Rosavin suppresses osteoclastogenesis in vivo and in vitro by blocking the nuclear factor kappa-light-chain-enhancer of activated B cells (NF-κB) and mitogen-activated protein kinase (MAPK) signaling pathways
Introduction
In bone remodeling, the homeostasis between osteoblast-mediated bone formation and osteoclast-mediated bone resorption is essential (1). The imbalance of bone homeostasis due to excessive osteoclastogenesis or reduced osteogenesis can result in various disorders, such as postmenopausal osteoporosis (PMOP), rheumatoid arthritis, and Paget’s disease (2-4). Promoting bone formation and inhibiting bone resorption are effective treatment strategies for bone loss diseases (5,6).
Osteoporosis is the most common bone loss disease, and is accompanied by an obvious decrease in bone density and lesions in bone tissue microstructure (7). The incidence of osteoporosis is often associated with age and sex (8). In recent years, there has been a dramatic increase in PMOP, with patients over 60 years old experiencing distinctly increased morbidity (9). Estrogen deficiency plays an important role in PMOP. During the postmenopausal period, various inflammation inhibitors decrease, and the expression of receptor activator of nuclear factor-κB ligand (RANKL) increases significantly, resulting in excessive activation of osteoclasts and the promotion of bone resorption (10). As a result, the suppression of osteoclastogenesis remains a potential therapy for osteoclast-related bone diseases, such as osteoporosis.
Osteoclasts differentiate from monocyte-macrophages under the induction of macrophage colony-stimulating factor (M-CSF) and RANKL (11-13). RANKL binds to receptor activator of nuclear factor-κB (RANK), which then recruits the tumor necrosis factor receptor-associated factors (TRAFs), activating a variety of downstream signaling pathways, such as the mitogen-activated protein kinase (MAPK) and nuclear factor kappa-light-chain-enhancer of activated B cells (NF-κB) pathways (14). Nuclear factor of activated T-cell cytoplasmic 1 (NFATc1) has been reported to be an essential transcriptional factor in osteoclast differentiation and is necessary for the expression of osteoclast-associated genes, such as cathepsin K, matrix metalloproteinase (MMP)-9, calcitonin receptor (CTR), and tartrate-resistant acid phosphatase (TRAP) (15,16). Targeting RANKL-mediated signaling to decrease the expression of NFATc1 is a potential treatment strategy for osteoclastogenesis-related disorders, including osteoporosis (17,18).
Natural medicines are important in clinical treatment (19). Rhodiola Rosea L is a traditional Chinese herb used for inflammation and pulmonary fibrosis, as well as for its antioxidant properties (20,21). Rosavin is one of the most important active alkylbenzene diglycoside compound ingredients of Rhodiola Rosea L, and has proven efficacy in nootropic, anti-depressant, anti-cancer, and antioxidative treatment (22-24). In the present study, we explored the role of rosavin on ovariectomy-induced osteoporosis in mice, which is an effective and widely used model to simulate PMOP, as well as the effects of rosavin on the differentiation and function of osteoclasts in vitro. The molecular mechanisms involved in this process were also investigated. We present the following article in accordance with the ARRIVE (Animal Research: Reporting of In Vivo Experiments) reporting checklist (available at http://dx.doi.org/10.21037/atm-20-4255).
Methods
All procedures were approved by and performed in accordance with the ethical standards of the Ethics Committee of Shanghai Ninth People’s Hospital (No. SH9H-2019-T159-2), and were in compliance with the NIH Guide for the Care and Use of Laboratory Animals [2018].
Reagents
Rosavin was obtained from Nuodande Standard Technical Services (Shanghai, China). Reagents were diluted in phosphate-buffered saline (PBS) solution. Fetal bovine serum (FBS), antibodies, streptomycin, and penicillin were purchased from BioTNT (Shanghai, China).
Cell proliferation analysis
The MTT assay was conducted according to the manufacturer’s instructions (Sigma-Aldrich, St. Louis, USA). Cells were placed into 96-well plates (1×104 cells/well). The doses of rosavin were as follows: 2.5, 5, 10, 20, and 40 µM. Bone marrow monocyte cells (BMMCs), isolated from C57BL/6 mice, and RAW264.7 cells were cultured. After 72 hours, 50 µg/µL of MTT solution was added, and the absorbance of the plate was detected at 490 nm using a linked immunosorbent assay plate reader (Olympus, Tokyo, Japan). The experiments were independently performed three times.
