Distinct prognostic values of programmed death-ligand 1 and programmed cell death protein 1 in lung adenocarcinoma and squamous cell carcinoma patients
Introduction
The checkpoint inhibitors that target the programmed cell death protein 1 (PD-1)/programmed death-ligand 1 (PD-L1) pathway represent a significant breakthrough in the treatment of non-small cell lung cancer (NSCLC) (1-5). However, only 15–20% of patients who receive PD-1/PD-L1 inhibitor therapy show a clinical response without screening. Therefore, elucidating the factors that could determine which patients would benefit from this treatment has become a primary priority.
The expression of PD-L1 has been extensively investigated as a predictive biomarker in human cancer. Most previous studies have found a high expression of PD-L1 correlated with an improved response rate (2,3); however, clinical response has also been observed in PD-L1 negative patients (6) the response in PD-L1-positive patients is unsatisfactory. Multiple factors can explain this limitation; firstly, a uniform assay is lacking for the assessment of PD-L1 (2-7); secondly, the immune tumor microenvironment (TME) is complex, with functional interrelation of several factors (8,9); and finally, tumor heterogeneity might lead to differential expression of PD-L1 between different tumors in the same patient (7,10,11).
The status of immunosuppression is also considered to be related to tumor development in patients. Immune factors such as PD-L1 and PD-1 may serve as prognostic biomarkers indicating the patient’s survival, independent of the immune checkpoint inhibitors received. Therefore, we set out to investigate the prognosis that is unrelated to treatment and reflects the tumor’s intrinsic factors.
Specifically, the two clinical trials of OPDIVO® (nivolumab) in previously treated NSCLC patients, CheckMate 017 for squamous cell carcinoma (SCC) and CheckMate 057 for non-squamous (non-SCC) NSCLC, showed similar clinical improvement in the overall survival (OS) rate; however, the predictive role of PD-L1 was variable (1,2). No association was found between PD-L1 expression and SCC response, but a significant association was observed in non-SCC NSCLC. These findings indicated that the expression and biological significance of the PD-L1/PD-1 pathway might differ between these two cancer types. However, most of the studies to date have analyzed PD-L1 and PD-1 in the total population of NSCLC patients, ignoring the potential effects of their divergence. This could partly explain the inconsistent results of studies on their prognostic roles in NSCLC (11-17). Therefore, it is necessary to assess the PD-L1/PD-1 pathway differences in different pathological types of NSCLC.
Hence, in the current study, an integrated analysis that incorporated results from a public database and two independent NSCLC patients' independent cohorts was undertaken. PD-L1 and PD-1 expression, and CD8+ T-cell infiltration were detected by immunohistochemistry (IHC) and multiplexed IHC staining (mIHC), and their prognostic values in lung adenocarcinoma (ADC) and SCC were analyzed, respectively. From the results, we found distinct prognostic biomarkers that potentially reflect T cell function in NSCLC subgroups. We present the following article following the REporting recommendations for tumor MARKer (REMARK) reporting checklist (available at http://dx.doi.org/10.21037/atm-20-968).
Methods
Study population
This study was conducted on two independent cohorts of NSCLC patients from Shengjing Hospital of China Medical University (cohort 1) and the First Hospital of China Medical University (cohort 2). Samples of NSCLC tissue from the archive of the institute of Pathology were reviewed. Clinical data including sex, age at diagnosis, smoking status, tumor differentiation, and epidermal growth factor receptor (EGFR) mutation status were collected from patient medical records, and pathological tumor node metastasis (TNM) stage was defined by the American Joint Committee on Cancer (AJCC) 7th edition. The inclusion criteria were: <75 years old; primary NSCLC with stage I–III; R0 resection; pathological type classified as SCC or ADC; and treatment-naive before surgery. The patients received standard therapy after surgery, either platinum-based adjuvant chemotherapy or observation, according to their TNM stage and the National Comprehensive Cancer Network (NCCN) guidelines.
The Shengjing Hospital cohort comprised retrospectively collected formalin-fixed paraffin-embedded (FFPE) samples from 118 lung carcinomas between January 2010 and December 2010, with an average follow-up of 56 months (range, 3–66 months). The First Hospital cohort consisted of 144 lung carcinomas between 2012 and 2014, with an average follow-up of 55 months (range, 7–75 months). A detailed description of the baseline characteristics of the two cohorts is displayed in Table S1. The study was conducted in line with the Declaration of Helsinki (as revised in 2013) and approved by the Ethics Committee at the First Hospital of China Medical University (AF-SOP-07-1.1-01). All patients signed informed consent form.
