A comparison of the inflammatory response following autologous compared with allogenic islet cell transplantation
Introduction
Allogenic islet cell transplantation is predominantly used to treat insulin dependent Type 1 diabetes mellitus, whereas autologous islet transplantation is employed following total pancreatectomy for unremitting pain in chronic pancreatitis. Both procedures are broadly similar conceptually but have key differences related to the islet procurement, islet isolation procedures and their immunobiology (1).
Allogenic islet transplantation is now established as a treatment for insulin-dependent diabetes mellitus and the continued development of anti-rejection therapies together with refinements in the islet isolation process have facilitated a progressive improvement in clinical outcomes (1-7). These improvements include islet isolation protocols, rates of insulin independence and prevention of hypoglycaemic unawareness (8). In addition, although the function of solid organ allografts declines over time, islet transplants generally continue to remain functional as evidenced by the continued production of C-peptide (9,10).
Between 1999 and 2002 islet transplant protocols were predominately based on the Edmonton Protocol which utilised an interleukin-2 receptor antagonist, an mTOR inhibitor and a calcineurin inhibitor (CNI) (11). After 2002 there was a shift towards the use of induction therapy with T-cell depleting agents and the judicious addition of tumour necrosis factor-α (TNF-α) inhibitor and these developments were introduced into clinical practice over the next decade (12,13). An mTOR inhibitor or an inosine monophosphate dehydrogenase inhibitor (IMPDH) combined with a CNI are now commonly used as maintenance therapy in allogenic islet transplantation (14).
Autologous islet cell transplantation is generally performed following a total pancreatectomy for the treatment of intractable pain in patients with chronic pancreatitis provided that they are not diabetic. Insulin independence following autologous islet transplantation is quite variable and although it declines over time falling from 46% at 5 years to 10% by 8 years (15). This is significantly higher than respective figures for allogenic transplantation. It is likely that differences in immunogenicity between allogenic and autologous infusions of islets may account for the observed differences in insulin independence. Both forms of islet cell transplantation stimulate an immediate innate immune response but autologous islets do not undergo ‘rejection’ or demonstrate susceptibility to the harmful effects of autoimmunity and consequently do not require immunosuppressive therapy. Another important difference is that maintenance immunosuppression following allogenic transplantation generally requires the use of diabetogenic agents which further reduces the prospect of insulin independence in the long term (16).
An important area of islet transplantation research concerns the initial phase following transplantation when the infusion of the islet preparation may precipitate an acute inflammatory response with the activation of complement. This inflammatory response has the potential to significantly damage the infused islets, inhibiting their implantation and resulting in a reduced effective islet cell mass (17-24). Indeed, it has been suggested that as many as 50–60% of infused allogenic islet cells may be lost in the immediate post-transplant period. This significant initial loss of islet cell mass is one of the contributing factors to the almost ubiquitous requirement for multiple donor pancreata for allogenic transplantation to achieve an adequate islet cell mass and consequent medium and long-term insulin independence.
There are numerous reports contrasting the inflammatory response following autologous and allogenic islet cell transplantation, but these are generally performed after the immediate post-transplant period and to our knowledge there are no studies examining the initial inflammatory phase during and/or immediately following islet cell infusion. The purpose of this study was to compare the serum cytokine profiles following allogenic and autologous islet cell transplantation in the early post-operative phase and to examine them in relation to the mode of induction therapy. We present the following article in accordance with the MDAR reporting checklist (available at http://dx.doi.org/10.21037/atm-20-3519).
Methods
Patients
Serum samples from patients who had received an islet transplant were obtained from three international centres; autologous islets following total pancreatectomy and digestion of the pancreas from the Leicester General Hospital (LGH; Leicester, United Kingdom), the Baylor Research Institute (BRI; Dallas, United States) and allogenic islets from the BRI and the Royal Adelaide Hospital (RAH; Adelaide, Australia and New Zealand Clinical Trials Registry No 083020). Two control patients were also recruited who had undergone total pancreatectomy without islet transplantation. The study was conducted in accordance with the Declaration of Helsinki (as revised in 2013). The study was approved by the institutional review board of University Hospitals of Leicester NHS Trust (EDGE-34223), and written informed consent was obtained from all patients included in the study.
Autologous islet protocol
In the Leicester series, islets were prepared and infused as previous described (25). The resected pancreas was digested with the neutral protease NB GMP Grade in combination with purified Collagenase NB 1 GMP Grade (SERVA Electrophoresis GmbH, Heidelberg, Germany). Unpurified whole pancreatic digest was suspended in a 20% human serum albumin and M199 transplant media. Islets were prepared while the surgeons completed the surgical reconstruction (26). Immediately prior to the islet infusion the patients received 5000 iU heparin intravenously. Islets were infused into the portal vein over 20–30 minutes via a recannalised umbilical vein and during the islet infusion the portal pressure were continuously monitored to ensure that the it remained below 20 mmHg (27).