In vitro osteoclastogenesis assay
BMMCs were washed from the femurs of C57 mice. Cells were cultured in minimum essential medium α (α-MEM) containing 1% penicillin, 1% streptomycin, and 10% FBS. Cells were seeded into 96-well plates containing M-CSF (30 ng/mL) and RANKL (100 ng/mL), and were incubated with different concentrations of rosavin (0, 1.25, 2.5, and 5 µM). After 7 days, TRAP staining was conducted to detect the TRAP-positive cells (Sigma-Aldrich, St, Louis, USA). The experiments were independently performed three times, and the data were analyzed by one-way analysis of variance (ANOVA).
F-actin ring formation and pit formation assay
For the F-actin formation assay, cells were initially fixed with 4% paraformaldehyde for 1 hour and then washed twice with PBS. Next, the cells were conducted with fluorescein isothiocyanate (FITC)-phalloidin for 2 hours and stained with 4’6-diamidino-2-phenylindole for 20 minutes.
For the pit formation assay, osteoclasts were cultured on a bone biomimetic synthetic plate (OsteoAssay Surface plates; Corning, NY, USA), and were induced with 30 ng/mL of M-CSF and 100 ng/mL of RANKL. The area resorbed by the osteoclasts was detected using a light microscope (Lecia CTR400, Germany). The experiments were independently performed three times, and the data were analyzed by one-way ANOVA.
In vitro osteogenesis assay
C57BL/6 mice were sacrificed via pentobarbital sodium overdose. Bone marrow mesenchymal stem cells (BMSCs) were obtained by washing the femurs of the mice. BMSCs were maintained in a complete medium supplemented with 1% streptomycin and penicillin, 20 mM β-glycerophosphate, 10 nM dexamethasone, and 100 µg/mL ascorbic acid. After 20 days, alkaline phosphatase (ALP) staining and alizarin red staining were performed to detect the osteoblasts. The experiments were independently performed three times.
Polymerase chain reaction (PCR) analysis
Real-time quantitative reverse transcription polymerase chain reaction (qRT-PCR) was used to detect the transcription of osteogenesis and osteoclastogenesis-related genes. Briefly, whole-cell ribonucleic acid (RNA) samples were extracted using TRIzol reagent, as previously reported, and complementary deoxyribonucleic acid (DNA) was generated using a reverse transcriptase kit (25). The TB Green premix Ex Taq kit (Takara, CA, USA) was used to detect gene expression. Mouse-specific PCR primers were used. Mouse runt-related transcription factor 2 (Runx2), forward: 5'-GACTGTGGTTACCGTCATGGC-3', reverse: 5'-ACTTGGTTTTTCATAACAGCGGA-3'; mouse osteocalcin (OCN), forward: 5'-GGACCATCTTTCTGCTCACTCTGC-3', reverse: 5'-TGTTCACTACCTTATTGCCCTCCTG-3'; mouse NFATc1, forward: 5'-ATGACGGGGCTGGAGCAGGA-3', reverse: 5'-TTAGGAGTGGGGGGATCGTGC-3'; mouse RANK, forward: 5'-CTGCTCCTCTTCATCTCTGTG-3', reverse: 5'- CTTCTGGAACCATCTTCTCCTC-3'; mouse colony-stimulating factor-1 receptor (c-fms), forward: 5'-TTCACTCCGGTGGTGGTGGCCTGT-3', reverse: 5'-GTTGAGTAGGTCTCCATAGCAGCA-3'. The experiments were independently performed three times, and the data were analyzed using the t-test and one-way ANOVA.
In vivo experiments
Eight-week-old female C57BL/6 mice were supplied by the Shanghai SLAC Laboratory Animal Center (SLAC, Shanghai, China). All mice were raised in a controlled environment at 25 °C under a 12-hour light/dark cycle. Sufficient food and water were available.
Eighteen mice were randomly separated into three groups; a sham-operated group, an ovariectomized treatment with vehicle group, and a rosavin treatment group, with six mice in each group. During the operation, the mice were anesthetized with 1% pentobarbital sodium (30 mg/kg). Mice underwent bilateral OVX. Small incisions were made on the dorsal skin and peritoneum. Two ovaries and part of the oviduct were removed and pressed to stop any bleeding. The incision on the skin was closed with 5-0 nonabsorbable suture lines. After the procedure, mice were allowed to recover for 24 h. The mice in the OVX (ovariectomy) + vehicle group and the OVX + rosavin group had both ovaries resected, while the mice in the sham-operated group received surgery to expose the ovaries without resection. Rosavin treatment group mice were intraperitoneally injected with rosavin (10 mg/kg) or PBS every day. After 6 weeks, the mice were sacrificed via pentobarbital sodium overdose (150 mg/kg).
