A graphical guide for constructing a finite element model of the cervical spine with digital orthopedic software
Introduction
Due to its high incidence and complexity, cervical spondylosis poses a constant challenge to the operation of spinal surgery (1,2). Sophisticated knowledge of the anatomy, biomechanics, and pathological mechanisms have been considered the requisite factors for exploring the therapeutic strategies in cervical spondylosis treatment (3). In particular, understanding the biomechanics of spinal motion and internal fixation is critical for spinal surgeons (4), surgical training, and for the testing of innovative instruments. Usually, innovative instruments or methods will be tested on cadaveric specimens, which are costly and have limited availability (5,6). In addition, the anatomy and function of animal specimens differ greatly from those of humans, and thus the data derived from animal specimens are not applicable to human characteristics in most cases (7,8).
Finite element analysis (FEA) can simulate different clinical situations in a realistic manner and complement ordinary experimental approaches in spinal research (9,10). Thus, finite element (FE) models have been increasingly applied to assist in the design and development of spine implants and the assessment of spine biomechanics with instrumentations of interest. The results of digital biomechanics studies can further clarify the mechanisms behind results obtained by other evaluations of implant behavior and spinal motion segments (5,8,9). Furthermore, FEA models allow us to explore a broad range of physiological conditions and to assess their combined impact on implant systems (10).
A variety of digital software has been used to study the cervical spine (11-14). In 1993, Kleinberger (11) used Patran and LS-DYNA three-dimensional (3D) software to establish the earliest 3D FE models of the cervical spine, including the vertebrae, intervertebral discs, and main ligaments, in order to investigate the mechanism of cervical spine injury sustained during automobile collisions. Since then, the FE model of the cervical spine has significantly improved from a 3D FE model (12) to models capable of simulating complex motion conditions (13) and studying bones and muscles (14). The continuous development of the cervical spine FE method has resulted in it being increasingly used for more complex mechanical analysis, such as cervical spine injury, artificial disc replacement, interbody fusion internal fixation, cervical spondylosis, and cervical spine instability (15-19).
However, the original reconstruction of FEA must be pre-handled by software like Mimics and Geomagic studio (10). Despite the provision of tutorials for these programs, many functions cannot be utilized for orthopedic study, especially for spine-related investigation. In addition, there are few step-by-step protocols available to guide orthopedic researchers through establishing a digital spine FEA model, while no routine consensus exists regarding the ideal way to build digital models through Mimics and Geomagic software for FEA. This weakens the reliability of experimental data and the dissemination of the results.
We have thus outlined a step-by-step protocol with which researchers can easily and reliably establish a digital model of the cervical spine. We hope this represents a valuable be a step towards revealing the internal mechanisms of the behaviors of implants and related spinal motion segments.
Materials and methods
We used computed tomography (CT) images (DICOM format, version 2.0 or higher), Mimics software (version 19.0 or higher; Materialise, Leuven, Belgium), Geomagic studio software (version 2012 or higher, 3D Systems, USA), and ANSYS software (version 19.0 or higher; Ansys, USA).
A step-by-step guide for 3D reconstruction in Mimics follows below:
- Obtain and archive consecutive CT images in DICOM format in the local drive. Importantly, all images must be stored in only one directory.
- Run the Mimics software and access the saved files. Select File and New Project Wizard and choose the DICOM directory. Then, click Next to select a study in the list and click Convert. After confirming that the orientations are correct, click OK to proceed (Figure 1).
- Click Segment followed by CT Bone Segmentation, and then choose a bone (here we chose C4) with the + button. Carefully select Seed Threshold and Sensitivity to obtain a full bone profile. Each bone should be segmented individually using the same method as above. Click Next to readjust the threshold and ensure the profiles are filled. Choose Next again and set the Gap Closing Distance to 2–3 px; then select Fill Long Bones and Apply Setting to All Masks if applicable. Finally, click Calculate to obtain masks of the bones of interest (Figure 2).
- Click Segment followed by Morphology Operations to operate the morphology of the mask. Click Open to open a source mask and remove small thorns and click Close to fill small cavities. After confirming that there are no errors in the mask, click Segment and Calculate 3D from Mask (Figure 3A). In Figure 3B, for example, the 3D model of C3 is calculated by selecting the mask of C4 and then choosing Optimal Quality.