IHC
Tissue samples were prepared and preserved in FFPE. Serial 4-µm-thick sections were prepared through deparaffination in xylene and dehydration with graded alcohols. For IHC staining, the procedure was performed followed by the standard procedure for the UltraSensitiveTM SP (Mouse/Rabbit) IHC Kit (#9710, Maixin Biotech, Fuzhou, Fujian, China). Firstly, heat-induced antigen retrieval in citrate buffer was conducted. After blocking with bovine serum albumin (BSA), the sections were incubated with the following primary antibodies at 4 °C overnight: PD-L1 (#13684, clone E1L3N, CST), CD8 (MAB-0021, clone c8/144B, Maixin Biotech, Fuzhou, Fujian, China), PD-1 (#86163, clone D4W2J, CST). Next, the sections were treated with second antibody-peroxidase-linked polymers and visualized with 3,3-diamino-benzidine tetrahydrochloride (DAB; Maixin Biotech, China).
Evaluation of IHC
All specimens were examined independently by two experienced pathologists who were blinded to all clinical outcomes. In cases of disagreement between the two pathologists, a third pathologist was consulted to achieve consensus. Tumor cells (TCs) and tumor-infiltrating immune cells (TIICs) were scored separately. PD-L1 positivity in TCs was defined as ≥5% TCs having membrane staining. For immune cells (ICs), positivity was defined as ≥1% of the TIICs in the tumor area being PD-L1 positive (5). Either TC or IC positivity was considered as PD-L1 positive. PD-1 in tumor-infiltrating lymphocytes (TILs) was assessed by counting the percentage of PD-1-positive TILs; PD-1 positivity was counted as ≥1% of the TILs being PD-1 positive. The value of CD8 was evaluated on the extent of positive lymphocytes infiltrating within TCs. Staining of CD8 cells was defined as less or more if the tumor site’s median value was <20% or ≥20%.
Multiplexed IHC staining (mIHC)
mIHC was performed using the OpalTM7-Color Manual IHC Kit (PerkinElmer Inc., Waltham, MA, USA) according to the manufacturer’s instructions. The primary antibodies used were the same as those used for IHC staining. Briefly, antigen retrieval was carried out in ethylene-diamine-tetraacetic acid (EDTA) buffer (pH 9.0) using microwave incubation (MWT) for 10 min. After cooling down, slides were covered with blocking buffer and incubated in a humidified chamber for 10 min at room temperature. Primary antibodies for CD8 were incubated for 1 h at room temperature followed by detection using the Opal Polymer HRP Ms+Rb (PerkinElmer Inc., Waltham, MA, USA) for 10 min. Opal signal generation of CD8 was accomplished using Opal 690 TSA Plus for 10 min, after which the slides were placed in EDTA buffer and heated using MWT to remove primary and secondary antibodies. Serially, we staining for PD-1, PD-L1, and pan-CK (MAB-0671, and clone MX005, Maixin Biotech, Fuzhou, Fujian, China) was performed. Nuclei were subsequently stained with 4’,6-diamidino-2-phenylindole (DAPI) for 5 min, and the sections were coverslipped with Vectashield HardSet mounting media (Vector Laboratories Inc., Burlingame, CA, USA).
Imaging and analysis
The slides were scanned using the Mantra System (PerkinElmer, Waltham, MA, USA). To establish the spectral library required for multispectral unmixing, images of unstained tissue and single-stained tissues were used to extract the autofluorescence spectrums of tissue and each fluorophore. The machine-learning platform InForm (PerkinElmer, Waltham, MA, USA) was used for tissue segmentation, cell segmentation, and intensity measurements. The marker creatine kinase (CK) was used to identify TCs; PD-1 and CD8 were used to detect TILs. Different cell types were quantified as a proportion to all cells. The levels of IC PD-L1, PD-1, and CD8 were classified as positive/negative using the median as the cutoff. Positivity of TC PDL-1 was defined as ≥1%.
Messenger RNA (mRNA) expression profiling and Gene Ontology (GO) analysis
For The Cancer Genome Atlas (TCGA) datasets, the RNA sequencing (RNA-Seq) (level 2) data of 594 adenocarcinomas and 551 SCCs were downloaded from the TCGA website and preprocessed according to the Bioconductor/TCGAbiolinks package. Genes co-expressed with PD-L1 in TCGA were selected for GO analysis by the Database for Annotation, Visualization and Integrated Discovery (DAVID, https://david.ncifcrf.gov). Seven Gene Expression Omnibus (GEO) datasets were enrolled for meta-analysis; four datasets (GSE37745, GSE30219, GSE8894, and GSE19188) that consisted of ADC and SCC groups were selected for comparative analysis. The cut-off values were chosen as the median of the entire dataset.