Immunofluorescence staining
The effects of rosavin (5 µM) on p65 nuclear translocation were examined in RAW264.7 cell lines. Immunofluorescence was conducted as described previously (26). Cells were fixed with 4% paraformaldehyde for 20 minutes, and were then washed with Triton X reagents and blocked with 5% bovine serum albumin (BSA) for 20 minutes. Next, anti-p65 antibody, anti-mouse immunoglobulin G (IgG) antibody, and fluorescein-conjugated streptavidin were added to the cells. The experiments were independently performed three times.
Western blotting
RAW264.7 cells cultured with RANKL or RANKL & rosavin (5 µM) for different durations were prepared for western blotting to determine the expression of osteoclastogenesis-related proteins, including phosphorylated (p)-p65, p-inhibitory subunit of NF-κB (IκB), p-extracellular-signal-regulated kinase (ERK), p-c-Jun N-terminal kinase (JNK), and p-p38. The samples were probed overnight with primary antibodies against β-actin (1:2,000, GB12001, Servicebio, China), TRAP (1:1,000, ab191406, abcam, USA), Cathepsin K (1:1,000, ab19027, abcam, USA), MMP-9 (1:1,000, ab219372, abcam, USA), CTR (1:1,000, ab114596, abcam, USA), TRAF6 (1:500, 13411-1-AP, proteintech, USA), p-p65 (1:10,000, ab76302, abcam, USA), p65 (1:1,000, ab32536, abcam, USA), IκBα (1:10,000, ab133462, abcam, USA), p-ERK (1:1,000, ab201015, abcam, USA), ERK (1:1,000, GB12087, Servicebio, USA), p-JNK (1:1,000, ab124956, abcam, USA), JNK (1:1,000, ab179461, abcam, USA), p-p38 (1:1,000, ab195049, abcam, USA), and p38 (1:1,000, ab31828, abcam, USA). The membranes were subsequently incubated with secondary antibodies of anti-rabbit IgG (1:2,000, ab6721, abcam, USA). The experiments were independently performed three times, and the data were analyzed using the t-test.
Bone histomorphometric analysis
The number of osteoclasts and amount of trabecular bone were evaluated by bone histomorphometric analyses as previously described. Mouse femurs were fixed and decalcified for at least 4 weeks. The 3-µm-thick bone sections were then stained with hematoxylin and eosin (H&E) and TRAP.
Micro-computed tomography (CT) analysis
We scanned 200 section planes below the growth plate of the mouse femurs (using Skyscan1172, Antwerp, Belgium). Built-in software was used to detect the trabecular bone and metaphyseal region.
Enzyme-linked immunosorbent assay
All mice were sacrificed with pentobarbital sodium, and the arterial blood of the mice was collected. Next, the serum was centrifuged at 3,000 rpm for 10 minutes. The expression of OCN, Cross Linked C-telopeptide of Type |Collagen (CTX-1), tartrate-resistant acid phosphatase 5b (TRAcp5b), and bone alkaline phosphatase (BALP) was detected using enzyme-linked immunosorbent assay (ELISA) kits according to the manufacturer’s instructions. The experiments were independently performed three times, and the data were analyzed by one-way ANOVA.
Statistical analysis
All data were expressed as the mean ± standard deviation (SD). Two independent group comparisons were conducted using the Student’s t-test. Comparisons among more than three groups were analyzed by one-way analysis ANOVA via the Statistical Product and Service Solutions (SPSS) statistical software. A P value <0.05 was regarded as being statistically significant.
Results
Rosavin suppressed osteoclastogenesis
To perform the in vitro experiments, two standard osteoclastogenesis cellular models, BMMCs and RAW264.7 cells, were used for an MTT assay. The results indicated that rosavin showed no cytotoxicity below 5 µM (Figure 1A). We then examined the role of rosavin in the differentiation of osteoclasts. Cells were induced with RANKL and M-CSF and treated with 1.25, 2.5, or 5 µM rosavin. Seven days later, the number of TRAP-positive cells was calculated. As shown in Figure 1B,C, compared to the control group, the TRAP-positive cells decreased significantly after treatment with rosavin (*, P<0.05, **, P<0.01), indicating that rosavin suppressed osteoclast differentiation in a concentration-dependent manner.
