF-β1), S2 group (n=10, 100 ng/kg TGF-β1), S3 group (n=10, 200 ng/kg TGF-β1), and S4 group (n=10, 400 ng/kg TGF-β1). Anesthesia was administered via intramuscular injection of 5% ketamine hydrochloride (10 mg/kg), before the tail hair was shaved off, and TGF-β1 at different concentrations was injected through the caudal vein.
The effects of TGF-β1 on the levels of Th1 and Th2 cytokines in tree shrews
After a period of 48 h, 1 mL of venous blood was drawn from the caudal vein of the tree shrews, and flow cytometry was employed to detect the levels of Th1 cytokines interleukin-2 (IL-2), tumor necrosis factor (TNF), and interferon-γ (IFN-γ), and Th2 cytokines IL-6, IL-4, and IL-10. The 488 nm spectral line of an argon ion laser provided the source for the excitation light. The specific surface markers of T cells were used, and allogeneic IgG-stained cells served as negative controls in each fluorescence channel. The results were determined based on the standard curves in each group.
The placement of the CVC in the tree shrews
Following anesthesia via an intramuscular injection of ketamine, the femoral vein of the tree shrews was punctured, and a CVC was inserted under aseptic conditions. Eighty tree shrews were divided at random into four groups: S1 group (n=20), S2 group (n=20), S3 group (n=20), and S4 group (n=20). After the weighing the tree shrews, TGF-β1 at different concentrations was injected via CVC.
Preparation of bacterial suspension
The preserved ATCC12228 (PIA−) (PIA,Polysaccharide Intercellular Adhesion) and ATCC35984 (PIA+) standard strains of Staphylococcus epidermidis were inoculated into the agar plate medium for 24 h at 37 °C. Then, a single colony was inoculated into LB medium (Luria Broth) for 16 h at 37 °C until the logarithmic growth phase, and the bacterial concentration was adjusted to 1×107 CFU/mL. Ten tree shrews in the S1, S2, S3, and S4 groups were injected with 1 mL of freshly prepared bacteria solution of ATCC12228 standard strains of Staphylococcus epidermidis, while the remaining 10 tree shrews in each group were injected with 1 mL of freshly prepared bacteria solution of ATCC35984 standard strains of Staphylococcus epidermidis. About 2 cm was cut off the catheter and placed into the femoral vein, and the incision was sutured with sterile silk thread.
Biofilm detection
At 72 h following intubation, the CVC in the femoral vein was removed and divided into 3 sections. The effects of different levels of TGF-β1 on biofilm formation in the tree shrew BCI model in were observed in the experimental group and the control group: (I) bacteria were identified using the API bacterial identification system; (II) bacterial biofilm formation in each group was detected via semi-quantitative biofilm formation assay; and (III) the surface state of biofilm was observed using the scanning electron microscope.
Bacterial identification using the API (Analytic Products INC) bacterial identification system
The CVC was immersed in bouillon culture medium, and the biofilm was ultrasonically eluted. After doubling dilution, the bouillon was implanted into the blood plate. After the bacterial colony had formed, the API bacterial identification system was used to establish whether the bacteria cultured were Staphylococcus epidermidis.
Detection of bacterial biofilm formation in each group via semi-quantitative biofilm formation assay
The CVC biofilm was dissolved in TSB (Tryptic Soy Broth) medium, diluted at 1:100, and inoculated into a sterile 96-well plate, with the clean fresh bouillon without bacteria solution added as a blank control. After culturing at 37 °C for 24 h, the biofilm was stained with crystal violet, a microplate reader was used to measure the absorbance at a wavelength of 490 nm (A490 nm), and the average was taken for each strain of bacteria and blank control. The biofilm formation was positive if mean A490 nm of bacterial strain to be detected—mean A490 nm of blank control >0.12.
The observation of surface state of biofilm using a scanning electron microscope
The CVC was removed, and immediately fixed with 3% glutaraldehyde and stored at 4 °C. A scanning electron microscope was used to observe bacterial growth on the internal surface of catheter.
