MicroRNA-377-3p targeting MMP-16 inhibits ovarian cancer cell growth, invasion, and interstitial transition
Introduction
Ovarian cancer (OC) is one of the most common cancers in women, with an incidence rate of 3–12/100,000. OC is characterized by rapid spread and high mortality rates (1-3). The development of drug resistance contributes to this to high mortality rate, which may be related to the increased expression of extracellular matrix (ECM) protein and drug transporters (4). At present, the treatment methods of OC are surgery, chemotherapy, and radiation therapy. Extensive side effects and chemoresistance are the leading causes of relapse or death in patients with epithelial OC. Therefore, efforts toward developing novel therapies and adjuvant therapies for OC treatment have been urgently applied.
In recent years, biological therapy has been widely used for cancer treatment (5,6). MicroRNAs (miRNAs) are endogenous, noncoding RNAs, with approximately 22 nucleotides. miRNAs regulate gene expression by combining with the 3' untranslated region (3'-UTR) of mRNAs to suppress translation or induction of mRNA cleavage (7). miRNAs have been deemed pivotal to the regulation of a cancer and have been proposed as minimally invasive prognostic markers for various types of cancer (8-10). To date, multiple miRNA types have been identified as participating in OC treatment. Wang et al. found that miR-193a-5p targeting HOXA7 regulation inhibited cell proliferation and induced cell apoptosis in human OC (11). Paliwal et al. revealed that miR-22 and miR-21 could be validated as good diagnostic biomarkers for the early detection of OC (12). It has further been reported that long non-coding RNA (lncRNA) MIF-AS1 could curtail the progression of OC by sponging miRNA-31-5p (13).
Matrix metalloproteinase (MMP)-16, a membrane-type metalloprotease, is associated with the proliferation, invasion and migration of cancer cells. (14,15). Atkinson et al. found that MMP-14, -15, and -17 may be good disease markers, especially as human non-small cell lung cancer (NSCLC) therapeutic targets (16). Studies have shown that MMP-16 functions by activating proMMP-2 (gelatinase A) in cell development of some diseases (17). Sakata et al. reported that MT1-MMP does not exist in normal ovarian surface epithelium or benign ovarian tumors, but it is widely expressed in all histological types of OC (18). These results suggest that MT1-MMP is a highly targeted marker of OC.
Epithelial-mesenchymal transition (EMT) is currently considered to be one of the key and initial links in the invasion and metastasis of tumor cells. During the progress of EMT, cells lose their epithelial traits, including cell adhesion and polarity, and obtain a mesenchymal morphology and the ability to migrate(19). The rapid spread of OC is closely related to the EMT of cancer cells. MiR-377 is proven to play an active anti-invasive effect in a variety of cancers. It is reported that MiR-377 could relieve proliferation and metastasis of gastric cancer cells via repressing the expression of VEGFA(20). miR-377-3p-E2F3 has been demonstrated to participate in NSCLC progression (21). However, it is not clear whether mir-377-3p is involved in the regulation of OC, and whether mir-377-3p is involved in the regulation of OC by targeting MMP16.
In present study, we used the SKOV3 cell line as a model to explore the effect of miRNA-377-3p overexpression on OC in vitro. The data suggested that the overexpression of miRNA-377-3p suppressed cell proliferation, invasion, and induced apoptosis. The prediction results of TargetScan software indicated that miRNA-377-3p can bind to the 3'-UTR region of MMP-16 directly. Interestingly, the colony formation rate of the co-transfection of miRNA-377-3p with MMP-16 was reduced significantly compared with that of MMP-16 transfection alone. Furthermore, the results of experiments in vivo were consistent with those of experiments in vitro. The results therefore provide evidence for miRNA-377-3p inhibiting OC cell growth, invasion, and interstitial transition via targeting MMP-16. The overexpression of miRNA-377-3p could thus be a novel adjuvant against OC.
We present the following article in accordance with the ARRIVE reporting checklist (available at http://dx.doi.org/10.21037/atm-20-8027).
Methods
Organize collection
A collection of 60 pairs of OC patients diagnosed pathologically in our hospital from January 2017 to December 2019 were surgically removed cancerous tissues and paracancerous tissues, ranging in age from 30-78 years old, with a median age of 57 years old. All patients did not receive radiotherapy, chemotherapy and other adjuvant treatments before surgery. This study was approved by the Medical Ethics Committee of our hospital, and all research subjects signed an informed consent form. All procedures performed in this study involving human participants were in accordance with the Declaration of Helsinki (as revised in 2013).