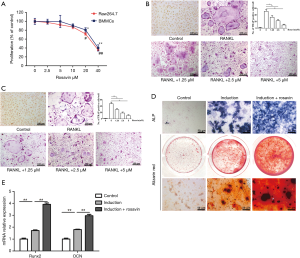
Rosavin promoted osteogenesis in vitro
To explore the effects of rosavin on osteogenesis, ALP staining and alizarin red staining were performed. As shown in Figure 1D, alizarin red- and ALP-positive cells increased significantly with rosavin treatment, indicating that rosavin promoted osteogenic differentiation. In addition, qRT-PCR revealed that the expression levels of Runx2 and OCN, which are related to osteogenesis, were significantly increased after treatment with rosavin (Figure 1E, **, P<0.01). These results demonstrated that rosavin promoted osteogenesis of BMSCs in vitro.
Rosavin inhibited the function of osteoclasts in vitro
To explore the effects of rosavin on the function of osteoclasts, we performed actin ring formation experiments. BMMCs were incubated with RANKL and M-CSF with 1.25, 2.5, or 5 µM rosavin. The fluorescein isothiocyanate (FITC)-phalloidin staining results indicated that the number and size of the F-actin rings decreased in a concentration-dependent manner (Figure 2A, **, P<0.01). The results demonstrated that rosavin suppressed the formation of F-actin rings during the process of osteoclastogenesis.
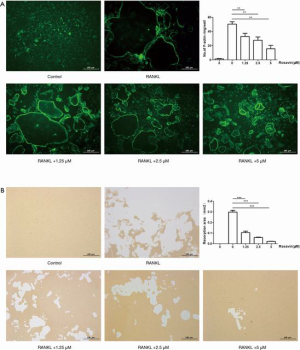
Bone resorption experiments were also conducted to identify the role of rosavin on the function of osteoclasts. The results showed that clear pits had formed on the bone biomimetic synthetic surface with RANKL and M-CSF induction. However, after the administration of rosavin, the resorbed area reduced markedly (Figure 2B, ***, P<0.001).
Rosavin inhibited osteoclastogenesis at an early stage
To investigate the influence of rosavin on the process of osteoclastogenesis, we treated BMMCs and RAW264.7 cells with rosavin (5 µM) on day 1, 3, or 5 after induction with M-CSF and RANKL. TRAP staining results showed that rosavin mainly suppressed osteoclast formation on the first day (**, P<0.01). However, when administered on day 3 for RAW 264.7 cells and day 5 for BMMCs, rosavin did not inhibit osteoclast formation (Figure 3A,B). Western blotting demonstrated that the expression levels of osteoclastogenesis-related genes, including TRAP, cathepsin K, MMP-9, CTR, and TRAF6, decreased considerably after administration of rosavin on day 1; however, the inhibitory effects decreased when rosavin was administered on days 3 and 5 (Figure 3C, **, P<0.01). NFATc1 plays an essential role in osteoclastogenesis and is necessary for the expression of osteoclast-associated factors (27). As shown in Figure 3D, the PCR results of NAFTc1 were consistent with those of western blotting of RAW264.7 cells (**, P<0.01). We also performed qRT-PCR to detect the expression of RANK and c-fms, and the results demonstrated that rosavin did not affect c-fms or RANK expression induced by M-CSF (Figure 3E). These findings indicated that rosavin suppressed osteoclast differentiation at an early stage.
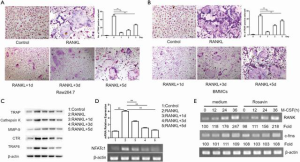
Rosavin suppressed the NF-κB pathway in osteoclastogenesis
RANKL-mediated NF-κB signaling is important for osteoclastogenesis (28). We conducted immunofluorescence staining to investigate the localization of p65 treated with rosavin. The nuclear translocation of p65 was significantly suppressed by rosavin after RANKL induction (Figure 4A). After stimulation of M-CSF and RANKL in RAW264.7 cells, p65 was phosphorylated and subsequently translocated into the nucleus. However, treatment with 5 µM rosavin markedly decreased nuclear translocation. Western blotting indicated that rosavin treatment could inhibit the RANKL-induced phosphorylation of p65 and IκBα (Figure 4B). The above results indicated that rosavin inhibited NF-κB signaling induced by RANKL stimulation.