Statistical analysis
Analyses were performed using SPSS version 16.0 (IBM Corp., Armonk, NY, USA) and R software 3.0.2 (R Foundation for Statistical Computing, Vienna, Austria, https://www.R-project.org/). Associations between clinicopathological parameters and PD-L1/PD-1 expression were assessed with the chi-squared (2) or Fisher’s exact test, as appropriate. Spearman’s correlation coefficients were obtained to explore the relationships between PD-L1 and CD8 expression. Welch’s two-sample t-test was used to compute the P value for continuous variables. Kaplan-Meier analysis and log-rank tests were used for survival analysis. To evaluate the prognostic value of PD-L1 and PD-1, univariate and multivariate analyses using a Cox proportional hazard regression classification were carried out, and hazard ratios (HRs) were estimated with 95% confidence interval (CI) limits. A P value <0.05 was considered statistically significant.
A meta-analysis based on the SurvExpress database was carried out. The study names, sample size, HR, and 95% CI were extracted. The pooled HR with a 95% CI was obtained by calculating the weighted average of the individual log (HR) estimates. A pooled HR >1 implied a worse survival for the group with high expression. Data were considered statistically significant if the 95% CI for the combined HR did not overlap.
Results
Patient characteristics
Cohort 1 comprised 70 ADC and 48 SCC patients, with a median age of 59 years (range, 30–75 years). At the final follow-up (June, 2015), 55 (46.7%) patients had relapsed and 47 (39.8%) patients had died due to the disease. Cohort 2 included 98 ADC and 46 SCC patients, with a median age of 59 years (range, 34–75 years). At the final follow-up (June, 2018), 71 (49.3%) patients had relapsed and 41 (28.5%) patients had died due to the disease. Information on the smoking status, histological type, TNM stage, tumor differentiation, and EGFR mutation status of ADC and SCC patients in the two independent cohorts is summarized in Table S1. There were no significant differences between the two cohorts in terms of baseline characteristics.
The expression levels of PD-L1 and PD-1 mRNA
First, the expression levels of PD-L1 mRNA were compared between ADC and SCC. The GEO datasets showed that the PD-L1 mRNA levels in the SCC group were increased compared to those in the ADC group (Figure S1A,B,C), except for GSE19188, in which statistical significance was not achieved (Figure S1D). In contrast with PD-L1, little difference was observed in PD-1 mRNA levels between the SCC and ADC subtypes in the GEO datasets (Figure S1E,F,G,H).
PD-L1 expression was increased in the SCC group compared to the ADC group
Next, IHC staining was used to investigate PD-L1 protein expression in cohort 1. Typically, the staining of PD-L1 is membrane-accentuated, which was often accompanied by low-intensity cytoplasmic staining. A total of 41/118 patients (34.7%) showed PD-L1 positivity in TCs, and 71/118 patients (60.2%) in ICs. PD-L1 was expressed more frequently in the SCC sub-type (Figure 1A,B,C) than in the ADC sub-type (Figure 1D,E,F) (TCs: 54.2% in SCC vs. 21.4% in ADC; ICs: 85.4% in SCC vs. 42.9% in ADC, P<0.05).
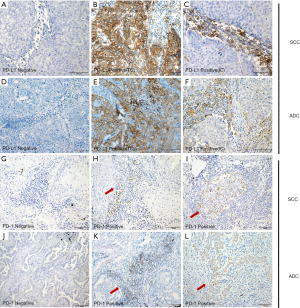
For PD-1 expression, most PD-1pos cells were distributed in the stromal bands dissecting the tumor mass or within tumor-infiltrating IC small aggregates, whereas others were expressed on TCs. In cohort 1, 45/118 patients (38.1%) were defined as IC PD-1pos, and no significant difference was found in positivity between the two subtypes (39.6% in SCC vs. 37.1% in ADC, P>0.05) (Figure 1G,H,I,J,K,L).
Expression of PD-L1 and PD-1 by mIHC
The assessment of immune markers by IHC was semi-quantitative and relatively crude. Therefore, to quantify and localize ICs in a more detailed manner, multiplexed immunofluorescence staining was conducted in cohort 2. Consistent with the IHC results, PD-L1 expression was more commonly observed in ICs than in TCs (IC median 3.52%, 0.02–86.76% vs. TC median 0.5%, 0–35.36%) (Figure 2A). In total, 52/144 patients (36.1%) were found to be TC PD-L1pos (30.6% in ADC vs. 47.8% in SCC, P<0.05). Using the median as the cut-off value, 43.9% and 63.0% of patients in the ADC and SCC groups, respectively, were defined as IC PD-L1pos.
