Role of a successful spontaneous breathing trial in ventilator liberation in brain-injured patients
Introduction
In critically ill patients with brain injury, mechanical ventilation aims to minimize secondary brain injury (e.g., due to ischemia and edema) by securing oxygenation and preventing hypercapnic acidosis (1-5). However, the time-dependent nature of ventilator-related complications requires timely ventilator liberation (6-10). Protocolized weaning, including the use of a spontaneous breathing trial (SBT), improves outcomes and is recommended in international guidelines (11-15).
Indications for endotracheal intubation and mechanical ventilation in patients with brain injury are largely different from those of critically ill patients without brain injury (further referred to general critically ill patients) (8,16-18). The predictive value of a successful SBT for ventilator liberation in patients with brain injury is largely unknown. Recent studies have demonstrated higher extubation failure rates after a successful SBT in patients with brain injury compared with general critically ill patients (19-23). After a successful SBT, extubation failure rates between 31% and 46% have been reported in patients with brain injury. In contrast, 10–14% of general critically ill patients completing an SBT failed ventilator liberation (24-27). Reasons for the high failure rates in patients with brain injury may be related to upper airway function or neurological status (19,21,23). Therefore, guidelines specifically recommend assessment for airway protective ability, including suctioning frequency, cough strength, and mental status (11,28). However, the role of impaired respiratory reserve has not been systematically evaluated in this population. This is important, as ventilator reconnection is frequent in tracheostomized patients with brain injury, indicating that factors other than upper airway protection may be involved (29). Therefore, the aim of the current study was to investigate patients with brain injury and to determine if the respiratory physiological parameters during a successful SBT are different between patients with successful liberation versus failed ventilator liberation.
We present the following article in accordance with the STROBE reporting checklist (available at http://dx.doi.org/10.21037/atm-20-6407).
Methods
Study design and patients
The present prospective study was conducted in the neurocritical intensive care unit (ICU) of the Beijing Tiantan Hospital, Capital Medical University, Beijing, China. Patients with brain injury (≥18 years old), who were mechanically ventilated for >48 h, were screened daily between 9 AM and 10 AM (from Monday to Friday) (Appendix S1). The readiness criteria for an SBT were as follows: (I) intracranial pressure <20 mmHg, or no clinical evidence of elevated intracranial pressure; (II) adequate oxygenation (PO2/FIO2 >200 mmHg or pulse oximetry >95% with FIO2 ≤0.5) and low level of ventilator support [positive end-expiratory pressure (PEEP) ≤5 cmH2O, and pressure support ≤8 cmH2O); (III) hemodynamic stability (no vasopressor support and systolic blood pressure between 90 and 160 mmHg); and (IV) no sedatives or intermittent dosing of sedatives. Patients were excluded if they were moribund or brain dead, had spinal cord injury or any contraindications for esophageal catheter placement, or were tracheostomized before or within 48 h after the SBT (as it affects weaning strategies).
The study was conducted in accordance with the Declaration of Helsinki (as revised in 2013), and was approved by the institutional ethics board of Beijing Tiantan Hospital (No. KY2016-018-02). Informed consent was obtained from all patients or legal representatives.
Study protocol
Patients were ventilated with a Servo-i ventilator (Maquet Critical Care, Sölna, Sweden). Nasogastric catheters for diaphragmatic electrical activity (EAdi) (Maquet Critical Care, Sweden) were inserted to replace the standard nasogastric feeding tube, and esophageal pressure (Pes) monitoring (SensorMedics, Yorba Linda, CA, USA) was conducted. Details for the catheter placement have been described previously (30-35). Baseline data were collected just before the SBT in pressure support ventilation (PSV) mode (inspiratory support: 8 cmH2O, PEEP: 5 cmH2O). Subsequently, patients underwent a 30-min SBT at continuous positive airway pressure (CPAP) of 5 cmH2O, with no inspiratory pressure support and without changing FIO2. At 1, 5, 10, 20, and 30 min after the start of SBT, an end-expiratory occlusion (EEO) maneuver was applied on the ventilator for at least 3 consecutive breathing efforts to determine neuromechanical efficiency. Criteria for terminating the SBT are listed in Table S1 (6).
After completing the SBT, patients were disconnected from the ventilator, either breathing through a T-tube circuit with humidified oxygen or extubated as decided by the clinical team using a screen checklist (Table S2) (36,37). Reconnection to the ventilator support was solely decided by the clinical team, who were unaware of the physiological data obtained for study purposes (especially data derived from EAdi and Pes). Reasons for resuming mechanical ventilation and/or reintubation were recorded. As per the clinical protocol, reintubation and/or reconnection to the ventilator was performed in patients meeting at least 1 of the following criteria: (I) decreased mental status compared with pre-SBT level of consciousness; (II) peripheral oxygen saturation <90%, despite FIO2 >0.5; (III) increased respiratory effort, such as tachypnea, accessory respiratory muscle recruitment, or thoracic–abdominal paradox (36). Failed ventilator liberation was defined as the need for invasive ventilator support within 48 h after the SBT, independent of the presence of an artificial airway (tracheostomy or endotracheal tube). The Glasgow Coma Scale (GCS) score was recorded before ventilator disconnection to assess neurological status. The verbal score was counted as 1 for patients with an artificial airway (38). Arterial blood samples and hemodynamic parameters (i.e., non-invasive blood pressure and heart rate) were collected before and at the end of the SBT. Patients were followed up until they were reconnected to ventilation, extubated, tracheostomized, discharged from the hospital, or 28 days after the first successful SBT, whichever came first.