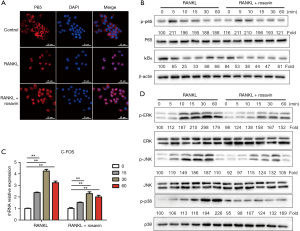
Rosavin suppressed the activation of the MAPK pathway
Next, we investigated the role of rosavin in MAPK signaling. It has been reported that RANKL-induced expression of NFATc1 is closely associated with Proto-oncogene c-fos (c-Fos), and that c-Fos is activated by the MAPK pathway (29). The results of qRT-PCR showed that rosavin treatment could inhibit the expression of c-Fos (Figure 4C, **, P<0.01). We also performed western blotting experiments to explore the phosphorylation of ERK, p38, and JNK, which are the major intermediates of the MAPK pathway. The results showed that ERK, p38, and JNK phosphorylation was substantially increased after the induction of RANKL and M-CSF. However, after rosavin treatment (5 µM), the activation levels of these factors decreased, indicating that rosavin treatment suppressed RANKL-induced MAPK signaling (Figure 4D).
Rosavin treatment reduced bone loss in OVX mice
To verify the impacts of rosavin in vivo, we constructed an ovariectomized mouse model to mimic postmenopausal osteoporosis (PMOP) in clinical work. All mice (six in each group) were healthy before and after the operation. The mice were intraperitoneally injected with rosavin (10 mg/kg) daily for 6 weeks. No adverse events were observed in the mice. The micro-CT results demonstrated that compared with sham group, OVX led to significant bone loss, while rosavin administration significantly preserved bone volume, with notable increases in Trabecular area (Tb.Area), Trabecular number (Tb.N), Bone volume/Total voume (BV/TV), and Bone Mineral Density (BMD) compared with the OVX group (Figure 5A,B). H&E staining showed that rosavin treatment attenuated bone loss in the distal femur (Figure 6A). In addition, we performed TRAP staining to explore the effects of rosavin on osteoclastogenesis in vivo. The results indicated that rosavin treatment reduced the amount of TRAP-positive cells on trabecula bone (Figure 6B). To investigate osteoclast and osteoblast activity, the serum expression levels of BALP, CTX-1, TRAcp5b, and OCN were determined (Figure 6C). Compared to the OVX group, the levels of CTX-1 and TRAcp5b were reduced by treatment with rosavin, indicating that osteoclastogenesis was inhibited (**, P<0.01). Also, the levels of OCN and BALP were increased after rosavin treatment (**, P<0.01), demonstrating the osteogenesis-promoting effect of rosavin. These data indicated that rosavin treatment attenuated bone loss in ovariectomized mice via promotion of osteogenesis and inhibition of osteoclastogenesis.
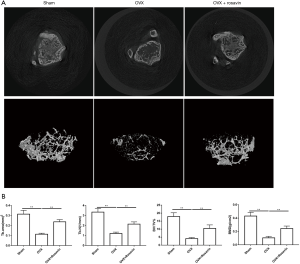
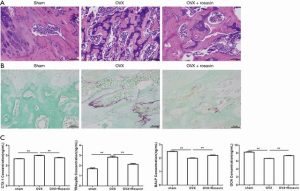
Discussion
In this study, we showed that rosavin promoted osteogenesis and suppressed osteoclast differentiation, F-actin formation, and bone resorption. For molecular mechanisms, rosavin suppressed RANKL-induced NF-κB and MAPK signaling in the early stage of osteoclastogenesis. Furthermore, we found that rosavin reduced OVX-induced bone loss in vivo.
The homeostasis between bone formation (mediated by osteoblasts) and bone resorption (mediated by osteoclasts) is important in bone metabolism (30-32). Inhibition of osteogenesis by osteoblasts or excessive osteoclastogenesis by osteoclasts can result in several disorders, such as PMOP and rheumatoid arthritis (9). Thus, there is a pressing need for research into potent agents for the treatment of osteoclast-induced disorders.
Natural products play a valuable role in clinical treatment. Rhodiola rosea L is a traditional Chinese herb used for inflammation and the prevention of pulmonary fibrosis, as well as for its antioxidant properties (21-24). Rosavin is among the most medicinal alkylbenzene diglycosides of Rhodiola Rosea L, and has been demonstrated to be effective in nootropic, anti-depressant, anti-cancer, and antioxidative treatment. Previous studies have demonstrated that disruption of the immune balance could lead to an imbalance between osteoclasts and osteoblasts (33). Therefore, we speculated that rosavin could be effective in the treatment of OVX-induced osteoporosis, which has not been reported previously.
In our research, we conducted an MTT assay before in vitro experiments to evaluate the cytotoxic effects of rosavin. We then explored the effects of rosavin on osteoclast differentiation. The results demonstrated that rosavin inhibited the formation of mature osteoclasts at an early stage of osteoclastogenesis. The following experiments showed that rosavin could promote BMSC osteogenesis in vitro.