Cell culture
SKOV-3 cell line was obtained from the American Type Culture Collection (ATCC). According to the supplier’s instructions, cells were cultivated in RPMI 1640 medium (Gibco, Rockville, MD, USA) in T75 cell culture flasks (Corning, New York, NY, USA).
Cell transfection
miR-377 mimic and pcDNA-MMP-16 were synthesized by Molbase Biological Reagent Company (Shanghai, China). Cells were seeded in 6-well plates and cultured to a density of 60%; then, the miR-377-3p mimics were added into the plates together with Lipofectamine 2000 (Invitrogen, Carlsbad, CA, USA) according to the manufacturer’s instructions. After 24-incubation, the transfection efficiency was confirmed using quantitative reverse transcription-polymerase chain reaction (qRT-PCR). For MMP-16 overexpression, cells were cultured for 24 h in a 6-well plate, and then incubated with plasmid cloning DNA (pcDNA)3.1-MMP-16 (Molbase, Shanghai, China) using Lipofectamine 2000 in RPMI 1640. After 48 h-incubation in a normal culture atmosphere, the level of MMP-16 was detected using RT-qPCR and Western blot (WB).
5-ethynyl-2'-deoxyuridine (EdU) staining for cell proliferation
SKOV3 cells were resuspended in RPMI 1640 medium with 10% fetal bovine serum (FBS), incubated with 10 µM EdU for 2 h, and then the cells were transfected and cultured for 72 h. After trypsin digestion, cells were collected according to standard procedures: centrifugation, washing, fixation, permeabilization, and incubation of the reaction solution. Cell proliferation was detected by FC 500 Flow Cytometer MCL, according to the manufacturer’s instructions (Beckman Coulter, Brea, CA, USA), and the proliferation ability was estimated by the percentage of EdU-positive cells.
Transwell assay
Matrigel diluted with serum-free medium was applied to the middle bottom of the upper chamber. The upper chamber was air-dried at room temperature for later use. After the upper chamber was incubated with serum-free medium for 24 hours, cell suspension (200 mL per chamber) was added. Then, 600 µL of medium containing 10% FBS was added to the lower chamber. After the chamber was incubated at 37 °C for 24 hours, the chamber was removed and washed twice with phosphate-buffered saline (PBS). Residual cells were removed with cotton swabs, and crystal violet staining was performed after fixation with 95% alcohol. Next, the cells were observed with a microscope (Leica, Germany). Each group selects 5 visual fields to record the number of invading cells, and calculate the average number of invasive cells.
Microtube formation assay
SKOV-3 cells respectively transfected with miRNA-377 mimic, MMP-16, co-transfected miRNA-377 mimic, MMP-16, and no-transfection controls were incubated for 48 h. The serum-free RPMI medium and Matrigel were mixed at a ratio of 1:1 and precooled at 4 °C. The precooled mixture was added to the bottom of the 24-well plate in amounts of 300 µL per well, and solidified in an incubator containing 5% CO2 at 37 °C for 30 min. The above 24-well plate was seeded with SKOV-3 cells at a concentration of 1.2×105/mL. The microtubule structure was observed every 4 h. The image was analyzed using Microvision Saisam software. Each group selects 5 fields of view to record the number of microtubule structures, and calculate the average number of microtubule structures.
RT-PCR
The total RNA in tissues and cells was extracted using TRIzol™ kit (Thermo Fisher Scientific, USA) according to the manufacturer’s instructions. After the total RNA was quantified, 0.5 µg of RNA from each sample was used to synthesize cDNA using a cDNA synthesis kit (Thermo Fisher Scientific). The expression levels of miRNA-377 mimics and MMP-16 were measured by SYBR PCR Master Mix kit (Thermo Fisher Scientific), and the expression of individual genes was normalized according to the 2−ΔΔCt method. This experiment used GAPDH as an internal reference.