In the BRI patients islets were prepared and infused again as previous described (28). Pancreata were digested with either Liberase MTF with Thermolysin (Roche, Indianapolis - United States) or Collagenase NB 1 GMP Grade (SERVA Electrophoresis GmbH, Heidelberg, Germany). Isolated islets were infused into the portal vein via a mesenteric vein with added heparin (70 iU/kg body weight) over 30 to 60 minutes while the patients were under general anaesthesia. During islet infusion, portal vein pressure was monitored intermittently. If the portal vein pressure exceeded 20 mmHg, the infusion of islets was discontinued until portal vein pressure decreased.
Allogenic islet transplant
In the BRI series islets were prepared and evaluated as previously described (29). Briefly, pancreatic ductal injection was performed with the ET-Kyoto solution and the oxygen-charged static two-layer method (29,30). The pancreas was infused with collagenase NBI and neutral protease (SERVA Electrophoresis, Germany). Islets were purified by means of a continuous density gradient in a COBE 2991 cell processor with Ficoll solution, and were assessed using dithizone staining to determine the yield and purity. The islet yield was then converted into a standard number of islet equivalents. Islet viability was measured with fluorescein diacetate and propidium iodide staining.
Rabbit anti-thymocyte globulin (ATG, Thymoglobulin, Genzyme, MA) was administered intravenously at a dose of 1.5 mg/kg on days 0, 2, 4 and 6 post-transplant for the induction of immunosuppression. Tacrolimus (Prograf, Astellas Inc) and mycophenolate mofetil (MMF, CellCept, Roche Laboratories, NJ) (2 g/day orally) were used to maintain immunosuppression. Anakinra (Kineret, Amgen, CA) (100 mg) was administered intravenously an hour pre-transplant and subcutaneously for 7 days following transplantation. In addition, etanercept (Enbrel, Immunex, WA) (50 mg) was administered intravenously an hour pre-transplant and subcutaneously on days 3, 6 and 10 post-transplantation.
In the series from Adelaide (Australia and New Zealand Clinical Trials Registry) islets were separated at two islet isolation facilities as described previously using a variation of the closed loop method described by Ricordi et al. (31,32). Donor pancreata were removed from heart-beating deceased donors and ductal infusion was performed with cold collagenase NB1 GMP grade (SERVA, Heidelberg, Germany) or Liberase HI GMP grade (Roche Indianapolis, IN). Dissociated islet and acinar tissue were separated on a continuous Biocoll (Biochrom AG, Berlin) density gradient (polysucrose 400 and amidotrizoic acid) on a refrigerated apheresis system (Model 2991, COBE Laboratories, Lakewood, Colorado).
All patients received tacrolimus (Astellas Inc.), mycophenolate mofetil (Roche) and rabbit anti-thymocyte globulin (Fresenius). ATG was administered intravenously at a dose of 3 mg/kg for 5 days at the time of transplant. Tacrolimus (Prograf, Astellas Inc.) was commenced at a dose of 0.1 mg/kg per day in two equal divided doses, with the dose adjusted to achieve a target blood concentration of 10 ng/mL. MMF was commenced at a dose of 1gm bd.
Serum tests
Serum samples were obtained during induction of the anaesthesia, at 15 and 30 minutes prior to islet infusion, at the end of the operation, and subsequently at 1, 3, 6, 24 hours and 2, 3, 5, and 7 days post islet infusion. Serum samples were stored at –80 °C until assayed centrally in Leicester.
Secretion of cytokines and chemokines was determined by measuring serum samples in a Luminex 200 (Millipore, Billerca, Massachussetts, USA) using xMAP technology. The bead assay was performed according to the manufacturer’s instructions with a sensitivity of 1 pg/ml/cytokine. Markers were interferon alpha (IFN-α), interferon gamma (IFN-γ), interleukin-10 (IL-10), interleukin-12p40 (IL-12p40), interleukin-12p70 (IL-12p70), interleukin-13 (IL-13), interleukin-15 (IL-15), interleukin-17 (IL-17), interleukin-1ra (IL-1ra), interleukin-1 alpha (IL-1α), interleukin-1 beta (IL-1β), interleukin-2 (IL-2), interleukin-3 (IL-3), interleukin-4 (IL-4), interleukin-5 (IL-5), interleukin-6 (IL-6), interleukin-7 (IL-7), interleukin-8 (IL-8), Epidermal growth factor (EGF), Eotaxin, Granulocyte colony-stimulating factor (G-CSF), granulocyte-macrophage colony-stimulating factor (GM-CSF), vascular endothelial growth factor (VEGF), Monocyte Chemotactic Protein-1 (MCP-1), macrophage inflammatory protein 1 alpha (MIP-1α), macrophage inflammatory protein 1 beta (MIP-1β), tumour necrosis factors-alpha (TNF-α) and tumour necrosis factors-beta (TNF-β). The Wieslab® Complement system Screen (Euro-Diagnostica, Malmö, Sweden) was used according to the manufacturer’s instructions.