Statistical methods
The experimental data were statistically analyzed using SPSS 11.5 software package. Kolmogorov-Smirnov normality test was performed for each group before data analysis. The experimental data conforming to the normal distribution or meeting the normal distribution after conversion were expressed as mean ± standard deviation (
Results
The effects of TGF-β1 at different concentrations (100, 200, and 400 ng/kg) on Th1 and Th2 cytokines in tree shrews
In TGF-β1 groups at different concentrations (100, 200, and 400 ng/kg), the levels of Th1 cytokines IL-2, TNF, and IFN-γ were lower than those of the normal group (F=18.124, P<0.05). According to further pairwise q tests among the groups, there was no significant difference in the levels between the 100 ng group and the 200 ng group (P>0.05), and the levels were lower in the 400 ng group than in 100 and 200 ng groups (P<0.01). In addition, the levels of Th2 cytokines IL-6, IL-4, and IL-10 in the TGF-β1 groups at different concentrations were higher than those in normal group (F=15.421, P<0.05). According to further pairwise q tests among the groups, there were no significant differences in the levels between the 100 ng group and the 200 ng group (P>0.05), and the levels were higher in 400 ng group than in the 100 ng group and the 200 ng group (P<0.01) (Table 1).
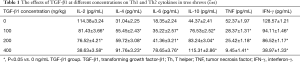
Full table
The effects of TGF-β1 at different concentrations (100, 200, and 400 ng/kg) on PIA+ Staphylococcus epidermidis biofilm formation in the BCI tree shrew model
- The PIA+ Staphylococcus epidermidis BCI model was established using the PIA+ ATCC35984 standard strains, and the CVC in the femoral vein was removed after 72 h and detected by semi-quantitative biofilm formation assay (Table 2). According to rank sum test, the positive rate of biofilm formation was higher in the TGF-β1 groups at different concentrations than in the non-TGF-β1 group (P<0.01). The results of further pairwise comparison showed that there were no significant differences in the positive rate of biofilm formation among the 100, 200, and 400 ng TGF-β1 groups (t=3.26, P>0.05).
- Based on the biofilm observed on the internal surface of the CVCs using the scanning electron microscope, there was obvious biofilm formation of Staphylococcus epidermidis in the 200 and 400 ng TGF-β1 groups, and its formation ability was higher than in the 100 ng TGF-β1 and control groups (Figure 1).
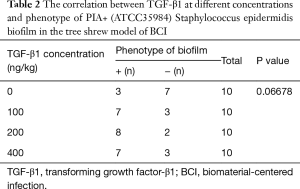
Full table

The effects of TGF-β1 at different concentrations (100, 200, and 400 ng/kg) on PIA− Staphylococcus epidermidis biofilm formation in the tree shrew BCI model
- The PIA− Staphylococcus epidermidis BCI model was established using the PIA− ATCC12228 standard strains, and the CVC in the femoral vein was removed after 72 h and detected through semi-quantitative biofilm formation assay (Table 3). According to rank sum test, there were no significant differences in the positive rate of biofilm formation between the TGF-β1 groups at different concentrations (100, 200, and 400 ng/kg) and the non-TGF-β1 group (P>0.05). The results of further pairwise comparison revealed that the positive rate of biofilm formation was not significantly different among the 100, 200, and 400 ng TGF-β1 groups (t=3.26, P>0.05).
- Based on the biofilm observed on the internal surface of the CVCs using the scanning electron microscope, there were no significant differences in the Staphylococcus epidermidis biofilm formation in 100, 200, and 400 ng TGF-β1 groups compared with control group (Figure 2).