Primer sequences were listed as follows: miR-377 forward primer: 5'TGCTGATCACACAAAGGCAACTTTTGTGTT3', and reverse primer: 5'TGTAGTGTGTTTCCGTTGAAAACACAAAACCGA3'; MMP-16 forward primer: 5'TGTGTTGTCCAGAGGCAATG3'; and reverse, 5'ATCACTAGGCCAGCTGGTTG3'; U6 forward primer: 5'CTCGCTTCGGCAGCACA3'; and reverse primer: 5'AACGCTTCACGAATTTGCGT3'.
Luciferase reporter assay
According to the prediction result of TargetScan software, MMP-16 was found to be one of the targets of miR-377-3p. The corresponding MUT fragment of MMP-16 (MMP-16-MUT) and the WT fragment of MMP-16 (MMP-16-WT) containing potential binding sites were inserted into the pMIR-reporter plasmid Vector (Invitrogen, USA) to produce an MMP-16 luciferase reporter gene construct. According to the manufacturer’s instructions, the vector and miR-377-3p mimic were co-transfected into SKOV3 cells using Lipofectamine 2000 reagent (Invitrogen). After 24 hours of transfection, the cells were detected with a luciferase detection kit (Promega, USA), and the Renilla luciferase/ luciferase ratio (Rluc/Luc) was recorded.
Tumor formation assay
Four-week-old immunodeficient BABL/c female nude mice (150-180 g) were used to form the xenograft model. All mice remained free of specific pathogens, and 1×106 SKOV3 cells transfected with miR-377-3p mimic or NC mimic were injected subcutaneously into BABL/c nude mice to finish tumor formation analysis (n=9). Every 5 days, the tumor volume was measured. After 30 days of modeling, the animals were sacrificed and dissected, and the xenograft tumors were weighed. The collected tumor tissues were then subjected to immunohistochemistry (IHC) and RT-PCR detection. Experiments were performed under a project license (NO.: XMXK2018-0021) granted by institutional ethics board of Sichuan Provincial People’s Hospital, in compliance with Sichuan Provincial People’s Hospital institutional guidelines for the care and use of animals.
IHC
Paraffin sections were first separated in xylene and then rehydrated in gradient ethanol. The antigen was extracted with 10 mM citrate buffer, and the tissue sections were incubated in 3% H2O2 for 10 min and then sealed at room temperature for 1 h. The tissue sections were incubated overnight with Ki67 and vascular endothelial growth factor (VEGF), respectively. Then, the sections were washed with tris-buffered saline with Tween20 (TBST) and incubated with SignalStain® Boost IHC detection reagent [Cell Signaling Technology (CST) Danvers, MA, USA] at room temperature for 30 minutes. After that, the sections were stained with SignalStain SignalStain®Boost DAB substrate kit (CST) and observed under a microscope.
Western Blot analysis
RIPA lysis buffer was used to lyse the SKOV3 cells. After the cell lysate was incubated on ice for 15 min, it was centrifuged at 12,000 rpm for 20 min. A bicinchoninic acid (BCA) kit (Thermo Fisher Scientific) was used to assess the protein level. First, 10 µg of protein sample was taken for SDS-PAGE, and then transferred to a polyvinylidene fluoride (PVDF) membrane. The membrane was sealed with 5% skimmed milk at room temperature for 1 h, and then incubated with the corresponding primary antibody and anti-β-actin at 4 °C overnight. Primary antibody: PCNA (ab29, 1:1,000), MMP-16 (ab73877, 1:1,000), VEGF (ab32152, 1:250), N-cadherin (ab76011, 1:5,000), E-cadherin (ab76055, 1:1,000), Ki67 (ab92742, 1:5,000), Survivin (ab134170, 1:1,000), β-actin was regarded as a standard control. After the membrane was washed thoroughly, it was incubated with horseradish peroxidase (HRP)-conjugated goat anti-rabbit antibody at room temperature for 1 h. Finally, the blotting membrane was enhanced with enhanced chemiluminescence (ECL) reagent (Thermo Fisher Scientific).
Statistical analysis
Statistical analysis was performed using SPSS 21.0 (IBM Corp., Armonk, NY, USA). Data are expressed as mean ± SD. Multiple sets of data were analyzed by one-way ANOVA followed by Bonferroni post-hoc test. Differences were considered statistically significant with a P value <0.05.