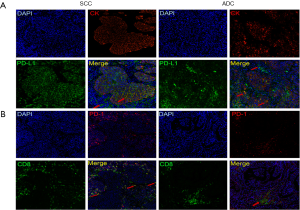
In cohort 2, the distribution of PD-1 was similar, but not completely coincident, to that of CD8, indicating that PD-1 was not limited to T cells (Figure 2B). The positivity of PD-1 in ADC and SCC was 49.0% and 45.7%, respectively.
Expression of CD8+ T lymphocytes
For further assessment, genes co-existing with PD-L1 in TCGA were predicted. A total of 60 genes in ADC and 2 genes (PD-L1 and PD-1) in SCC were selected with a Pearson correlation coefficient of ≥0.4. These genes were annotated in the GO analysis, and the results indicated that the genes co-expressed with PD-L1 in ADC mostly participated in immune response (Table S2). Nevertheless, as there were too few genes to evaluate, further analysis was impossible in SCC.
As CD8+ T cells play a central role in immune response, CD8+ T cells’ expressions were assessed first. Conventional IHC staining showed CD8+ T cells to have a diffuse pattern, with more cells in the peritumoral areas than within the tumor nests (Figure 3A). Using 20% as the cut-off value, almost half of the patients (53/118, 44.9%) had more CD8+ T cell infiltration. The SCC group demonstrated significantly higher CD8+ T cells levels compared to the ADC group (58.3% in SCC vs. 35.7% in ADC, P<0.05). According to mIHC staining, three patterns of T cells associated with TCs were demonstrated: (I) CD8+ TILs infiltrating the peritumoral and tumor areas simultaneously (Figure 3B); (II) CD8+ TILs limited to the peritumoral areas, with little infiltration of tumor areas (Figure 3C); and (III) very low T-cell infiltration (data not shown).
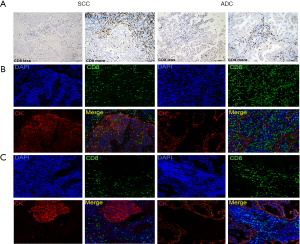
Different relationships between PD-L1 and CD8+ T lymphocytes
Next, the correlations of CD8+ T cells with PD-L1 were examined in the subgroups. Increasing density of CD8+ TILs showed a positive correlation with IC PD-L1 in both the ADC and SCC groups. In the ADC group, CD8+ TILs were positively correlated with TC PD-L1; however, no significant relationship was observed in the SCC group (Table S3). These results suggested that the mechanisms in the up-regulation of PD-L1 were potentially different between ICs and TCs, and especially between ADC and SCC.
PD-L1 was the survival predictor for ADC patients
A meta-analysis of TCGA and GEO databases was carried out to evaluate the prognostic value of PD-L1. There were 7 studies including 1,115 ADC participants and 8 studies including 656 SCC enrolled participants. The main characteristics of these studies are summarized in Table S4. As the heterogeneity test showed no significant differences (I2=18.6% and 0 in ADC and SCC, P=0.2883 and 0.9101, respectively), a fixed-effects model was used. The results showed that high PD-L1 mRNA levels were strongly correlated with shorter OS in ADC population (pooled HR =1.27, 95% CI, 1.01–1.58, Figure S2A). However, the 95% CI was overlapped for the SCC population, indicating no such correlation (Figure S2B).
To confirm these observations from public databases, the relationship of PD-L1 expression with OS and disease-free survival (DFS) were evaluated in the two cohorts. In Kaplan-Meier analysis, ADC patients with PD-L1+ TCs (Figure 4A,B,C,D) or ICs (Figure 4E,F,G,H) had worse OS and DFS than patients with PD-L1– TCs or ICs. In the SCC population, PD-L1 expression showed no significant prognostic value for OS or DFS (Figure 4I,J,K,L,M,N,O,P).