- Click the Save button to save the project; this will prompt a dialog box to appear. Click File, Export, and Binary STL. Click 3D, choose a model, and click Add in the Object to Convert Field. Ensure the Output Directory is properly set before clicking Finish to export the 3D model in STL format (Figure 4).
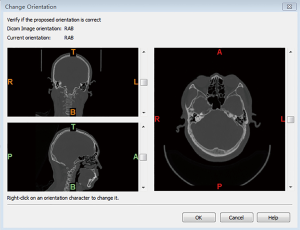
A step-by-step guide for model processing in Geomagic follows below:
- Run the Geomagic software. Click File and Import to open the C4 STL. As shown in Figure 5, the C4 STL file will be automatically imported in Polygons mode, and Mesh Doctor will be also automatically activated. Next, click the Apply button to remove Highly Creased Edges and Spikes. Various types of imperfections can be removed at this step.
- If uneven or spiked surfaces remain, right-click to delete them (Figure 6). To fill any hole caused by deleting a surface, click Polygons and Fill Single.
- Click Exact Surfaces and Exact Surfacing to enter the exact surfacing mode (Figure 7A,B). After this, make sure to click Detect Contours to divide the surface model into several parts. The surfaces can be separated not only by curvature but also by functional area. For clarity, click Compute to measure the curvatures of different regions. Then, manually select each separate region according to its functional areas. Subsequently, check these regions carefully and click Extract to extract the boundaries from them. When a dialog appears (as shown in Figure 7C,D) click OK to edit the boundaries at a later point. Users can manually add or delete the contours of the regions by selecting Edit Contours (left-click or Ctrl+ left-click).
- After editting the contours, click OK and Construct Patches to construct patches of the model. If an error is reported, select Specify Patch Count with a particular number for Target Patch Count and click Apply until the error disappears. The Repair Patches and Shuffle tools can also be used to repair or rearrange the patches (Figure 8) if the surface has no patch.
- Click Construct Grids, input a number into Resolution, and click Apply to inspect the grids and confirm that there are no errors. Click OK to continue (Figure 9).
- Set the parameters of the step as Fit Surfaces, (as shown in Figure 10). Click Apply to apply these parameters and inspect the model. The outline of the model should not be present in the important patches. Click OK to begin the next step.
- Save the project on your local drive, and then right-click the model name and click Save to output the model as a STP or IGS file (Figure 11).
A step-by-step guide for model processing in Ansys follows below:
- Run the Ansys Workbench software. Double-click Static Structural to operate static structural analysis of the C4 model, and click Engineering Data to add bone or other required materials (Figure 12).
- Enter Design Modeler by double-clicking Geometry, and then import the C4 file by clicking File and Import External Geometry File (Figure 13A). If more than one model is necessary, users can click Boolean to import extra models and the Body Transformation operation to assemble them (Figure 13B).
- Close Design Modeler and double-click Model and select Mechanical. The material property should be determined first. Then, select a model by right-clicking Materials, Insert, and Material Assignment (C4 in the example), and choose the material type (cortical bone is shown in Figure 14A). Then, to trace the model with mesh, right-click Mesh and apply Generate Mesh in the Default Settings (Figure 14B).
- The loading and boundary conditions can be inserted as needed by right-clicking Analysis Settings followed by Insert and the desired conditions (as depicted in Figure 15). In the example, we used force and fixed support to simulate head weight on the upper surface of the C4.
- Click Solution, Insert, Stress, Equivalent (von-Mises) to insert an equivalent stress result and other results including strain and deformation. Finally, click Solution and Solve to solve the FE model, which may take several minutes (Figure 16).
- After completion of the above steps, the FE model with elements, nodes, and boundary conditions will be created (Figure 17).
Discussion
Herein, we have presented the stepwise instructions for how to use (I) Mimics software to reconstruct an original model of a cervical spine segment based on a series of CT images, (II) Geomagic studio to refine the original model, and (III) preliminary treatment to generate a detailed model for FEA with ANSYS. Throughout the entire process, key interfaces were captured and are presented here. After a FEA model of C4 was successfully constructed, a simple simulation was applied to make it more comprehensible.