Results
miR-377 targeted MMP-16
From Figure 1A and B, miRNA-377 is low expressed in ovarian cancer tissues and cells. From Figure 1C and D, MMP-16 is highly expressed in ovarian cancer tissues and cells. Targetscan software predicted that miR-377 could bind to MMP-16 (Figure 1E). In the miR-377 mimic group, miR-377 expression was significantly enhanced compared with the control group (Figure 1F). Meanwhile, expression of MMP-16 was downregulated after miR-377 mimic transfection (Figure 1G). The results of dual luciferase reporter gene detection showed that miR-377 mimics significantly reduced the luciferase activity of MMP-16-WT in SKOV3 cells (Figure 1H). However, miR-377 mimics had no effect on the luciferase activity of MMP-16-MUT in SKOV3 cells. The results indicated that miR-377 and MMP-16 had a good targeting relationship.
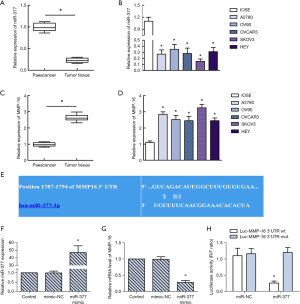
miR-377 decreased MMP-16 expression in SKOV3 cells
RT-PCR revealed that MMP-16 expression in SKOV3 cells were upregulated significantly after transfection of MMP-16 (Figure 2A). WB analysis confirmed this result and revealed that co-transfection with miR-377 mimic and MMP-16 significantly downregulated MMP-16 expression in SKOV3 cells (Figure 2B,C). The trend line analysis further confirmed that miR-377 could decrease MMP-16 expression in SKOV3 cells (Figure 2D).
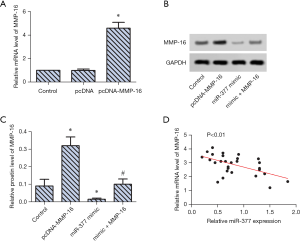
miR-377 inhibited proliferation of SKOV3 cells via targeting MMP-16
To explore the effect of MMP-16 on cell proliferation in OC, miR-377 overexpression, MMP-16 overexpression, and co-transfection with miR-377 and MMP-16 were respectively conducted. The percentage of EdU-positive cells decreased in the miR-377 overexpression group and increased markedly in the MMP-16 overexpression group compared with the control group, while it was decreased in the miR-377 and MMP-16 co-transfection group (Figure 3A). Compared with the control group, the number of microtubules formed decreased in the miR-377 overexpression group and increased markedly in the MMP-16 overexpression group compared with the control group, while it was decreased in the miR-377 and MMP-16 co-transfection group (Figure 3B). RT-PCR and WB analysis confirmed that the expression of survivin and Ki67 was obviously upregulated in the MMP-16 overexpression group and downregulated significantly in the miR-377 and MMP-16 co-transfection group (Figure 3C,D). These findings revealed that miR-377 inhibited proliferation of SKOV3 cells via targeting MMP-16.
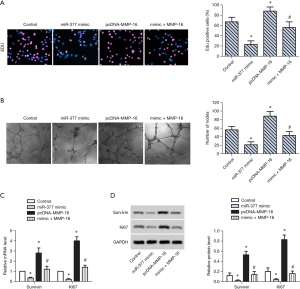
miR-377 inhibited invasion of SKOV3 cells via targeting MMP-16
To further explore whether MMP-16 is associated with invasion and interstitial transition of SKOV3 cells, Transwell assay and WB analysis were conducted. The number of invasive cells per field was significantly increased in the MMP-16 overexpression group compared with the control group (Figure 4A). Of note, miR-377 and MMP-16 co-transfection decreased the number of invasive cells per field. WB analysis showed that the expression of microtubule formation-associated proteins, VEGF and N-cadherin, were enhanced significantly the in MMP-16 overexpression group and decreased significantly in the co-transfection group (Figure 4B). Inversely, expression of E-cadherin was downregulated in the MMP-16 overexpression group while being upregulated significantly in the co-transfection group (Figure 4B). These results suggested that miR-377 inhibited the invasive and interstitial transition of SKOV3 cells via targeting MMP-16.