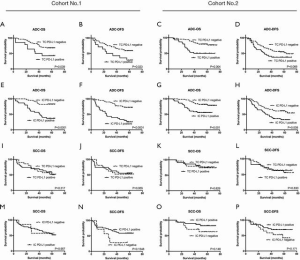
PD-1+ TIL predicted survival for SCC patients
Next, the TCGA and GEO databases were meta-analyzed again, as previously described. PD-1 mRNA level was not found to be correlated with OS in either the ADC (Figure S2C) or the SCC (Figure S2D) population. PD-1+ TILs, as assessed by IHC in cohort 1, were not associated with OS or DFS in ADC patients (Figure 5A,B); however, PD-1+ TILs were associated with worse OS and DFS in SCC patients (Figure 5C,D). In cohort 2, the overall count of PD-1+ cells in the stroma was again observed to have no prognostic value in either group (Figure 5E,F,G,H). This result was similar to that arising from online data analysis. Since we found in cohort 1 that PD-1+ TILs had a prognostic effect, we then investigated whether the combination of PD-1 stroma expression and CD8+ T cells could identify sub-groups of patients with a distinct prognosis in cohort 2. As expected, a low CD8+ PD-1+ T-cell count was related to superior survival only in the SCC subgroup (P=0.018 for OS and P=0.01 for DFS) (Figure 5I,J,K,L).
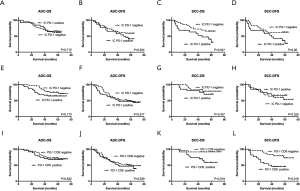
Univariate and multivariate analyses of independent prognostic factors
In univariate analysis, PD-L1 expression (either TC or IC), tumor differentiation, and pTNM stage were associated with OS in ADC patients and were then selected for multivariate analyses. For SCC patients, pTNM stage, PD-1+ TILs, or CD8+ PD-1 showed associations with OS (in cohort 1, tumor differentiation was also selected for multivariate analyses with P=0.058) (Table 1 and Table S5). Finally, multivariate analysis of the ADC sub-group showed that PD-L1 expression was an independent predictor of OS (HR =2.682, P=0.031 in cohort 1; HR =2.658, P=0.028 in cohort 2) as was TNM stage (HR =2.377, P=0.002 in cohort 1; HR =2.507, P<0.001 in cohort 2). Meanwhile, in the SCC sub-group, PD-1+ TILs (HR =5.143, P=0.037 in cohort 1, HR =2.304, P=0.053 in cohort 2) and TNM stage (HR =5.945, P=0.013 in cohort 1; HR =2.482, P=0.003 in cohort 2) were considered to be independent predictors of poorer OS.
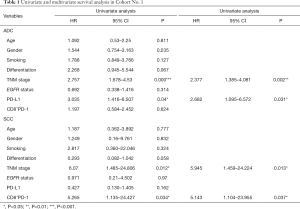
Full table
Discussion
This study was driven by the question of whether or not PD-L1 expression exhibited different predictive values in ADC and SCC in clinical trials; thus, we explored the differences in expression and function of PD-L1/PD-1 in ADC and SCC through an extensive analysis. Interestingly, we found that PD-L1/PD-1 up-regulation mechanisms and their prognostic values differed between the two subtypes. As the current checkpoint blockade therapies mainly focus on sustaining T cells against exhaustion, T cells' function was considered a critical predictor for both prognosis and clinical response (17-20). Therefore, we inferred that the biomarkers indicating T cell function were distinct between the two sub-types.
Adaptive and intrinsic immune resistance modalities are mechanisms for regulating PD-L1 expression by TCs. PD-L1 up-regulation in response to IFN-γ secreted by CD8+ T cells reflects adaptive resistance, which in turn represents a dynamic biomarker of active inflammation (21,22). Meanwhile, intrinsic resistance is observed in increased PD-L1 expression secondary to constitutive oncogene activation (23-27). Staining of NSCLC specimens in this study showed that PD-L1 expression on ICs was significantly correlated with CD8+ T cell density in both subgroups, indicating an adaptive resistance. As for PD-L1 in TCs, a positive correlation with CD8+ T cells was observed in ADC, but not in SCC. Besides, GO analysis based on the TCGA database showed immune response with PD-L1 only in ADC, further supporting the hypothesis of a distinct regulatory mechanism of PD-L1 in TCs. This means that the PD-L1 expression in ADC TCs might occur due to the adaptive resistance or combined action of adaptive resistance and an intrinsic mechanism. Although PD-L1 was more frequently and more intensively expressed in SCC, it showed no up-regulation upon stimulation of CD8+ T cells. Thus, intrinsic resistance is perhaps considered as the predominant regulatory mechanism in SCC. These findings, to some extent, support our speculation that PD-L1 expression in ADC may represent the state of CD8+ T cells and thus serve as a prognostic marker.