Even though FEA has been widely used in simulation studies of cervical spine injury, degeneration, and surgical treatment, current cervical spine FE research still has several limitations First, the structures of neck muscles, nerves, and blood vessels are neglected by this approach. Second, the majority of studies have simplified the connection structure between the vertebrae, resulting in differences between the calculated results and true values. Third, the material parameters used in the current simulation are not consistent with the real situation, as large-scale simplification occurs, significantly reducing the accuracy of research results. Fourth, FE technology lends itself to mathematical and theoretical research and is less accessible to spinal surgeons who are more interested in the clinical implications of cervical spine disease. Therefore, to help those with less experience understand this technology, we have created clear and detailed instructions for establishing a cervical FE model.
Mimics is a highly integrated and easy-to-use image generation and editing software. It is extremely capable of establishing 3D models from CT or magnetic resonance imaging (MRI) data, with integrated editing, analysis, and result output functions. The model can be saved in computer-aided design (CAD), FEA, and rapid prototyping (RP) formats, which is highly amenable to large-scale data conversion and processing on a personal computer (20). It also facilitates the use of CT or MR images for the purpose of either clinical practice or research. For example, it is commonly used to make pre-operative plans for spinal surgery. More specifically, a comprehensive and stereoscopic understanding of the pathology of a specific case can allow researchers to create more detailed plans and simulations for the procedure by translating the stitched images into a 3D model (21). Knowing the exact pathology of a patient through using a model can help avoid the potential failures that would occur from referring to an unrealistic anatomical representation (22,23).
A 3D model is invaluable for providing critical information necessary to understanding the complexity of the pathology, and thus saves time and labor in comparison to interpreting 2D images (22). In addition, precisely reconstructed 3D models can be used for anatomical measurement. Mimics software has been used by Li et al. to measure the spinal canal volume and neural foramen dimension of pedicle-lengthening osteotomy (24), by Feng et al. (25) to measure cervical endplate surfaces, and by Ma et al. (26) to simulate bone resections and measure anatomy parameters during total knee arthroplasty. Mimics use in relation to the feasibility and radiological features of sacral alar fixation has also been reported (27).
Geomagic studio software can automatically generate accurate digital models based on any physical component by scanning the spot cloud (28). As automated reverse engineering software, it also provides an ideal option for recently developed applications, such as in the design of new prostheses, on-demand production patterns, and automatic reconstruction of human physiological structure. It also perfectly complements CAD software, CA education, and CA manufacturing tools, the models of which can be output into standard industry formats, including STL, IGES, STEP, and CAD (29). Geomagic software acts as a firm bridge between Mimics and FEA by constructing the characteristic boundary constraint conditions and providing reference conditions for curved surface slices. This results in the best effect across the whole surface through modification (30).
The applications of Geomagic software in the medical field include the design of prosthesis implants, assistance in surgery, and the analysis of human biomechanical features. Zhao et al. applied Geomagic to establish a model for FEA to investigate the biomechanical properties of a novel modular intercalary prosthesis for humeral diaphyseal segmental defect reconstruction (31). Wu et al. measured the biomechanics of a novel anterior trans-pedicular screw artificial vertebral body system for lower cervical spine fixation (30) with Geomagic software being applied to acquire non-uniform rational b-splines surfaces for FEA to simulate facet joints and intervertebral discs.
With advances in digital techniques and computer science, the FEA method can simulate a variety of clinical situations in a more realistic way by acting in a fully complementary partnership with the experimental approaches of cervical spine biomechanics research (32). FEA models of bones and joints are created using geometrical and material parameters obtained from imaging modalities. The results obtained from FEA analysis allow the exploration of an essentially limitless range of physiological and pathological conditions, which will facilitate a greater understanding of system biomechanics (24,25,33,34).