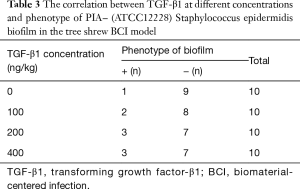
Full table

Discussion
At present, there are around 47 species and more than 100 subspecies of tree shrews in the world. Due to constraints on resources, there are only a small number of feeding and breeding centers for tree shrews around the globe, including Germany Primate Center (Gottingen), the University of Alabama at Birmingham (USA), San Diego Wild Animal Park (USA), Mexico Wolff Breeding Center, and Europe Primate Center (Netherlands); far fewer than the number of feeding and breeding centers for primates. In most literature reports, tree shrews in Germany Primate Center were used. In China, there are now 1 species (Tupaia belangeri) and 6 subspecies of tree shrews, and wild tree shrews are mostly distributed in Yunnan (3 out of 6 subspecies). The domestication and breeding of tree shrews and related research in China dates back to the 1970−80s.
Tree shrews are small mammals that resemble squirrels, which are taxonomically classified as mammalia, placentalia, and scandentia. They are mainly distributed in Bangkok (Thailand), Nepal, Myanmar, and Yunnan and Guangxi (China). Due to their special taxonomic status, tree shrews have attracted the attention of biologists. For a long time, tree shrews have been regarded as primates, and many studies have shown them to have close kinship with primates, although their specific phylogenetic taxonomic status is still debated.
Tree shrews have many similarities with primates and even humans in terms of their physiological anatomy, neurodevelopment, viral infection characteristics, and psychological stress mode. The prefrontal lobe is highly developed in humans, developed in tree shrews and primates, and almost not developed in rats and mice. The brain/weight ratio of tree shrews exceeds that of humans. Studies have demonstrated that (7) tree shrews and humans have similar cranial nerves and optic nerves, and they are also the only species other than chimpanzees and gibbons that can be infected with human hepatitis A, hepatitis B, hepatitis C, rotavirus, herpes virus, adenovirus, and rhabdovirus.
TGF-β1 and cellular immune status in tree shrews
TGF-β1 is a kind of polypeptidase growth inhibitor with various biological functions (8), which has a molecular weight of 25 KD. It has the signal transduction pathway, inhibits the proliferation and activity of T cells and macrophages, and regulates the expression of various target genes, serving as a negative regulator of embryo growth and development, cell differentiation and proliferation, as well as a pro-apoptotic factor. Basic research has also shown that (9) TGF-β1 is the most potent of the TGF superfamily members in the human body (10). TGF-β1 can inhibit the expression of protooncogene C-myc at the post-transcriptional level, arrest the cell cycle in the G1 phase and inhibit tumor formation. Abnormalities in TGF-β1 can lead to the blockage of signal transduction pathways, enhance the expression of C-myc, and relieve the dephosphorylation of Rb and P53 protein, which sees the cells grow excessively, resulting in malignant transformation (11).
TGF-β1 has been proved to be a potent immunosuppressive factor in immunoregulation (12) which can induce donor-specific immune tolerance through multiple pathways. TGF-β1 can also exert an immunoregulatory effect in tree shrews (13). In this experiment, TGF-β1 at different concentrations (100, 200, and 400 ng/kg) was injected into the femoral vein of tree shrews, and venous blood was drawn after 48 h to detect cytokines via flow cytometry. The levels of Th1 cytokines IL-2, TNF, and IFN-γ were found to be lower in the TGF-β1 groups at different concentrations than in the normal group (F=18.124, P<0.05). According to further pairwise q tests among groups, there were no significant differences in the levels between the 100 ng group and the 200 ng group (P>0.05), and the levels were lower in the 400 ng group than in the 100 and 200 ng groups (P<0.01). Furthermore, the levels of Th2 cytokines IL-6, IL-4, and IL-10 in the TGF-β1 groups at different concentrations were higher than in the normal group (F =15.421, P<0.05). According to further pairwise q tests among the groups, there were no significant differences in the levels between the 100 ng group and the 200 ng group (P>0.05), and the levels in the 400 ng group were higher than in the 100 and 200 ng groups (P<0.01). The above findings indicate that the increased concentration of TGF-β1 in tree shrews can inhibit the levels of Th1 cytokines, and promote the level of Th2 cytokine IL-6. Moreover, TGF-β1 causes the imbalance of Th1/Th2 cytokines and Th1/Th2 shift in tree shrews, leading to the Th1 cell-led decline in cellular immune function.