NFATc1 is an essential regulator of osteoclast differentiation and is associated with the expression of osteoclastogenesis-related genes, including CTR, MMP-9, TRAP, TRAF6, and cathepsin K (34,35). The results of qRT-PCR and immunofluorescence staining showed that rosavin inhibited the RANKL-induced transcription of NFATc1 in RAW264.7 cells. The results of western blotting demonstrated that the expression of TRAP, TRAF6, MMP-9, CTR, and cathepsin K was inhibited by rosavin in a concentration-dependent manner. Further experiments showed that rosavin inhibited osteoclastogenesis at an early stage. Bone loss can be effectively attenuated by blocking RANKL-induced signaling to inhibit osteoclastogenesis (36).
Therefore, we performed immunofluorescence staining and western blotting to explore the potential mechanisms of action of rosavin. The results showed that rosavin blocked p65 nuclear translocation and inhibited RANKL-induced phosphorylation of p65, IκBα, ERK, p38, and JNK, indicating that rosavin inhibited osteoclastogenesis via suppression of the NF-κB and MAPK signaling pathways.
Osteoporosis, a chronic age-related disease, is typically accompanied by abundant bone loss and a higher incidence of fracture. The main pathogenic factors of this disease include excessive bone resorption and disruption of bone formation. Specifically, excessive osteoclastogenesis, which is caused by abnormal activation of RANKL signaling, has been recognized as being the key aspect in the development of osteoporosis (37). Therefore, inhibition of osteoclastogenesis is an effective therapy for osteoclast-related diseases such as osteoporosis.
Through the in vivo study, we found that rosavin reduced bone loss in the OVX mouse model. The results of H&E staining and micro-CT showed that rosavin treatment attenuated trabecular bone loss in the distal femur. Furthermore, TRAP staining indicated that rosavin treatment reduced the number of TRAP-positive cells around the bone trabecula. The serum levels of CTX-1, TRAcp5b, OCN, and BALP were determined to investigate osteoclast and osteoblast activity. Compared to the OVX group, the levels of CTX-1 and TRAcp5b were reduced after treatment with rosavin, indicating that osteoclastogenesis was inhibited. Furthermore, the levels of OCN and BALP increased after rosavin treatment, demonstrating an osteogenesis-promoting effect of rosavin. These results demonstrated that rosavin treatment attenuated bone loss in OVX mice via promotion of osteogenesis and inhibition of osteoclastogenesis.
However, there are still some limitations to this study. For instance, it did not investigate the exact drug target of rosavin, and further studies are needed to explore whether rosavin could be used to treat osteoporosis patients.
Conclusions
Via a series of in vivo and in vitro experiments, the present study demonstrated that rosavin promoted osteogenesis and suppressed RANKL-induced osteoclastogenesis. We found that rosavin inhibits osteoclastogenesis at an early stage by suppressing the NF-κB and MAPK signaling pathways. Our research indicated that rosavin could be a potentially effective therapy for osteoclast-related disorders.
Acknowledgments
Funding: This work was supported by the Key Biological and Pharmaceutical Projects of the Shanghai Science and Technology Commission [17411963900, 16411953300] and the Clinical research MDT project of Shanghai Ninth People’s Hospital, Shanghai Jiao Tong University [201701017, 201701018].
Footnote
Reporting Checklist: The authors have completed the ARRIVE (Animal Research: Reporting of In Vivo Experiments) reporting checklist. Available at http://dx.doi.org/10.21037/atm-20-4255
Peer Review File: Available at http://dx.doi.org/10.21037/atm-20-4255
Data Sharing Statement: Available at http://dx.doi.org/10.21037/atm-20-4255
Conflicts of Interest: All authors have completed the ICMJE uniform disclosure form (available at http://dx.doi.org/10.21037/atm-20-4255). The authors have no conflicts of interest to declare.
Ethical Statement: The authors are accountable for all aspects of the work in ensuring that questions related to the accuracy or integrity of any part of the work are appropriately investigated and resolved. All procedures were approved by and performed in accordance with the ethical standards of the Ethics Committee of Shanghai Ninth People’s Hospital (No. SH9H-2019-T159-2), and were in compliance with the NIH Guide for the Care and Use of Laboratory Animals [2018].