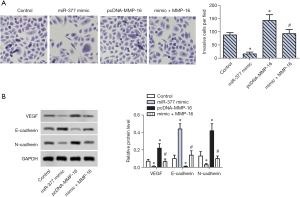
miR-377 suppressed tumor formation in vivo
To clarify the effect of miR-377 on tumor formation in vivo, an in vivo tumor transplant model was established. Tumor size, tumor volume, and tumor weight were all reduced in the miR-377 mimic-transfected group compared with the NC mimic-transfected group (Figure 5A,B,C). RT-PCR assay showed that MMP-16 expression was downregulated significantly in the miR-377 mimic-transfected group compared with the NC mimic-transfected group. Meanwhile, miR-377 expression exhibited an opposing trend (Figure 5D). IHC results showed that the contents of Ki67 and VEGF were decreased in the miR-377 mimic-transfected group compared with NC mimic-transfected group. It is worth nothing that these results are consistent with those of the in vitro experiments. These findings indicated that miR-377 had a negative effect on the tumor formation of OC in vivo. The potential mechanism may be related to the negative regulation of miR-377 on MPP16.
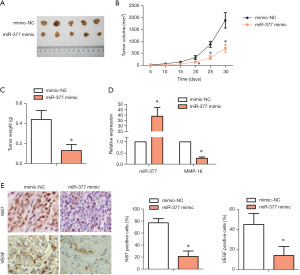
Discussion
As the sixth most common cancer in women globally, OC is regarded as the most dangerous gynecologic malignancy in women in modern society (22). In recent years, biological therapies have been implemented in the treatment of OC, and include miRNAs (23) and lncRNA (24). However, the advances in the detection and therapeutics of OC are still limited. The major obstacle in miRNA application for cancer treatment is the identification of functional targets for miRNAs, but significant progress is being made in the miRNA target search. In the present study, we discovered that miRNA-377-3p inhibited OC cell proliferation, invasion, and interstitial transition, with the possible target being MMP-16.
Membrane-type matrix metalloproteinases (MT-MMP) are pericellular ECM-degrading proteases with substrate specificities for fibrin, fibrillar collagens, as and basement membrane components (25,26). According to reports, MMPs are involved in the development and pathological process of multiple diseases, such as tumor cell invasion, rheumatoid arthritis angiogenesis, and bone resorption (27-29). MMP2 is deemed to be one of the most significant MMP associated with cell migration and tissue remodeling (30,31). It has been reported that MMP16 overexpression is present in the most aggressive nodular melanoma subtype, which grows as adhesive nodules within the dermis that metastasize early into lymph nodes (32,33). Chen et al. reported that upregulated MMP16 expression significantly promoted the invasive and migratory capability of tumor cells, but the high expression of miRNA-145 could suppress osteosarcoma metastasis via targeting MMP16 (34). The results of our study are consistent with those reported above. In the present study, the elevated expression of MMP16 significantly upregulated the expression of proliferation-related proteins, survivin and Ki67; conversely, VEGF and N-cadherin in SKOV3 cells downregulated E-cadherin expression. These results indicated that MMP16 expression promoted the proliferation and invasion of tumor cells. Notably, we found that co-transfection of miR-377 mimic and MMP-16 downregulated MMP-16 expression in SKOV3 cells and reversed abnormal protein proliferation induced by MMP16. The result of luciferase reporter assay indicated that MMP-16 is an obvious target of miR-377.
Studies have shown that miR-377 participates in the development and progression of many tumors via different genes. For instance, miRNA-377 was found to restrain the progression of hepatocellular carcinoma by inhibiting T-cell lymphoma invasion and metastasis (TIAM1) or Iroquois homeobox protein 3 (IRX3) (35,36). Multiple studies have shown that miRNA mediates the proliferation and invasion of tumor cells. For example, miRNA-377 was shown to suppress the proliferation and invasion of human glioblastoma cells by directly targeting specificity protein 1 (37). Meanwhile, Ye et al. discovered that miRNA-377 suppressed cell proliferation and invasion of cervical cancer via targeting zinc finger e-box-binding homeobox 2 (38). Similarly, our results are consistent with the above findings. We found that miRNA-377-3p mimic transfection downregulated the expression of survivin, Ki67, VEGF, and N-cadherin in SKOV3 cells; importantly, the expression of survivin, Ki67, VEGF, and N-cadherin were downregulated after co-transfection with MMP-16 and miRNA-377-3p. We also found that E-cadherin expression was downregulated in the MMP-16–transfected group, but upregulated in the co-transfection group. These results indicated that miRNA-377-3p suppressed the proliferation and invasion of SKOV3 cells, with the functional target being MMP-16.