TILs are another key factor in adaptive immunity. However, in cancer, sustained up-regulation of inhibitory receptors, on TILs has been found to lead to functional deficiency of TILs (28), and this dysfunctional state of T cells has been defined as T-cell exhaustion (18,29-31). PD-1 is the major inhibitory receptor that regulates T-cell exhaustion, and the associated clinical benefits obtained by antibodies of PD-1 or PD-L1 highlight the importance of this mechanism. Previous research has proven that the effect or function of PD-1+ TILs in NSCLC is impaired (18); however, PD-1+ CD8+ T cells have been found to show a strong correlation with anti-PD1 therapy (18,32). This shows that PD-1 expression can affect the function of CD8+ T cells. In our study, CD8+ PD-1+ was considered as a predictive biomarker of shorter OS and DFS in SCC, indicating a state of T-cell dysfunction.
To date, no consensus regarding the association of PD-L1 and PD-1 with survival has been reached. The observations presented in our paper might reflect inherent differences in the immune environments of ADC and SCC. We also demonstrated a negative correlation of PD-L1 and PD-1 between different prognoses. Although there is evidence that TILs are associated with immune response, the prognostic predictive value of CD8+ T cells remain controversial (33-35). A possible reason for this is that the count of CD8+ T cells was insufficient to reflect the immune status, as T cells’ function was more important. In ADC, the up-regulation of PD-L1 was subsequently associated with adaptive immune resistance and the induction of T-cell suppression through PD-L1 and PD-1 binding. The induction of PD-L1 is the key step during this process; thus, PD-L1 could be used as a prognostic and predictive marker. However, PD-L1 was found to be up-regulated due to intrinsic immune resistance and pre-existence in SCC. Concurrently, PD-1 expression, which is especially expressed on TILs, reflected the status of the TME in triggering the reaction.
Some studies may have offered possible molecular evidence of involvement in suppressing immune responses in SCC patients in recent years. Leclerc et al. reported neuropilin-1 (Nrp-1) to define a subset of CD8+ T cells that can display PD-1hi status and negatively influence CD8+ T cell immunity (36). Another report has identified CD103 and its ligand E-cadherin as important adhesion molecules that promote TIL anti-tumor functions in human lung tumors (37).
A limitation of this research was that it did not include data on therapy in post-operative recurrence cases, which have could influence the OS time of patients, especially with the development of novel agents for lung cancer in recent years. To some extent, DFS, which was unaffected by therapy for post-operative recurrence, was analyzed to address this problem. The clinical relevance of treatment factors with the PD-1/PD-L1 pathway and OS needs to be elucidated in future research. Another limitation is that these findings were established in a relatively smaller cohort, thus larger future studies are warranted for confirmation. Lastly, although we have uncovered the difference between NSCLC subtypes from the perspective of such a phenomenon, the specific molecular mechanism remains unclear and will focus on our future research.
In conclusion, the current findings give an insight into the distinct factors that indicate the T cell state between ADC and SCC. Different regulatory mechanisms and prognostic values of PD-L1 have also been discovered. This work expanded our knowledge of the PD-L1/PD-1 pathway and provides a theoretical basis for optimizing current immunotherapy.
Acknowledgments
Funding: This work was supported by National Science and Technology Major Project of the Ministry of Science and Technology of China (2017ZX09304025); the National Natural Science Foundation of China (81602098, 81673025, and 31300743); Science and Technology Plan Project of Liaoning Province (2014225013, 2014226033, and 2016007010); Distinguished professor of Liaoning Province; The General Project of Liaoning Province Department of Education (LZ2014037).
Footnote
Reporting Checklist: The authors have completed the REMARK reporting checklist. Available at http://dx.doi.org/10.21037/atm-20-968
Data Sharing Statement: Available at http://dx.doi.org/10.21037/atm-20-968
Peer Review File: Available at http://dx.doi.org/10.21037/atm-20-968
Conflicts of Interest: All authors have completed the ICMJE uniform disclosure form (available at http://dx.doi.org/10.21037/atm-20-968). The authors have no conflicts of interest to declare.
Ethical Statement: The authors are accountable for all aspects of the work in ensuring that questions related to the accuracy or integrity of any part of the work are appropriately investigated and resolved. The study was conducted in line with the Declaration of Helsinki (as revised in 2013) and approved by the Ethics Committee at the First Hospital of China Medical University (AF-SOP-07-1.1-01). All patients signed informed consent form.
Open Access Statement: This is an Open Access article distributed in accordance with the Creative Commons Attribution-NonCommercial-NoDerivs 4.0 International License (CC BY-NC-ND 4.0), which permits the non-commercial replication and distribution of the article with the strict proviso that no changes or edits are made and the original work is properly cited (including links to both the formal publication through the relevant DOI and the license). See: https://creativecommons.org/licenses/by-nc-nd/4.0/.