Statistical analysis
All data were initially entered into an Excel database (Microsoft, Redmond, Washington, USA) and the analysis was performed using the Statistical Package for the Social Sciences Windows, version 13.0 (SPSS, Chicago, Illinois, USA). Descriptive statistics consisted of the mean and standard deviation for parametric distributions and median and range for non-parametric distributions after confirmation with the Kolmogorov-Smirnov test and histograms.
Comparisons over time were performed with the Friedman test for non-parametric variables. Comparison among the patient cohorts were performed using Mann-Whitney test for non-parametric variables. A “P” value of less than 0.05 was considered statistically significant.
Results
Patients
Eleven autologous and six allogenic islet cell transplant patients participated in the study from 2010-2012 and the demographics are shown in Tables 1,2. Pre-transplant haemoglobin A1c (HbA1c) levels were significantly higher in the islet allogenic cohort than in the autologous group (P=0.05) (Table 3). Transplanted islet cell suspension volumes were significantly lower in the allogenic islet cell transplant group than in the autologous islet cell transplantation group (P<0.05) (Table 3). Islet purity was significantly higher in the allogenic than in the autologous group (P<0.05) (Table 3).
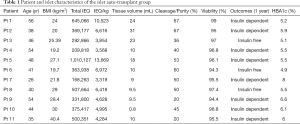
Full table
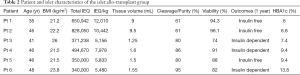
Full table
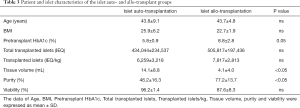
Full table
The cytokine changes that occurred in patients who underwent total pancreatectomy followed by autologous islet transplantation were not significantly different from the changes observed in the control group of patients who only underwent a total pancreatectomy. The Initial increase in cytokine levels occurred during induction of anaesthesia and during the surgical resection (Figure 1A).
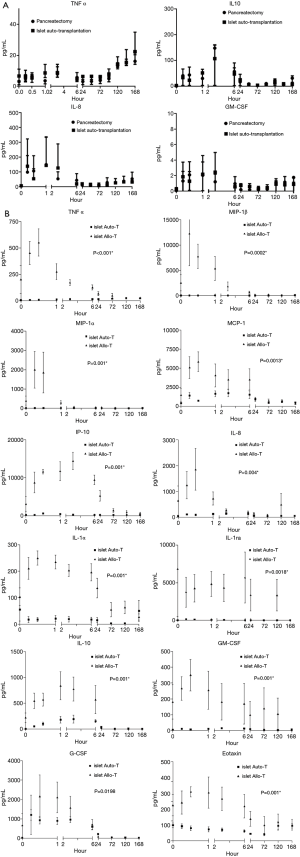
Cytokines changes
Significantly elevated cytokine concentrations following allogenic transplantation which compared with recipients of autologous transplants included; TNF-α (P<0.001), MIP-1α (P=0.001), IP-10 (P=0.001), MIP-1β (P=0.0002), MCP-1 (P=0.013), IL-8 (P=0.004), IL-1α (P=0.001), IL-10 (P=0.001), G-CSF (P=0.0198), IL-1ra (P=0018), GM-CSF (P=0.001) and Eotaxin (P=0.001) (Figure 1B). IL-6 concentrations were initially higher in the allogenic group compared with the autologous group, but after the first hour post infusion this finding was reversed with higher concentrations in the autologous group (P<0.0001). In autologous recipients EGF concentrations at completion of the infusion, 15 minutes following infusion and on the first postoperative day were all significant lower compared with pre-infusion levels.