FEA analysis also has utility in soft-tissue research. For instance, Polak et al. (35) reported an FE micro-scale model of the denticulate ligaments to investigate tensile characterization. To understand the biomechanical features of multilevel hybrid constructs of the cervical spine, Li et al. (36) used FEA and analyzed several different fixation models. Similar work was performed by Yuan et al. (37), in which an FE model of the whole cervical spine was used to study artificial disc replacement. Based on this biomechanical data, they confirmed that the replacement of different heights was a significant factor in prosthesis. Other researchers (30,38) have also evaluated the novel integrated artificial axis before preclinical fixation by FEA. Zheng et al. (38) demonstrated remarkable biomechanical application by applying a 3D FE model to detect the biomechanical features of a newly designed fixation system from the occiput to the fourth cervical vertebra. An FE model used by Wu et al. (30) efficiently compared the biomechanical properties of a novel anterior trans-pedicular screw vertebral body system (AVBS) and a conventional anterior screw plate system (ASPS). Their results proved that AVBS possesses better stability and a lower risk for internal fixator failure then ASPS fixation. The stiffness of bone material adjacent to the implant has also been examined by several researchers (39,40). In a study of the biomechanics of traditional Chinese cervical manipulation, Deng et al. (41) established an FE model of the cervical spine and demonstrated that cervical manipulation cannot reduce intradiscal pressure.
Continuous improvements in algorithms and computing power will, in the future, allow surgeons to use FE simulation to quickly determine alternate operation plans, saving time and optimizing surgical outcomes. In our view, the combination of FE analysis and artificial intelligence algorithms will greatly improve the application of spine and cervical surgery. We present this literature review detailing the integration of the Mimics, Geomagic, and FEA software to assist those working in these fields.
There are several limitations to this study. First, various methods and procedures are available for building a 3D model with Mimics reconstruction for FEA. We offer a simplified instructional guide for users, and most methodologically compatible procedures can be customer-modified according to the user’s needs. Second, a full understanding of Ansys and other software requires mathematical and mechanical knowledge, the lack of which may bring difficulty in operating these programs. Moreover, we have only provided an introduction to the basic methodology, and some steps and extra extensions have not been thoroughly explained. Users can learn such knowledge by searching for corresponding domains and consulting each program’s help sections.
To summarize, we have presented clear and useful step-by-step instructions for combining Mimics, Geomagics, and FEA for spinal biomechanics analysis. We advocate this protocol for its simplicity, reliability, and convenience in obtaining FE biomechanics simulations.
Acknowledgments
Funding: This work was supported by the Classroom Instructional Reform Research Topic of Wenzhou Medical University (No. YBKG201843).
Footnote
Conflicts of Interest: All authors have completed the ICMJE uniform disclosure form (available at http://dx.doi.org/10.21037/atm-20-2451). The authors have no conflicts of interest to declare.
Ethical Statement: The authors are accountable for all aspects of the work in ensuring that questions related to the accuracy or integrity of any part of the work are appropriately investigated and resolved.
Open Access Statement: This is an Open Access article distributed in accordance with the Creative Commons Attribution-NonCommercial-NoDerivs 4.0 International License (CC BY-NC-ND 4.0), which permits the non-commercial replication and distribution of the article with the strict proviso that no changes or edits are made and the original work is properly cited (including links to both the formal publication through the relevant DOI and the license). See: https://creativecommons.org/licenses/by-nc-nd/4.0/.
References
- Chang CC, Huang WC, Wu JC, et al. The Option of Motion Preservation in Cervical Spondylosis: Cervical Disc Arthroplasty Update. Neurospine 2018;15:296-305. [Crossref] [PubMed]
- Grasso G. Role of hybrid construct in cervical spondylosis. Spine J 2018;18:2164-5. [Crossref] [PubMed]
- Tan LA, Riew KD, Traynelis VC. Cervical Spine Deformity-Part 1: Biomechanics, Radiographic Parameters, and Classification. Neurosurgery 2017;81:197-203. [Crossref] [PubMed]
- Zhang QH, Teo EC. Finite element application in implant research for treatment of lumbar degenerative disc disease. Med Eng Phys 2008;30:1246-56. [Crossref] [PubMed]
- Schmidt H, Galbusera F, Rohlmann A, et al. What have we learned from finite element model studies of lumbar intervertebral discs in the past four decades? J Biomech 2013;46:2342-55. [Crossref] [PubMed]
- Halou H, Chalkias A, Mystrioti D, et al. Evaluation of the willingness for cadaveric donation in Greece: a population-based study. Anat Sci Educ 2013;6:48-55. [Crossref] [PubMed]
- Bertocci GE, Brown NP, Embleton NA, et al. Canine Stifle Biomechanics Associated With a Novel Extracapsular Articulating Implant Predicted Using a Computer Model. Vet Surg 2016;45:327-35. [Crossref] [PubMed]
- Bertocci GE, Brown NP, Mich PM. Biomechanics of an orthosis-managed cranial cruciate ligament-deficient canine stifle joint predicted by use of a computer model. Am J Vet Res 2017;78:27-35. [Crossref] [PubMed]
- Zhang Z, Fogel GR, Liao Z, et al. Biomechanical Analysis of Lateral Lumbar Interbody Fusion Constructs with Various Fixation Options: Based on a Validated Finite Element Model. World Neurosurg 2018;114:e1120-9. [Crossref] [PubMed]
- Zhang Z, Fogel GR, Liao Z, et al. Biomechanical evaluation of four surgical scenarios of lumbar fusion with hyperlordotic interbody cage: A finite element study. Biomed Mater Eng 2018;29:485-97. [Crossref] [PubMed]
- Kleinberger M. Application of Finite Element Techniques to the Study of Cervical Spine Mechanics. Element Eng 1993;48:SAE Technical Paper 933131.