References
- Brahmer J, Reckamp KL, Baas P, et al. Nivolumab versus Docetaxel in Advanced Squamous-Cell Non-Small-Cell Lung Cancer. N Engl J Med 2015;373:123-35. [Crossref] [PubMed]
- Borghaei H, Paz-Ares L, Horn L, et al. Nivolumab versus Docetaxel in Advanced Nonsquamous Non-Small-Cell Lung Cancer. N Engl J Med 2015;373:1627-39. [Crossref] [PubMed]
- Herbst RS, Baas P, Kim DW, et al. Pembrolizumab versus docetaxel for previously treated, PD-L1-positive, advanced non-small-cell lung cancer (KEYNOTE-010): a randomised controlled trial. Lancet 2016;387:1540-50. [Crossref] [PubMed]
- Carbone DP, Reck M, Paz-Ares L, et al. First-Line Nivolumab in Stage IV or Recurrent Non-Small-Cell Lung Cancer. N Engl J Med 2017;376:2415-26. [Crossref] [PubMed]
- Wu YL, Lu S, Cheng Y, et al. Nivolumab Versus Docetaxel in a Predominantly Chinese Patient Population With Previously Treated Advanced Non-Small Cell Lung Cancer: CheckMate 078 Randomized Phase III Clinical Trial. J Thorac Oncol 2019;14:867-75. [Crossref] [PubMed]
- Topalian SL, Hodi FS, Brahmer JR, et al. Safety, activity, and immune correlates of anti-PD-1 antibody in cancer. N Engl J Med 2012;366:2443-54. [Crossref] [PubMed]
- McLaughlin J, Han G, Schalper KA, et al. Quantitative Assessment of the Heterogeneity of PD-L1 Expression in Non-Small-Cell Lung Cancer. JAMA Oncol 2016;2:46-54. [Crossref] [PubMed]
- Tang H, Wang Y, Chlewicki LK, et al. Facilitating T Cell Infiltration in Tumor Microenvironment Overcomes Resistance to PD-L1 Blockade. Cancer Cell 2016;29:285-96. [Crossref] [PubMed]
- Fridman WH, Pages F, Sautes-Fridman C, et al. The immune contexture in human tumours: impact on clinical outcome. Nat Rev Cancer 2012;12:298-306. [Crossref] [PubMed]
- Ilie M, Long-Mira E, Bence C, et al. Comparative study of the PD-L1 status between surgically resected specimens and matched biopsies of NSCLC patients reveal major discordances: a potential issue for anti-PD-L1 therapeutic strategies. Ann Oncol 2016;27:147-53. [Crossref] [PubMed]
- Velcheti V, Schalper KA, Carvajal DE, et al. Programmed death ligand-1 expression in non-small cell lung cancer. Lab Invest 2014;94:107-16. [Crossref] [PubMed]
- Tsao MS, Le Teuff G, Shepherd FA, et al. PD-L1 protein expression assessed by immunohistochemistry is neither prognostic nor predictive of benefit from adjuvant chemotherapy in resected non-small cell lung cancer. Ann Oncol 2017;28:882-9. [Crossref] [PubMed]
- Mao Y, Li W, Chen K, et al. B7-H1 and B7-H3 are independent predictors of poor prognosis in patients with non-small cell lung cancer. Oncotarget 2015;6:3452-61. [Crossref] [PubMed]
- Uruga H, Bozkurtlar E, Huynh TG, et al. Programmed Cell Death Ligand (PD-L1) Expression in Stage II and III Lung Adenocarcinomas and Nodal Metastases. J Thorac Oncol 2017;12:458-66. [Crossref] [PubMed]
- Scheel AH, Ansen S, Schultheis AM, et al. PD-L1 expression in non-small cell lung cancer: Correlations with genetic alterations. Oncoimmunology 2016;5:e1131379. [Crossref] [PubMed]
- Yang CY, Lin MW, Chang YL, et al. Programmed cell death-ligand 1 expression in surgically resected stage I pulmonary adenocarcinoma and its correlation with driver mutations and clinical outcomes. Eur J Cancer 2014;50:1361-9. [Crossref] [PubMed]
- Azuma K, Ota K, Kawahara A, et al. Association of PD-L1 overexpression with activating EGFR mutations in surgically resected nonsmall-cell lung cancer. Ann Oncol 2014;25:1935-40. [Crossref] [PubMed]
- Lavin Y, Kobayashi S, Leader A, et al. Innate Immune Landscape in Early Lung Adenocarcinoma by Paired Sin-gle-Cell Analyses. Cell 2017;169:750-765.e17. [Crossref] [PubMed]