Cytokines differences in allogenic versus autologous transplantation group over time (Table 4)
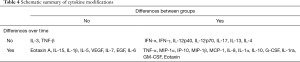
Full table
Lower initial concentrations of the following cytokines were noted in the patients receiving autologous transplants compared with patients who received allografts; IFN-α (P<0.001), IFN-γ (P<0.001) IL-12p40 (P<0.0001), IL-12p70 (P<0.0001) and IL-17 (P<0.0001), but these differences were not sustained. Higher concentrations of IL-13 (P<0.0001) and IL-4 (P<0.001) were observed following autologous compared with allogenic islet cell transplantation. Eotaxin A concentrations were increased significantly compared with pre-infusion levels (P<0.0001) until the 6th postoperative day. After day 6 levels returned to those found preoperatively. IL-15 concentrations on the first and third postoperative days were significantly higher than on admission (P<0.05). IL-1β concentrations were higher compared to baseline levels from the third to seventh postoperative day in the allogenic transplant group and findings were similar for IL-5 and VEGF concentrations in the autologous group and for concentrations of IL-7 concentrations in both groups.
Complement activity
Mannose-binding lectin (MBL) functional pathway deficiency was found in four patients following autologous islet cell infusion and one patient following an allogenic transplant. Conversely classic pathway (CP) activity was reduced compared with baseline levels from the completion of the islet infusion to the 3rd postoperative day, when it recovered to preoperative levels (Figure 2A). These changes were also seen in both the MBL and alternative pathway (AP) activities in both groups (Figure 2B,C). C3 and C4 complement components reduced in the acute phase and were similar in both groups of patients (Figure 2D,E).
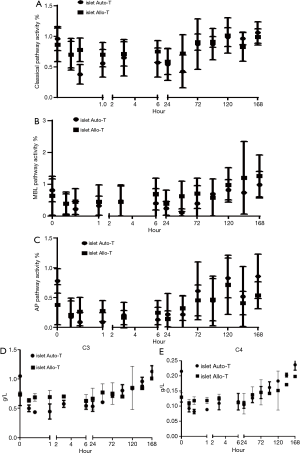
Correlations with insulin-free or insulin-dependent outcome
Eight patients were insulin dependent and three patients insulin free following autologous islet cell transplantation compared with three patients who were insulin dependent and three patients were insulin free following allogenic transplantation. There were no statistically significant differences in ∆VEGF and ∆IL-10 levels between the two groups. However, ∆VEGF levels were higher in the insulin free group when compared with the insulin dependent group in allogenic patients (227.7±228.6 pg.mL−1vs. 30±41.5 pg.mL−1). ∆IL-10 levels were six-fold higher in the insulin-free group compared with in the insulin-dependent group in the allogenic patients (1,389.3±1,080.6 vs. 213.3±178.2 pg.mL−1) (Table 5 and Figure 3A,B).
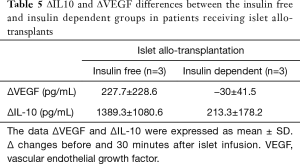
Full table
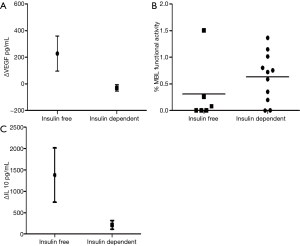
Although MBL functional activity in patients that were insulin free was half that of the insulin dependent group (0.31±0.59 vs. 0.63±0.45) this was not statistically significant (Figure 3C).
Differences in cytokine profiles across institutions
A comparison of the serum cytokine responses between allogenic islet recipients treated with two different induction regimens (Figure 4) was performed to examine the influence of these drugs in the early inflammatory phase. In the two patients from the USA the regimen included interleukin-1 receptor antagonism (IL1ra; Anakinra) and anti-TNF-α (Etanercept) in combination with T cell depletion with anti-thymocyte globulin. This contrasted with our Australian patient cohort who were treated with anti-thymocyte globulin alone. Both groups received maintenance with CNI and tacrolimus. The Australian cohort had elevated levels of IL-8, TNF-α, MCP-1 and Eotaxin compared with the IL1ra/TNF-α treated patients (Figure 5).
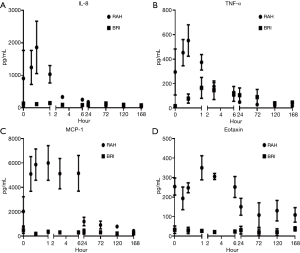
Discussion
The long-term outcome of islet transplantation is critically dependent on the number of viable islets that survive the immediate post-transplant period, implant and continue to function in the long-term. Many factors contribute to poor islet viability including pre-transplant factors, the toxicity of different immunosuppressive regimens (33-36) and activation of the coagulation cascade (37).
The comparison of autologous islet transplantation with allogenic islet transplantation provides a unique insight into the systemic innate immune response in the absence of human leukocyte antigen (HLA) mismatches and immunosuppression. We postulate that the improved functional advantage of autografts is due to the absence of rejection conferred by autoimmunity. Examining the systemic inflammatory response to human islet tissue in vivo may identify factors that could be targets for therapeutic peri-transplant immunosuppressive regimens (7,38).