Open Access Statement: This is an Open Access article distributed in accordance with the Creative Commons Attribution-NonCommercial-NoDerivs 4.0 International License (CC BY-NC-ND 4.0), which permits the non-commercial replication and distribution of the article with the strict proviso that no changes or edits are made and the original work is properly cited (including links to both the formal publication through the relevant DOI and the license). See: https://creativecommons.org/licenses/by-nc-nd/4.0/.
References
- Tikhonova AN, Dolgalev I, Hu H, et al. The bone marrow microenvironment at single-cell resolution. Nature 2019;569:222-8. [Crossref] [PubMed]
- Drake MT, Clarke BL, Oursler MJ, et al. Cathepsin K Inhibitors for Osteoporosis: Biology, Potential Clinical Utility, and Lessons Learned. Endocr Rev 2017;38:325-50. [Crossref] [PubMed]
- Singer FR. Paget's disease of bone-genetic and environmental factors. Nat Rev Endocrinol 2015;11:662-71. [Crossref] [PubMed]
- Puchner A, Saferding V, Bonelli M, et al. Non-classical monocytes as mediators of tissue destruction in arthritis. Ann Rheum Dis 2018;77:1490-7. [Crossref] [PubMed]
- Cheung LC, Tickner J, Hughes AM, et al. New therapeutic opportunities from dissecting the pre-B leukemia bone marrow microenvironment. Leukemia 2018;32:2326-38. [Crossref] [PubMed]
- Farr JN, Xu M, Weivoda MM, et al. Targeting cellular senescence prevents age-related bone loss in mice. Nat Med 2017;23:1072-9. [Crossref] [PubMed]
- Morris JA, Kemp JP, Youlten SE, et al. An atlas of genetic influences on osteoporosis in humans and mice. Nat Genet 2019;51:258-66. [Crossref] [PubMed]
- Zhang X, Dai Z, Lau EHY, et al. Prevalence of bone mineral density loss and potential risk factors for osteopenia and osteoporosis in rheumatic patients in China: logistic regression and random forest analysis. Ann Transl Med 2020;8:226. [Crossref] [PubMed]
- Baccaro LF, Conde DM, Costa-Paiva L, et al. The epidemiology and management of postmenopausal osteoporosis: a viewpoint from Brazil. Clin Interv Aging 2015;10:583-91. [Crossref] [PubMed]
- Zhu X, Zhang K, Lu K, et al. Inhibition of pyroptosis attenuates Staphylococcus aureus-induced bone injury in traumatic osteomyelitis. Ann Transl Med 2019;7:170. [Crossref] [PubMed]
- Silbermann R, Bolzoni M, Storti P, et al. Bone marrow monocyte-/macrophage-derived activin A mediates the osteoclastogenic effect of IL-3 in multiple myeloma. Leukemia 2014;28:951-4. [Crossref] [PubMed]
- Zou W, Reeve JL, Liu Y, et al. DAP12 couples c-Fms activation to the osteoclast cytoskeleton by recruitment of Syk. Mol Cell 2008;31:422-31. [Crossref] [PubMed]
- Wu M, Chen W, Lu Y, et al. Galpha13 negatively controls osteoclastogenesis through inhibition of the Akt-GSK3beta-NFATc1 signalling pathway. Nat Commun 2017;8:13700. [Crossref] [PubMed]
- Ha H, Kwak HB, Le SW, et al. Lipid rafts are important for the association of RANK and TRAF6. Exp Mol Med 2003;35:279-84. [Crossref] [PubMed]
- Zarei A, Morovat A, Javaid K, et al. Vitamin D receptor expression in human bone tissue and dose-dependent activation in resorbing osteoclasts. Bone Res 2016;4:16030. [Crossref] [PubMed]
- Cui Y, Zhao X, Mei L, et al. Osteon Myospalacem Baileyi attenuates osteoclast differentiation through RANKL induced NFAT pathways. J Ethnopharmacol 2018;213:65-71. [Crossref] [PubMed]
- Liu W, Zhou L, Zhou C, et al. GDF11 decreases bone mass by stimulating osteoclastogenesis and inhibiting osteoblast differentiation. Nat Commun 2016;7:12794. [Crossref] [PubMed]
- Wang X, Wei W, Krzeszinski JY, et al. A Liver-Bone Endocrine Relay by IGFBP1 Promotes Osteoclastogenesis and Mediates FGF21-Induced Bone Resorption. Cell Metab 2015;22:811-24. [Crossref] [PubMed]
- Xin Z, Jin C, Chao L, et al. A Matrine Derivative M54 Suppresses Osteoclastogenesis and Prevents Ovariectomy-Induced Bone Loss by Targeting Ribosomal Protein S5. Front Pharmacol 2018;9:22. [Crossref] [PubMed]
- Yuan Y, Wu SJ, Liu X, et al. Antioxidant effect of salidroside and its protective effect against furan-induced hepatocyte damage in mice. Food Funct 2013;4:763-9. [Crossref] [PubMed]
- Liang SZ, Meng YC, An XZ, et al. Studies on antioxidative activities of different polarity fractions of Rhodiola rosea L. extract in vitro. Med Plant 2010;1:68-70.