The prerequisite for tumor cell invasion and metastasis is epithelial–mesenchymal transition (EMT) (39). In the process of EMT, cells lose their polarity, the adhesion force weakens, and the mesenchymal morphology and migration ability develop. E-cadherin and N-cadherin are the typical proteins of interstitial transition (40). In the present study, N-cadherin expression was enhanced significantly in the MMP-16 overexpression group while E-cadherin was decreased. Notably, the opposite results appeared in the co-transfection group. These results indicated that miR-377 inhibited interstitial transition of SKOV3 cells via targeting MMP-16. Our study is similar to previous studies, which also found that overexpression of MMP16 could promote cell invasion and migration (30). We therefore speculated that mir-377 could inhibit some of the malignant biological behaviors of OC cells by acting on MMP16; indeed, similar and corroborating results were produced in the in vivo experiment.
In conclusion, the results of our study support the targeting of MMP-16 by miRNA-377-3p as a novel therapeutic strategy for OC in vivo. We found that miR-377 inhibited the proliferation, invasion, and interstitial transition of SKOV3 cells, while suppressing tumor formation in vivo. It is worth noting that miRNA-377-3p targeted MMP-16, decreased MMP-16 expression in SKOV3 cells, and reversed the overexpression of MMP-16 in vitro and in vivo. The underlying mechanism is thus clearly associated with the inhibition of MMP-16 expression. These findings may provide a new treatment and prevention strategy for OC. However, the sample size of this study was limited, and further in-depth research is needed to verify our conclusion concerning this mechanism.
Acknowledgments
Funding: Performance Incentive Project of Chongqing Science and Technology Commission (No. cstc2018jxjl130059).
Footnote
Reporting Checklist: The authors have completed the ARRIVE reporting checklist. Available at http://dx.doi.org/10.21037/atm-20-8027
Data Sharing Statement: Available at http://dx.doi.org/10.21037/atm-20-8027
Conflicts of Interest: All authors have completed the ICMJE uniform disclosure form (available at http://dx.doi.org/10.21037/atm-20-8027). The authors have no conflicts of interest to declare.
Ethical Statement: The authors are accountable for all aspects of the work in ensuring that questions related to the accuracy or integrity of any part of the work are appropriately investigated and resolved. This study was approved by the Medical Ethics Committee of our hospital, and all research subjects signed an informed consent form. All procedures performed in this study involving human participants were in accordance with the Declaration of Helsinki (as revised in 2013). Animal experiments were performed under a project license (NO.: XMXK2018-0021) granted by institutional ethics board of Sichuan Provincial People’s Hospital, in compliance with Sichuan Provincial People’s Hospital institutional guidelines for the care and use of animals.
Open Access Statement: This is an Open Access article distributed in accordance with the Creative Commons Attribution-NonCommercial-NoDerivs 4.0 International License (CC BY-NC-ND 4.0), which permits the non-commercial replication and distribution of the article with the strict proviso that no changes or edits are made and the original work is properly cited (including links to both the formal publication through the relevant DOI and the license). See: https://creativecommons.org/licenses/by-nc-nd/4.0/.
References
- Lheureux S, Gourley C, Vergote I, et al. Epithelial ovarian cancer. Lancet 2019;393:1240-53.