Islet cell transplantation, whether allografts or autotransplants, initiates a generalised inflammatory response, manifesting as elevated biochemical markers, raised leukocyte counts and elevated acute phase proteins. Inflammatory mediators including cytokines, chemokines, and complement activation products may well contribute to the early loss of islets (7).
This aim of this study was to investigate the release of those cytokines, chemokines and activated complement components that occurs immediately following islet cell infusion. The different inflammatory patterns and the degree of systemic involvement in both forms of islet cell transplantation were varied and supported the hypothesis that the early phase following transplantation represents a crucial period for the infused islets. In patients receiving an allograft, markedly raised levels of EGF, GM-CSF, IL-1ra, IL-1α, IL-2, IL-8, IL-10, MIP-1β and TNF-α are found which contrasts sharply with the findings in the autologous islet cell recipients. The primary cytokines released after islet cell transplantation play an important role during the acute-phase response (35). Several studies have shown that combinations of cytokines such as IL-1β, IFN-γ and TNF-α lead to islet cell death or dysfunction (39). In our study, TNF-α is significantly elevated in patients following allogenic islet cell infusion compared with those receiving an autologous transplant. In patients receiving the Baylor protocol, which utilises TNF-α inhibitors, it has been shown that TNF-α levels are significant mediators of processes involved in islet cell dysfunction or death (40). The Baylor group has also demonstrated that a dual combination regimen of IL-1β and TNF-α inhibition reduced islet damage resulting from non-specific inflammation (41,42).
IL-8, classified as a CXC-chemokine (the two N-terminal cysteines of CXC chemokines are separated by one amino acid, represented by “X”), induces chemotaxis and the activation of T cells and granulocytes. The persistent elevation of these chemokines during islet cell transplantation may potentially reflect the involvement of granulocytes causing early loss of cell mass (43). In contrast to the rise of several inflammatory cytokines and changes in the anti-inflammatory cytokine IL-10 were modest, further supporting a net inflammatory response during islet transplantation. IL-10 may however play an important role in the immune regulation and in our study IL-10 levels were significantly raised in allogenic islet cell transplant patients compared with those receiving an autologous transplant (44). Furthermore, IL-10 levels were found to be six-fold higher in patients who became insulin independent compared with those who were insulin dependent. This is in agreement with Huurman et al. who investigated the role of IL-10 in preventing the onset of diabetes in non-diabetic mice following islet cell allo-transplantation (45,46).
Long-term insulin independence following islet transplantation depends on an adequate islet cell mass (islet equivalents) and early and complete revascularization of islet cell clusters to facilitate engraftment and subsequent survival and function. Delayed or incomplete revascularization may cause early islet loss and graft failure and previous studies have reported that levels of VEGF, as a surrogate for revascularisation, were markedly reduced in the first few days post-operatively and that it may take two weeks for transplanted islets to undergo complete and stable neo-vascularization (47,48). In our study, we demonstrated a similar pattern with VEGF levels being reduced for 72 hours following islet transplantation, but increasing again after day 4. Interestingly, we also found that VEGF produced two peaks in the insulin free cohort following allogenic transplantation with the first peak observed 30 minutes after transplantation.
Complement is another important component of the response to islet transplantation and a determinant of the success or failure of engraftment particularly in the initial phase. It is part of the instant blood-mediated inflammatory reaction (IBMIR) which occurs at the time of intra-portal infusion and involves the coagulation and complement cascades. Complement activation is well described in reperfusion injury during organ transplantation and we have previously studied complement activation and deficiency in islet transplantation (49-51).
It is generally believed that complement activation is a short, transient innate immune response (49) and although our study has confirmed that complement activation does occur immediately following surgery we have also shown that activation can continue for 72 hours. Complement activation increases C3a and C5a which initiates triggers of the pro-inflammatory response and is a likely candidate involved in islet cell loss in the postoperative period (52). MBL functional activity was however low in the insulin-free cohorts in both groups and these preliminary results suggest that deficiency of the MBL pathway may result in a better clinical outcome following islet cell transplantation. This is supported by our previous studies where we found that MBL deficiency or reduced activity improved the outcome in autologous transplantation patients (51).
This study also provides novel data regarding the cytokine profile of islet allograft recipients treated with two different induction regimes. Although the patient numbers were small, there were marked differences between the induction regimes with in vivo human data demonstrating a marked reduction in a range of inflammatory mediators. Studies from the Collaborative Islet Transplant Registry (CITR) suggest that addition of T cell depletion and anti-TNF-α significantly improve the rate of insulin independence following islet transplantation. The present data provides further supporting evidence for the reduction of inflammation by the addition of agents producing IL1ra and TNF-α antagonism.