The correlation between TGF-β1 and CVC-centered Staphylococcus epidermidis biofilm formation in tree shrews
The cellular immunity in the body is mediated by T lymphocytes, and their main function is to regulate all immune responses caused by protein antigens (14) as well as to scavenge cell surface antigens or intracellular microorganisms, to play a leading role in resisting bacterial infection in cells. When T cells come into contact with some pathogens or vaccines (15), they differentiate and proliferate into sensitized or immune T cells, mainly Th lymphocytes (CD4+/CD3+) and cytotoxic T lymphocytes (CD8+/CD3+). Cytokines are secreted by Th lymphocytes to respond to the antigenic stimulus, which can facilitate the proliferation and differentiation of T lymphocytes, B lymphocytes, and macrophages.
At the infection site, foreign antigens enter the body and are captured by dendritic cells and gathered in the lymph nodes, where they are responsible for the activation of juvenile lymphocytes, and lymphocytes migrate to the lymph nodes via the blood vessels. Then, effector and memory T cells are produced in the lymph nodes and enter into the blood circulation so that T cells can migrate to the surrounding tissues. Antibodies originating in the lymphoid organs then enter the blood circulation, finding and binding to specific antigens at any site (16).
Many studies have found that (17-19) the adhesion of bacteria to the surface of biomaterials and adjacent tissues is the initiating factor of BCI. The emergence of biomaterials reduces the minimum amount of bacteria required for pathogenesis, biofilm production increases bacterial resistance to antibiotics by 10–1,000 times compared with airborne bacteria, and the subsequent infection often cannot be controlled until the implant is removed.
Bacterial biofilm refers to the membrane-like multi-bacterial complex comprising bacterial cells and aqueous polymeric matrix (mainly exopolysaccharides) secreted by them, which adheres to the body surface during bacterial growth (20). During evolution, a fine adhesion mechanism is gradually formed in most bacteria, which secrete the matrix and form the membranous substances adhering to the surface of lesion, thereby producing the complex group of biofilms (21).
Staphylococcus epidermidis is a kind of Gram-positive cocci (22) widely distributed in nature, most of which are opportunistic pathogens. Some people may carry pathogenic strains in their skin and nasopharynx, and the carrier rate can reach more than 70% in medical staff. Staphylococcus epidermidis is an important source of cross infection in hospitals and is an important opportunistic pathogen in nosocomial infection (23). In addition, Staphylococcus epidermidis adheres to the mucosa or the surface of biomedical materials, such as cannula and artificial joints, which secrete polysaccharide complexes such as polysaccharide matrix, fibrin and lipopolysaccharide, and then they adhere to each other and clone to become biofilms (24).
In this experiment, the Staphylococcus epidermidis BCI model of tree shrews was established using the PIA+ ATCC35984 and PIA− ATCC12228 standard strains. The CVC in the femoral vein was removed after 72 h and detected via semi-quantitative biofilm formation assay. The positive rate of ATCC35984 biofilm formation was found to be higher in the TGF-β1 groups at different concentrations (100, 200, and 400 ng/kg) than in the non-TGF-β1 group (P<0.01). The results of further pairwise comparison showed that there were no significant differences in the positive rate of biofilm formation among the 100, 200, and 400 ng TGF-β1 groups (P>0.05). According to the biofilm observed on the CVC using the scanning electron microscope, the ATCC35984 biofilm formation ability in the 200 and 400 ng TGF-β1 groups was higher than in the 100 ng TGF-β1 and control groups, which demonstrates that TGF-β1 promotes PIA+ Staphylococcus epidermidis biofilm formation in the tree shrew BCI model, although it has no significant influence on PIA− Staphylococcus epidermidis biofilm formation on the surface of CVC.