- Thommen DS, Koelzer VH, Herzig P, et al. A transcriptionally and functionally distinct PD-1(+) CD8(+) T cell pool with predictive potential in non-small-cell lung cancer treated with PD-1 blockade. Nat Med 2018;24:994-1004. [Crossref] [PubMed]
- Simoni Y, Becht E, Fehlings M, et al. Bystander CD8(+) T cells are abundant and phenotypically distinct in human tumour infiltrates. Nature 2018;557:575-9. [Crossref] [PubMed]
- Chevrier S, Levine JH, Zanotelli VRT, et al. An Immune Atlas of Clear Cell Renal Cell Carcinoma. Cell 2017;169:736-749.e18. [Crossref] [PubMed]
- Spranger S, Spaapen RM, Zha Y, et al. Up-regulation of PD-L1, IDO, and T(regs) in the melanoma tumor micro-environment is driven by CD8(+) T cells. Sci Transl Med 2013;5:200ra116. [Crossref] [PubMed]
- Abiko K, Matsumura N, Hamanishi J, et al. IFN-gamma from lymphocytes induces PD-L1 expression and promotes progression of ovarian cancer. Br J Cancer 2015;112:1501-9. [Crossref] [PubMed]
- Cheng H, Liu P, Zhang F, et al. A genetic mouse model of invasive endometrial cancer driven by concurrent loss of Pten and Lkb1 is highly responsive to mTOR inhibition. Cancer Res 2014;74:15-23. [Crossref] [PubMed]
- Atsaves V, Tsesmetzis N, Chioureas D, et al. PD-L1 is commonly expressed and transcriptionally regulated by STAT3 and MYC in ALK-negative anaplastic large-cell lymphoma. Leukemia 2017;31:1633-7. [Crossref] [PubMed]
- Coelho MA, de Carne Trecesson S, Rana S, et al. Oncogenic RAS Signaling Promotes Tumor Immunoresistance by Stabilizing PD-L1 mRNA. Immunity 2017;47:1083-1099.e6. [Crossref] [PubMed]
- Chen N, Fang W, Zhan J, et al. Upregulation of PD-L1 by EGFR Activation Mediates the Immune Escape in EGFR-Driven NSCLC: Implication for Optional Immune Targeted Therapy for NSCLC Patients with EGFR Mutation. J Thorac Oncol 2015;10:910-23. [Crossref] [PubMed]
- Dong ZY, Zhong WZ, Zhang XC, et al. Potential Predictive Value of TP53 and KRAS Mutation Status for Response to PD-1 Blockade Immunotherapy in Lung Adenocarcinoma. Clin Cancer Res 2017;23:3012-24. [Crossref] [PubMed]
- Baitsch L, Baumgaertner P, Devevre E, et al. Exhaustion of tumor-specific CD8(+) T cells in metastases from melanoma patients. J Clin Invest 2011;121:2350-60. [Crossref] [PubMed]
- Day CL, Kaufmann DE, Kiepiela P, et al. PD-1 expression on HIV-specific T cells is associated with T-cell exhaustion and disease progression. Nature 2006;443:350-4. [Crossref] [PubMed]
- Thommen DS, Schreiner J, Muller P, et al. Progression of Lung Cancer Is Associated with Increased Dysfunction of T Cells Defined by Coexpression of Multiple Inhibitory Receptors. Cancer Immunol Res 2015;3:1344-55. [Crossref] [PubMed]
- Wherry EJ. T cell exhaustion. Nat Immunol 2011;12:492-9. [Crossref] [PubMed]
- Daud AI, Loo K, Pauli ML, et al. Tumor immune profiling predicts response to anti-PD-1 therapy in human melanoma. J Clin Invest 2016;126:3447-52. [Crossref] [PubMed]
- Thompson ED, Zahurak M, Murphy A, et al. Patterns of PD-L1 expression and CD8 T cell infiltration in gastric adenocarcinomas and associated immune stroma. Gut 2017;66:794-801. [Crossref] [PubMed]
- Liu SY, Dong ZY, Wu SP, et al. Clinical relevance of PD-L1 expression and CD8+ T cells infiltration in patients with EGFR-mutated and ALK-rearranged lung cancer. Lung Cancer 2018;125:86-92. [Crossref] [PubMed]
- Leclerc M, Voilin E, Gros G, et al. Regulation of antitumour CD8 T-cell immunity and checkpoint blockade immunotherapy by Neuropilin-1. Nat Commun 2019;10:3345. [Crossref] [PubMed]
- Boutet M, Gauthier L, Leclerc M, et al. TGF-b signaling intersects with CD103 integrin signaling to promote T lymphocyte accumulation and antitumor activity in the lung tumor microenvironment. Cancer Res 2016;76:1757-69. [Crossref] [PubMed]