Conclusions
Allogenic islet cell transplantation produces a significantly raised inflammatory profile in comparison with autologous islet cell transplantation in the initial post-infusion phase. There remains a need to fully understand all the contributory components and to develop strategies which will abrogate the response and maximise islet cell engraftment, survival and long-term function.
Acknowledgments
We thank the patients and staff of the hospitals in the UK, USA and Australia for contributing patients to this analysis.
Funding: This work was partially supported by a Diabetes UK Small Grant (BAD 11/0004278; 26/09/2011).
Footnote
Reporting Checklist: The authors have completed the MDAR reporting checklist. Available at http://dx.doi.org/10.21037/atm-20-3519
Data Sharing Statement: Available at http://dx.doi.org/10.21037/atm-20-3519
Peer Review File: Available at http://dx.doi.org/10.21037/atm-20-3519
Conflicts of Interest: All authors have completed the ICMJE uniform disclosure form (available at http://dx.doi.org/10.21037/atm-20-3519). The authors have no conflicts of interest to declare.
Ethical Statement: The authors are accountable for all aspects of the work in ensuring that questions related to the accuracy or integrity of any part of the work are appropriately investigated and resolved. The study was conducted in accordance with the Declaration of Helsinki (as revised in 2013). The study was approved by the institutional review board of University Hospitals of Leicester NHS Trust (EDGE-34223), and written informed consent was obtained from all patients included in the study.
Open Access Statement: This is an Open Access article distributed in accordance with the Creative Commons Attribution-NonCommercial-NoDerivs 4.0 International License (CC BY-NC-ND 4.0), which permits the non-commercial replication and distribution of the article with the strict proviso that no changes or edits are made and the original work is properly cited (including links to both the formal publication through the relevant DOI and the license). See: https://creativecommons.org/licenses/by-nc-nd/4.0/.
References
- Robertson RP. Islet transplantation for type 1 diabetes, 2015: what have we learned from alloislet and autoislet successes? Diabetes Care 2015;38:1030-5. [Crossref] [PubMed]
- Shapiro AM, Lakey JR, Ryan EA, et al. Islet transplantation in seven patients with type 1 diabetes mellitus using a glucocorticoid-free immunosuppressive regimen. N Engl J Med 2000;343:230-8. [Crossref] [PubMed]
- Shapiro AM, Lakey JR. Future trends in islet cell transplantation. Diabetes Technol Ther 2000;2:449-52. [Crossref] [PubMed]
- Hilbrands R, Huurman VA, Gillard P, et al. Differences in baseline lymphocyte counts and autoreactivity are associated with differences in outcome of islet cell transplantation in type 1 diabetic patients. Diabetes 2009;58:2267-76. [Crossref] [PubMed]
- Froud T, Ricordi C, Baidal DA, et al. Islet transplantation in type 1 diabetes mellitus using cultured islets and steroid-free immunosuppression: Miami experience. Am J Transplant 2005;5:2037-46. [Crossref] [PubMed]
- Matsumoto S. Islet cell transplantation for Type 1 diabetes. J Diabetes 2010;2:16-22. [Crossref] [PubMed]
- McCall M, Shapiro AM. Update on islet transplantation. Cold Spring Harb Perspect Med 2012;2:a007823. [Crossref] [PubMed]
- Chang CA, Lawrence MC, Naziruddin B. Current issues in allogeneic islet transplantation. Curr Opin Organ Transplant 2017;22:437-43. [Crossref] [PubMed]
- Lablanche S, Vantyghem MC, Kessler L, et al. Islet transplantation versus insulin therapy in patients with type 1 diabetes with severe hypoglycaemia or poorly controlled glycaemia after kidney transplantation (TRIMECO): a multicentre, randomised controlled trial. Lancet Diabetes Endocrinol 2018;6:527-37. [Crossref] [PubMed]
- McCall M, Shapiro AM. Islet cell transplantation. Semin Pediatr Surg 2014;23:83-90. [Crossref] [PubMed]
- Ryan EA, Lakey JR, Rajotte RV, et al. Clinical outcomes and insulin secretion after islet transplantation with the Edmonton protocol. Diabetes 2001;50:710-9. [Crossref] [PubMed]