- Buchwald W, Mordalski R, Kucharski WA, et al. Effect of fertilization on roseroot (Rhodiola rosea L.) yield and content of active compounds. Acta Sci Pol Hortorum Cultus 2015;14:109-21.
- Xin X, Yao D, Zhang K, et al. Protective effects of Rosavin on bleomycin-induced pulmonary fibrosis via sup-pressing fibrotic and inflammatory signaling pathways in mice. Biomed Pharmacother 2019;115:108870. [Crossref] [PubMed]
- Panossian A, Nikoyan N, Ohanyan N, et al. Comparative study of Rhodiola preparations on behavioral despair of rats. Phytomedicine 2008;15:84-91. [Crossref] [PubMed]
- Chen X, Zhi X, Cao L, et al. Matrine derivate MASM uncovers a novel function for ribosomal protein S5 in osteoclastogenesis and postmenopausal osteoporosis. Cell Death Dis 2017;8:e3037. [Crossref] [PubMed]
- Chen X, Zhi X, Yin Z, et al. 18beta-Glycyrrhetinic Acid Inhibits Osteoclastogenesis In Vivo and In Vitro by Blocking RANKL-Mediated RANK-TRAF6 Interactions and NF-kappaB and MAPK Signaling Pathways. Front Pharmacol 2018;9:647. [Crossref] [PubMed]
- Zhang Y, Xu S, Li K, et al. mTORC1 Inhibits NF-kappaB/NFATc1 Signaling and Prevents Osteoclast Precursor Differentiation, In Vitro and In Mice. J Bone Miner Res 2017;32:1829-40. [Crossref] [PubMed]
- Choi HK, Kang HR, Jung E, et al. Early estrogen-induced gene 1, a novel RANK signaling component, is essential for osteoclastogenesis. Cell Res 2013;23:524-36. [Crossref] [PubMed]
- Xiao Y, Li K, Wang Z, et al. Pectolinarigenin prevents bone loss in ovariectomized mice and inhibits RANKL-induced osteoclastogenesis via blocking activation of MAPK and NFATc1 signaling. J Cell Physiol 2019;234:13959-68. [Crossref] [PubMed]
- Fujita K, Iwasaki M, Ochi H, et al. Vitamin E decreases bone mass by stimulating osteoclast fusion. Nat Med 2012;18:589-94. [Crossref] [PubMed]
- Lian JB, Stein GS, van Wijnen AJ, et al. MicroRNA control of bone formation and homeostasis. Nat Rev Endocrinol 2012;8:212-27. [Crossref] [PubMed]
- Chen X, Zhi X, Wang J, et al. RANKL signaling in bone marrow mesenchymal stem cells negatively regulates osteoblastic bone formation. Bone Res 2018;6:34. [Crossref] [PubMed]
- Jin Z, Wei W, Yang M, et al. Mitochondrial complex I activity suppresses inflammation and enhances bone re-sorption by shifting macrophage-osteoclast polarization. Cell Metab 2014;20:483-98. [Crossref] [PubMed]
- Klein-Hessling S, Muhammad K, Klein M, et al. NFATc1 controls the cytotoxicity of CD8(+) T cells. Nat Commun 2017;8:511. [Crossref] [PubMed]
- Liu H, Liu Z, Du J, et al. Thymidine phosphorylase exerts complex effects on bone resorption and formation in myeloma. Sci Transl Med 2016;8:353ra113. [Crossref] [PubMed]
- Ikebuchi Y, Aoki S, Honma M, et al. Coupling of bone resorption and formation by RANKL reverse signalling. Nature 2018;561:195-200. [Crossref] [PubMed]
- Bartelt A, Behler-Janbeck F, Beil FT, et al. Lrp1 in osteoblasts controls osteoclast activity and protects against osteoporosis by limiting PDGF-RANKL signaling. Bone Res 2018;6:4. [Crossref] [PubMed]
(English Language Editors: A. Kassem and J. Reynolds)