- Flores I, Hevia D, Tittarelli A, et al. Dendritic Cells Loaded with Heat Shock-Conditioned Ovarian Epithelial Carcinoma Cell Lysates Elicit T Cell-Dependent Antitumor Immune Responses In Vitro. J Immunol Res 2019;2019:9631515. [Crossref] [PubMed]
- Li RH, Yue C, Wei BB, et al. Sichuan Da Xue Xue Bao Yi Xue Ban 2020;51:320-4. In vivo Study of siRNA Silencing XIAP Gene to Reverse Taxol-resistance in Human Ovarian Cancer Cells. [PubMed]
- Kazmierczak D, Jopek K, Sterzynska K, et al. The Significance of MicroRNAs Expression in Regulation of Extracellular Matrix and Other Drug Resistant Genes in Drug Resistant Ovarian Cancer Cell Lines. Int J Mol Sci 2020;21:2619. [Crossref] [PubMed]
- Ventura C, Vieira L, Silva C, et al. Functional effects of differentially expressed microRNAs in A549 cells exposed to MWCNT-7 or crocidolite. Toxicol Lett 2020;328:7-18. [Crossref] [PubMed]
- Hashemi A, Gorji-Bahri G. MicroRNA: Promising Roles in Cancer Therapy. Curr Pharm Biotechnol 2020;21:1186-203. [Crossref] [PubMed]
- Sakurai T, Kudo M. Signaling pathways governing tumor angiogenesis. Oncology 2011;81 Suppl 1:24-9. [Crossref] [PubMed]
- Carmeliet P, Jain RK. Molecular mechanisms and clinical applications of angiogenesis. Nature 2011;473:298-307. [Crossref] [PubMed]
- Zygalaki E, Tsaroucha EG, Kaklamanis L, et al. Quantitative real-time reverse transcription PCR study of the expression of vascular endothelial growth factor (VEGF) splice variants and VEGF receptors (VEGFR-1 and VEGFR-2) in non small cell lung cancer. Clin Chem 2007;53:1433-9. [Crossref] [PubMed]
- Robinson CJ, Stringer SE. The splice variants of vascular endothelial growth factor (VEGF) and their receptors. J Cell Sci 2001;114:853-65. [PubMed]
- Wang S, Diao YJ, Zhu BB. MiR-193a-5p suppresses cell proliferation and induces cell apoptosis by regulating HOXA7 in human ovarian cancer. Neoplasma 2020;67:825-33. [Crossref] [PubMed]
- Paliwal N, Vashist M, Chauhan M. Evaluation of miR-22 and miR-21 as diagnostic biomarkers in patients with epithelial ovarian cancer. 3 Biotech 2020;10:142.
- Fan Y, Wang L, Han XC, et al. LncRNA MIF-AS1 aggravates the progression of ovarian cancer by sponging miRNA-31-5p. Eur Rev Med Pharmacol Sci 2020;24:2248-55. [PubMed]
- Yao Y, Shen H, Zhou Y, et al. MicroRNA-215 suppresses the proliferation, migration and invasion of non-small cell lung carcinoma cells through the downregulation of matrix metalloproteinase-16 expression. Exp Ther Med 2018;15:3239-46. [Crossref] [PubMed]
- Wang H, Li XT, Wu C, et al. miR-132 can inhibit glioma cells invasion and migration by target MMP16 in vitro. Onco Targets Ther 2015;8:3211-8. [PubMed]
- Atkinson JM, Pennington CJ, Martin SW, et al. Membrane type matrix metalloproteinases (MMPs) show differential expression in non-small cell lung cancer (NSCLC) compared to normal lung: correlation of MMP-14 mRNA expression and proteolytic activity. Eur J Cancer 2007;43:1764-71. [Crossref] [PubMed]
- Zhang WL, Chen YF, Meng HZ, et al. Role of miR-155 in the regulation of MMP-16 expression in intervertebral disc degeneration. J Orthop Res 2017;35:1323-34. [Crossref] [PubMed]
- Sakata K, Shigemasa K, Nagai N, et al. Expression of matrix metalloproteinases (MMP-2, MMP-9, MT1-MMP) and their inhibitors (TIMP-1, TIMP-2) in common epithelial tumors of the ovary. Int J Oncol 2000;17:673-81. [Crossref] [PubMed]
- Zhang L, Song Y, Ling Z, et al. R-spondin 2-LGR4 system regulates growth, migration and invasion, epithelial-mesenchymal transition and stem-like properties of tongue squamous cell carcinoma via Wnt/β-catenin signaling. EBioMedicine 2019;44:275-88. [Crossref] [PubMed]
- Wang CQ, Chen L, Dong CL, et al. MiR-377 suppresses cell proliferation and metastasis in gastric cancer via repressing the expression of VEGFA. Eur Rev Med Pharmacol Sci 2017;21:5101-11. [PubMed]