- Takita M, Matsumoto S, Shimoda M, et al. Safety and tolerability of the T-cell depletion protocol coupled with anakinra and etanercept for clinical islet cell transplantation. Clin Transplant 2012;26:E471-84. [Crossref] [PubMed]
- Bellin MD, Barton FB, Heitman A, et al. Potent induction immunotherapy promotes long-term insulin independence after islet transplantation in type 1 diabetes. Am J Transplant 2012;12:1576-83. [Crossref] [PubMed]
- Shapiro AM. State of the art of clinical islet transplantation and novel protocols of immunosuppression. Curr Diab Rep 2011;11:345-54. [Crossref] [PubMed]
- Bramis K, Gordon-Weeks AN, Friend PJ, et al. Systematic review of total pancreatectomy and islet autotransplantation for chronic pancreatitis. Br J Surg 2012;99:761-6. [Crossref] [PubMed]
- Stevens RB LJ, Boerner BP, Miles CD, et al. Single-dose rATG induction at renal transplantation: superior renal function and glucoregulation with less hypomagnesemia. Clin Transplant 2012;26:123-32. [Crossref] [PubMed]
- Matsuoka N, Itoh T, Watarai H, et al. High-mobility group box 1 is involved in the initial events of early loss of transplanted islets in mice. J Clin Invest 2010;120:735-43. [Crossref] [PubMed]
- Tjernberg J, Ekdahl KN, Lambris JD, et al. Acute antibody-mediated complement activation mediates lysis of pancreatic islets cells and may cause tissue loss in clinical islet transplantation. Transplantation 2008;85:1193-9. [Crossref] [PubMed]
- Teramura Y, Iwata H. Surface modification of islets with PEG-lipid for improvement of graft survival in intraportal transplantation. Transplantation 2009;88:624-30. [Crossref] [PubMed]
- Ito T, Omori K, Rawson J, et al. Improvement of canine islet yield by donor pancreas infusion with a p38MAPK inhibitor. Transplantation 2008;86:321-9. [Crossref] [PubMed]
- Spirig R, Gajanayake T, Korsgren O, et al. Low molecular weight dextran sulfate as complement inhibitor and cytoprotectant in solid organ and islet transplantation. Mol Immunol 2008;45:4084-94. [Crossref] [PubMed]
- Johansson H, Goto M, Dufrane D, et al. Low molecular weight dextran sulfate: a strong candidate drug to block IBMIR in clinical islet transplantation. Am J Transplant 2006;6:305-12. [Crossref] [PubMed]
- Barshes NR, Wyllie S, Goss JA. Inflammation-mediated dysfunction and apoptosis in pancreatic islet transplantation: implications for intrahepatic grafts. J Leukoc Biol 2005;77:587-97. [Crossref] [PubMed]
- Goto M, Johansson H, Maeda A, et al. Low molecular weight dextran sulfate prevents the instant blood-mediated inflammatory reaction induced by adult porcine islets. Transplantation 2004;77:741-7. [Crossref] [PubMed]
- Clayton HA, Davies JE, Pollard CA, et al. Pancreatectomy with islet autotransplantation for the treatment of severe chronic pancreatitis: the first 40 patients at the leicester general hospital. Transplantation 2003;76:92-8. [Crossref] [PubMed]
- White SA, Davies JE, Pollard C, et al. Pancreas resection and islet autotransplantation for end-stage chronic pancreatitis. Ann Surg 2001;233:423-31. [Crossref] [PubMed]
- Pollard C, Gravante G, Webb M, et al. Use of the recanalised umbilical vein for islet autotransplantation following total pancreatectomy. Pancreatology 2011;11:233-9. [Crossref] [PubMed]
- Takita M, Matsumoto S, Noguchi H, et al. One hundred human pancreatic islet isolations at Baylor Research Institute. Proc (Bayl Univ Med Cent) 2010;23:341-8. [Crossref] [PubMed]
- Matsumoto S, Noguichi H, Shimoda M, et al. Seven consecutive successful clinical islet isolations with pancreatic ductal injection. Cell Transplant 2010;19:291-7. [Crossref] [PubMed]
- Matsumoto S, Rigley TH, Qualley SA, et al. Efficacy of the oxygen-charged static two-layer method for short-term pancreas preservation and islet isolation from nonhuman primate and human pancreata. Cell Transplant 2002;11:769-77. [Crossref] [PubMed]
- O'Connell PJ, Hawthorne WJ, Holmes-Walker DJ, et al. Clinical islet transplantation in type 1 diabetes mellitus: results of Australia's first trial. Med J Aust 2006;184:221-5. [Crossref] [PubMed]