A biofilm is a well differentiated group formed by coordination among bacteria, which is one of the important means for bacteria to resist external adverse factors (25). The bacteria in a biofilm are different from single planktonic bacteria, and they can effectively resist the body's defense mechanism and antibiotic treatment, placing them as the root cause of uncontrollable biomaterial infection. Therefore, studying whether the interference in cellular immunity affects biofilm formation during bacterial adhesion, exploring the internal mechanism of biofilm formation from the perspective of genetic manipulation, establishing the initiating and terminating factors during adhesion, and searching for the methods to block bacterial adhesion and resist bacterial biofilm formation will help with the prevention and treatment of BCI in tumor therapy. This will in turn create the conditions for further advancements in tumor treatments, which is of great significance in improving the quality of life and prolonging the lifespan of patients.
Acknowledgments
Funding: This work was supported by National Natural Science Foundation of China (81960335, 82060426); Natural Science Foundation of Yunnan Province (2017FA039); Natural Science Foundation of Yunnan Province (2017FE468-214, 2017FE468-159, 2017FE467-098); Medical Experts Training Project of Yunnan Province (D-201641, H-2018025).
Footnote
Reporting Checklist: The authors have completed the MDAR reporting checklist. Available at http://dx.doi.org/10.21037/atm-20-4526
Data Sharing Statement: Available at http://dx.doi.org/10.21037/atm-20-4526
Conflicts of Interest: All authors have completed the ICMJE uniform disclosure form (available at http://dx.doi.org/10.21037/atm-20-4526). The authors have no conflicts of interest to declare.
Ethical Statement: The authors are accountable for all aspects of the work in ensuring that questions related to the accuracy or integrity of any part of the work are appropriately investigated and resolved. The study was performed according to the international, national and institutional rules considering animal experiments, clinical studies and biodiversity rights. The study protocol was approved by the Ethics Committee of Yunnan Cancer Hospital.
Open Access Statement: This is an Open Access article distributed in accordance with the Creative Commons Attribution-NonCommercial-NoDerivs 4.0 International License (CC BY-NC-ND 4.0), which permits the non-commercial replication and distribution of the article with the strict proviso that no changes or edits are made and the original work is properly cited (including links to both the formal publication through the relevant DOI and the license). See: https://creativecommons.org/licenses/by-nc-nd/4.0/.
References
- Huang YC, Yang KY, Lei YJ, et al. Relationship between bacterial adhesion to prosthetic valve materials and bacterial growth. Zhongguo Zuzhi Gongcheng Yanjiu yu Linchuang Kangfu 2008;12:7777-80.
- Tucci G, Romanini E, Zanoli G, et al. Prevention of surgical site infections in orthopaedic surgery: a synthesis of current recommendations. Eur Rev Med Pharmacol Sci 2019;23:224-39. [PubMed]
- Chen Y, Wang XY, Huang YC, et al. Study on the Structure of Candida Albicans-Staphylococcus Epidermidis Mixed Species Biofilm on Polyvinyl Chloride Biomaterial. Cell Biochem Biophys 2015;73:461-468. [Crossref] [PubMed]
- Ye L, Xu G, Huang Y, et al. Brominated furanone of Cardiothoracic Surgery and polyvinyl chloride in surface of Staphylococcus aureus biofilm formation. Shengwuyixue Gongcheng yu Linchuang 2010;14:203-6.