- Sun C, Li S, Zhang F, et al. Long non-coding RNA NEAT1 promotes non-small cell lung cancer progression through regulation of miR-377-3p-E2F3 pathway. Oncotarget 2016;7:51784-814. [Crossref] [PubMed]
- Li Q, Peng Z, Wang H, et al. Sichuan Da Xue Xue Bao Yi Xue Ban 2003;34:5-8. Gene therapy for ovarian cancer by adenovirus-mediated transduction of cytosine deaminase gene in vitro. [PubMed]
- Deb B, Uddin A, Chakraborty S. miRNAs and ovarian cancer: An overview. J Cell Physiol 2018;233:3846-54. [Crossref] [PubMed]
- Yu Y, Zhang X, Tian H, et al. Knockdown of long non-coding RNA HOTAIR increases cisplatin sensitivity in ovarian cancer by inhibiting cisplatin-induced autophagy. J buon 2018;23:1396-401. [PubMed]
- Willis AL, Sabeh F, Li XY, et al. Extracellular matrix determinants and the regulation of cancer cell invasion stratagems. J Microsc 2013;251:250-60. [Crossref] [PubMed]
- Rowe RG, Weiss SJ. Navigating ECM barriers at the invasive front: the cancer cell-stroma interface. Annu Rev Cell Dev Biol 2009;25:567-95. [Crossref] [PubMed]
- Liotta LA. Tumor invasion and metastases--role of the extracellular matrix: Rhoads Memorial Award lecture. Cancer Res 1986;46:1-7. [PubMed]
- Stetler-Stevenson WG, Aznavoorian S, Liotta LA. Tumor cell interactions with the extracellular matrix during invasion and metastasis. Annu Rev Cell Biol 1993;9:541-73. [Crossref] [PubMed]
- Ouyang YW, Peng ZL, Yao XY, et al. The expression of matrix metalloproteinase-2 and -9 in cervical cancer and a study of their relationship. Sichuan Da Xue Xue Bao Yi Xue Ban 2004;35:330-3. [PubMed]
- Azzam HS, Arand G, Lippman ME, et al. Association of MMP-2 activation potential with metastatic progression in human breast cancer cell lines independent of MMP-2 production. J Natl Cancer Inst 1993;85:1758-64. [Crossref] [PubMed]
- Al-Raawi D, Abu-El-Zahab H, El-Shinawi M, et al. Membrane type-1 matrix metalloproteinase (MT1-MMP) correlates with the expression and activation of matrix metalloproteinase-2 (MMP-2) in inflammatory breast cancer. Int J Clin Exp Med 2011;4:265-75. [PubMed]
- Jaeger J, Koczan D, Thiesen HJ, et al. Gene expression signatures for tumor progression, tumor subtype, and tumor thickness in laser-microdissected melanoma tissues. Clin Cancer Res 2007;13:806-15. [Crossref] [PubMed]
- Poetsch M, Dittberner T, Woenckhaus C. Can different genetic changes characterize histogenetic subtypes and biologic behavior in sporadic malignant melanoma of the skin? Cell Mol Life Sci 2003;60:1923-32. [Crossref] [PubMed]
- Chen B, Huang Z, Zhang Y, et al. MicroRNA-145 Suppresses Osteosarcoma Metastasis via Targeting MMP16. Cell Physiol Biochem 2015;37:2183-93. [Crossref] [PubMed]
- Chen G, Lu L, Liu C, et al. MicroRNA-377 suppresses cell proliferation and invasion by inhibiting TIAM1 expression in hepatocellular carcinoma. PLoS One 2015;10:e0117714. [Crossref] [PubMed]
- Wang P, Zhuang C, Huang D, et al. Downregulation of miR-377 contributes to IRX3 deregulation in hepatocellular carcinoma. Oncol Rep 2016;36:247-52. [Crossref] [PubMed]
- Zhang R, Luo H, Wang S, et al. MicroRNA-377 inhibited proliferation and invasion of human glioblastoma cells by directly targeting specificity protein 1. Neuro Oncol 2014;16:1510-22. [Crossref] [PubMed]
- Ye C, Hu Y, Wang J. MicroRNA-377 Targets Zinc Finger E-box-Binding Homeobox 2 to Inhibit Cell Proliferation and Invasion of Cervical Cancer. Oncol Res 2019;27:183-92. [Crossref] [PubMed]
- Das V, Bhattacharya S, Chikkaputtaiah C, et al. The basics of epithelial-mesenchymal transition (EMT): A study from a structure, dynamics, and functional perspective. J Cell Physiol 2019. Epub ahead of print. [Crossref] [PubMed]
- Micalizzi DS, Farabaugh SM, Ford HL. Epithelial-mesenchymal transition in cancer: parallels between normal development and tumor progression. J Mammary Gland Biol Neoplasia 2010;15:117-34. [Crossref] [PubMed]
(English Language Editor: J. Gray)