- Bozic KJ, Keyak JH, Skinner HB, et al. Three-Dimensional Finite Element Modeling of a Cervical Vertebra. J Spinal Disord 1994;7:102-10. [Crossref] [PubMed]
- Teo EC, Ng HW. Evaluation of the role of ligaments, facets and disc nucleus in lower cervical spine under compression and sagittal moments using finite element method. Med Eng Phys 2001;23:155-64. [Crossref] [PubMed]
- Hedenstierna S, Halldin P, Siegmund GP. Neck muscle load distribution in lateral, frontal, and rear-end impacts: a three-dimensional finite element analysis. Spine (Phila Pa 1976) 2009;34:2626-33. [Crossref] [PubMed]
- Zhang JG, Wang F, Zhou R, et al. A three-dimensional finite element model of the cervical spine: an investigation of whiplash injury. Med Biol Eng Comput 2011;49:193-201. [Crossref] [PubMed]
- Kang H, Park P, La Marca F, et al. Analysis of load sharing on uncovertebral and facet joints at the C5-6 level with implantation of the Bryan, Prestige LP, or ProDisc-C cervical disc prosthesis: an in vivo image-based finite element study. Neurosurg Focus 2010;28:E9. [Crossref] [PubMed]
- Fernandes PC, Fernandes PR, Folgado JO, et al. Biomechanical analysis of the anterior cervical fusion. Comput Methods Biomech Biomed Engin 2012;15:1337-46. [Crossref] [PubMed]
- Kumaresan S, Yoganandan N, Pintar FA, et al. Contribution of disc degeneration to osteophyte formation in the cervical spine: a biomechanical investigation. J Orthop Res 2001;19:977-84. [Crossref] [PubMed]
- Zhang H, Bai J. Development and validation of a finite element model of the occipito-atlantoaxial complex under physiologic loads. Spine (Phila Pa 1976) 2007;32:968-74. [Crossref] [PubMed]
- An G, Hong L, Zhou XB, et al. Accuracy and efficiency of computer-aided anatomical analysis using 3D visualization software based on semi-automated and automated segmentations. Ann Anat 2017;210:76-83. [Crossref] [PubMed]
- Shin DS, Lee S, Park HS, et al. Segmentation and surface reconstruction of a cadaver heart on Mimics software. Folia Morphol (Warsz) 2015;74:372. [Crossref] [PubMed]
- Kim Y. Prediction of mechanical behaviors at interfaces between bone and two interbody cages of lumbar spine segments. Spine (Phila Pa 1976) 2001;26:1437-42. [Crossref] [PubMed]
- Chiang MF, Zhong ZC, Chen CS, et al. Biomechanical comparison of instrumented posterior lumbar interbody fusion with one or two cages by finite element analysis. Spine (Phila Pa 1976) 2006;31:E682-9. [Crossref] [PubMed]
- Li P, Qian L, Wu WD, et al. Impact of pedicle-lengthening osteotomy on spinal canal volume and neural foramen size in three types of lumbar spinal stenosis. Bone Joint Res 2016;5:239-46. [Crossref] [PubMed]
- Feng H, Li H, Ba Z, et al. Morphometry evaluations of cervical osseous endplates based on three dimensional reconstructions. Int Orthop 2019;43:1521-8. [Crossref] [PubMed]
- Ma QL, Lipman JD, Cheng CK, et al. A Comparison Between Chinese and Caucasian 3-Dimensional Bony Morphometry in Presimulated and Postsimulated Osteotomy for Total Knee Arthroplasty. J Arthroplasty 2017;32:2878-86. [Crossref] [PubMed]