- Li MJ, Wang X, Chen Y, et al. The influences of TGF-beta1 upon the human adenocarcinoma cell of lung A549 and cellular immunity. Ann Transl Med 2020;8:1076. [Crossref] [PubMed]
- Xiang YJ, Ren M, Jiang H, et al. Ex vivo expansion of antigen-specific CD4+CD25+ regulatory T cells from autologous naïve CD4+ T cells of multiple sclerosis patients as a potential therapeutic approach. Eur Rev Med Pharmacol Sci 2016;20:5261-70. [PubMed]
- Chen WT, Liu TM, Wu SH, et al. Improving diagnosis of central venous catheter-related bloodstream infection by using differential time to positivity as a hospital-wide approach at a cancer hospital. J Infect 2009;59:317-23. [Crossref] [PubMed]
- Kassar R, Hachem R, Jiang Y, et al. Management of Bacillus bacteremia: the need for catheter removal. Medicine (Baltimore) 2009;88:279-83. [Crossref] [PubMed]
- Chee L, Brown M, Sasadeusz J, et al. Gram-negative organism spredominate in Hickman line-related infections in non-neutropenic patients with hematological malignancies. J Infect 2008;56:227-33. [Crossref] [PubMed]
- Suzuki K, Ohishi K, Ino K, et al. Marked hyponatremia withconsciousness disturbance probably caused by linezolid in a patient with acutemyeloid leukemia. Kansenshogaku Zasshi 2008;82:38-42. [Crossref] [PubMed]
- Loh AH, Chui CH. Port-A-Cath insertions in acute leukaemia and childhood malignancies. Asian J Surg 2007;30:193-9. [Crossref] [PubMed]
- Console G, Calabro C, Nardulli P, et al. Clinical and economic effects of central venous catheters on oncology patient care. J Chemother 2007;19:309-14. [Crossref] [PubMed]
- Shukrallah B, Hanna H, Hachem R, et al. Correlation between early clinical response after catheter removal and diagnosis of catheter-related bloodstream infection. Diagn Microbiol Infect Dis 2007;58:453-7. [Crossref] [PubMed]
- García-Teresa MA, Casado-Flores J, Delgado Domínguez MA, et al. Infectious complications of percutaneous central venous catheterization in pediatric patients: a Spanish multicenter study. Intensive Care Med 2007;33:466-76. [Crossref] [PubMed]
- McConnell SA, Gubbins PO, Anaissie EJ. Do antimicrobial-impregnated centralvenous catheters prevent catheter-related bloodstream infection? Clin Infect Dis 2003;37:65-72. [Crossref] [PubMed]
- Seifert H, Cornely O, Seggewiss K, et al. Bloodstream infection in neutropenic cancer patients related to short-term non tunnelled catheters determined by quantitative blood cultures, differential time to positivity, and molecular epidemiological typing with pulsed-field gel electrophoresis. J Clin Microbiol 2003;41:118-23. [Crossref] [PubMed]
- Hachem R, Raad I. Prevention and management of long-term catheter related infections in cancer patients. Cancer Invest 2002;20:1105-13. [Crossref] [PubMed]
- Raad II, Hanna HA. Intravascular catheter-related infections: new horizons and recent advances. Arch Intern Med 2002;162:871-8. [Crossref] [PubMed]
- Rothberg MB, Lindenauer PK, Lahti M, et al. Risk factor model to predict venous thromboembolism in hospitalized medical patients. J Hosp Med 2011;6:202-9. [Crossref] [PubMed]
- Li MJ, Wang X, Chen Y, et al. The influences of TGF-β1 upon the human adenocarcinoma cell of lung A549 and cellular immunity. Ann Transl Med 2020;8:1076. [Crossref] [PubMed]
- Tao C, Zhang J, Yang S. Preparation and biocompatibility of BSA monolayer on silicon surface. J Nanosci Nanotechnol 2011;11:5068-74. [Crossref] [PubMed]
- Solouk A, Mirzadeh H, Shokrgozar MA, et al. The study of collagen immobilization on a novel nanocomposite to enhance cell adhesion and growth. Iran Biomed J 2011;15:6-14. [PubMed]
- Wang R, Mikoryak C, Li S, et al. Cytotoxicity screening of single-walled carbon nanotubes: detection and removal of cytotoxic contaminants from carboxylated carbon nanotubes. Mol Pharm 2011;8:1351-61. [Crossref] [PubMed]
- Lim J, Cui Y, Oh YJ, et al. Studying the effect of alginate overproduction on Pseudomonas aeruginosa biofilm by atomic force microscopy. J Nanosci Nanotechnol 2011;11:5676-81. [Crossref] [PubMed]
- Waines PL, Moate R, Moody AJ, et al. The effect of material choice on biofilm formation in a model warm water distribution system. Biofouling 2011;27:1161-74. [Crossref] [PubMed]