- Ricordi C, Lacy PE, Scharp DW. Automated islet isolation from human pancreas. Diabetes 1989;38 Suppl 1:140-2. [Crossref] [PubMed]
- Zammit NW, Tan BM, Walters SN, et al. Low-dose rapamycin unmasks the protective potential of targeting intragraft NF-kappaB for islet transplants. Cell Transplant 2013;22:2355-66. [Crossref] [PubMed]
- Cantley J, Walters SN, Jung MH, et al. A preexistent hypoxic gene signature predicts impaired islet graft function and glucose homeostasis. Cell Transplant 2013;22:2147-59. [Crossref] [PubMed]
- Cowley MJ, Weinberg A, Zammit NW, et al. Human islets express a marked proinflammatory molecular signature prior to transplantation. Cell Transplant 2012;21:2063-78. [Crossref] [PubMed]
- Campbell PD, Weinberg A, Chee J, et al. Expression of pro- and antiapoptotic molecules of the Bcl-2 family in human islets postisolation. Cell Transplant 2012;21:49-60. [Crossref] [PubMed]
- Ozmen L, Ekdahl KN, Elgue G, et al. Inhibition of thrombin abrogates the instant blood-mediated inflammatory reaction triggered by isolated human islets: possible application of the thrombin inhibitor melagatran in clinical islet transplantation. Diabetes 2002;51:1779-84. [Crossref] [PubMed]
- White SA, James RF, Swift SM, et al. Human islet cell transplantation--future prospects. Diabet Med 2001;18:78-103. [Crossref] [PubMed]
- Eizirik DL, Mandrup-Poulsen T. A choice of death--the signal-transduction of immune-mediated beta-cell apoptosis. Diabetologia 2001;44:2115-33. [Crossref] [PubMed]
- Arnush M, Heitmeier MR, Scarim AL, et al. IL-1 produced and released endogenously within human islets inhibits beta cell function. J Clin Invest 1998;102:516-26. [Crossref] [PubMed]
- Naziruddin B, Kanak MA, Chang CA, et al. Improved outcomes of islet autotransplant after total pancreatectomy by combined blockade of IL-1beta and TNFalpha. Am J Transplant 2018;18:2322-9. [Crossref] [PubMed]
- Onaca N, Takita M, Levy MF, et al. Anti-inflammatory Approach With Early Double Cytokine Blockade (IL-1beta and TNF-alpha) Is Safe and Facilitates Engraftment in Islet Allotransplantation. Transplant Direct 2020;6:e530. [Crossref] [PubMed]
- Lai Y, Chen C, Linn T. Innate immunity and heat shock response in islet transplantation. Clin Exp Immunol 2009;157:1-8. [Crossref] [PubMed]
- Li S, Li X, Yang M, et al. Identification of the Subsets of IL-10-Producing Regulatory B Cells in the Course of Tolerance Induction and Maintenance in Islet Allotransplantation. Transplant Proc 2018;50:3900-5. [Crossref] [PubMed]
- Pennline KJ, Roque-Gaffney E, Monahan M. Recombinant human IL-10 prevents the onset of diabetes in the nonobese diabetic mouse. Clin Immunol Immunopathol 1994;71:169-75. [Crossref] [PubMed]
- Huurman VA, Velthuis JH, Hilbrands R, et al. Allograft-specific cytokine profiles associate with clinical outcome after islet cell transplantation. Am J Transplant 2009;9:382-8. [Crossref] [PubMed]
- Jansson L, Carlsson PO. Graft vascular function after transplantation of pancreatic islets. Diabetologia 2002;45:749-63. [Crossref] [PubMed]
- Vasir B, Jonas JC, Steil GM, et al. Gene expression of VEGF and its receptors Flk-1/KDR and Flt-1 in cultured and transplanted rat islets. Transplantation 2001;71:924-35. [Crossref] [PubMed]
- Koskinen AR, Tukiainen E, Arola J, et al. Complement activation during liver transplantation-special emphasis on patients with atypical hemolytic uremic syndrome. Am J Transplant 2011;11:1885-95. [Crossref] [PubMed]
- He S, Atkinson C, Qiao F, et al. A complement-dependent balance between hepatic ischemia/reperfusion injury and liver regeneration in mice. J Clin Invest 2009;119:2304-16. [Crossref] [PubMed]
- Chung WY, Pollard CA, Stover C, et al. Pilot study: deficiency of mannose-binding lectin-dependent lectin pathway, a novel modulator in outcome from pancreatic islet auto-transplantation. Ann Transl Med 2020;8:170. [Crossref] [PubMed]
- Cedzynski M, Atkinson AP, St Swierzko A, et al. L-ficolin (ficolin-2) insufficiency is associated with combined allergic and infectious respiratory disease in children. Mol Immunol 2009;47:415-9. [Crossref] [PubMed]