- Wu AM, Chi YL, Ni WF, et al. The feasibility and radiological features of sacral alar iliac fixation in an adult population: a 3D imaging study. PeerJ 2016;4:e1587. [Crossref] [PubMed]
- Zeller S, Guichet D, Kontogiorgos E, et al. Accuracy of three digital workflows for implant abutment and crown fabrication using a digital measuring technique. J Prosthet Dent 2019;121:276-84. [Crossref] [PubMed]
- Vecsei B, Joós-Kovács G, Borbély J, et al. Comparison of the accuracy of direct and indirect three-dimensional digitizing processes for CAD/CAM systems – An in vitro study. J Prosthodont Res 2017;61:177-84. [Crossref] [PubMed]
- Wu W, Chen C, Ning J, et al. A Novel Anterior Transpedicular Screw Artificial Vertebral Body System for Lower Cervical Spine Fixation: A Finite Element Study. J Biomech Eng 2017;139:061003. [Crossref] [PubMed]
- Zhao LM, Tian DM, Wei Y, et al. Biomechanical Analysis of a Novel Intercalary Prosthesis for Humeral Diaphyseal Segmental Defect Reconstruction. Orthop Surg 2018;10:23-31. [Crossref] [PubMed]
- Gilbertson LG, Goel VK, Kong WZ, et al. Finite element methods in spine biomechanics research. Crit Rev Biomed Eng 1995;23:411-73. [Crossref] [PubMed]
- Acar B, Kose O, Kati YA, et al. Comparison of volar versus dorsal screw fixation for scaphoid waist fractures: A finite element analysis. Orthop Traumatol Surg Res 2018;104:1107-13. [Crossref] [PubMed]
- Seyed Vosoughi A, Joukar A, Kiapour A, et al. Optimal satellite rod constructs to mitigate rod failure following pedicle subtraction osteotomy (PSO): a finite element study. Spine J 2019;19:931-41. [Crossref] [PubMed]
- Polak-Kraśna K, Robak-Nawrocka S, Szotek S, et al. The denticulate ligament - Tensile characterisation and finite element micro-scale model of the structure stabilising spinal cord. J Mech Behav Biomed Mater 2019;91:10-7. [Crossref] [PubMed]
- Li Y, Zhu J, Liao Z, et al. Hybrid Constructs for Performing Three-level Hybrid Surgery: A Finite Element Study. World Neurosurg 2018;114:e1302-9. [Crossref] [PubMed]
- Yuan W, Zhang H, Zhou X, et al. The Influence of Artificial Cervical Disc Prosthesis Height on the Cervical Biomechanics: A Finite Element Study. World Neurosurg 2018;113:e490-8. [Crossref] [PubMed]
- Zheng Y, Wang J, Liao S, et al. Biomechanical evaluation of a novel integrated artificial axis: A finite element study. Medicine (Baltimore) 2017;96:e8597. [Crossref] [PubMed]
- Liu S, Qi W, Zhang Y, et al. Effect of bone material properties on effective region in screw-bone model: an experimental and finite element study. Biomed Eng Online 2014;13:83. [Crossref] [PubMed]
- Folgado J, Fernandes PR, Jacobs CR, et al. Influence of femoral stem geometry, material and extent of porous coating on bone ingrowth and atrophy in cementless total hip arthroplasty: an iterative finite element model. Comput Methods Biomech Biomed Engin 2009;12:135-45. [Crossref] [PubMed]
- Deng Z, Wang K, Wang H, et al. A finite element study of traditional Chinese cervical manipulation. Eur Spine J 2017;26:2308-17. [Crossref] [PubMed]
(English Language Editor: B. Draper; Quality Control Editor: J. Gray)