Data acquisition during SBT
Flow was measured with a heated Fleisch pneumotachograph (Vitalograph, Lenexa, KS, USA) placed between the Y-piece of the ventilator circuit and the endotracheal tube. Two differential pressure transducers (KT 100D-2; KleisTEK di Cosimo Micelli, Monopoli, Italy; range: ±100 cmH2O) were used to measure the airway opening pressure (Pao) and the Pes. The transducers were connected proximal to the endotracheal tube (Pao) and to the esophageal catheter (Pes), respectively. Flow and pressures signals were recorded by an ICU-Lab pressure box (ICU Lab, KleisTEK Engineering, Bari, Italy), with a sample frequency of 100 Hz. The pressure transducers were calibrated with a water column and the pneumotachograph with a 1-L calibration syringe (SN: 554–2266; Hans Rudolph, Shawnee, KS, USA) prior to each measurement (30). The EAdi catheter was connected to the Servo-i ventilator. EAdi signals were recorded at a sample frequency of 100 Hz using dedicated software (Servo-tracker version 4.1. Maquet, Sweden). All recordings were saved and synchronized for offline analysis in a software developed for the ICU-Lab monitoring system (DigimaClic-1, ICU-Lab System, KleisTEK, Italy).
Data analysis
As per the study design, only patients completing the SBT were included for further analysis. Data were analyzed on a breath-by-breath basis at 6 time points as follows: at baseline (PSV prior to the SBT), and at 1, 5, 10, 20, and 30 min after start of the SBT. These time points were arbitrarily selected, as used previously (39,40). For each time point, 5 consecutive breaths without artifacts (e.g., esophageal contractions) were selected.
Inspiratory time, expiratory time, respiratory rate (RR), minute ventilation, and duty cycle were derived from the flow signal. Tidal volume (Vt) was computed as the time integral of inspiratory flow. Intrinsic PEEP was calculated as the decrease in Pes until the start of inspiratory flow (41). The chest wall recoil pressure (Pcw) was calculated as the product of lung volume and the predicted elastance of the chest wall (4% of vital capacity). The effort of the inspiratory muscles was quantified by calculating the global inspiratory muscle pressure (Pmus) and the inspiratory esophageal pressure–time product (PTPes,insp). Pmus was calculated as the peak difference between Pcw and Pes during inspiration (ΔPmus). PTPes,insp was calculated as the time interval of the difference between Pes and Pcw (Figure S1) (42).
Neural respiratory drive was measured as the inspiratory increase in EAdi from basal activity (ΔEAdi). The static neuromechanical efficiency (NMEoccl) of the diaphragm was computed as ΔPao/ΔEAdi during an EEO maneuver (31). The NMEoccl was calculated from the first occluded breathing effort. In the presence of artifacts in the EAdi waveform, the second or third breathing effort of the same series was selected (Figure S2). This was acceptable, as we have demonstrated that NMEoccl remains stable during a 20-s occlusion. The dynamic neuromechanical efficiency of the diaphragm was defined as ΔPmus/ΔEAdi measured during tidal breathing (Figure S3). As the respiratory centers are located in the brainstem, we performed χ2-test analysis to determine if responses were different between patients with or without brainstem involvement (43).
Statistical analysis
Data were analyzed with IBM SPSS Statistics version 22.0 (IBM Corp., Armonk, NY, USA) and GraphPad Prism version 7.0 (GraphPad Software, Inc., San Diego, CA, USA). Assumption of normality was tested using the Shapiro-Wilk normality test. Differences in patient characteristics between two groups were analyzed using the t-test, Mann-Whitney U-test, or χ2-test, as appropriate.
To analyze the effects of time and group (failure and success) on each respiratory parameter, a linear mixed model design was used with a fixed effect of time, group, and group-by-time interaction, and a random effect of patient. For nonparametric respiratory parameters, an appropriate mathematical transformation was applied. Post-hoc pairwise comparisons of estimated means over time and between groups at each time point were performed after applying Bonferroni correction (34). Sensitivity analyses were performed in a subgroup analysis by defining the failed ventilator liberation as the need for invasive ventilator support within 7 days after the SBT.
For all tests, a 2-tailed P value <0.05 was considered to be statistically significant. Values were given as mean ± standard deviation or median (interquartile range) for continuous variables, and as number and percentage for categorical variables. Because of the exploratory nature of this study, convenience sampling was used (39,40).
Results
During the 12-month study period, 251 patients were screened, and 46 were included for analysis (Figure 1). Reasons for exclusion included mechanical ventilation <48 h (n=82) and early tracheostomy (n=59). Patient characteristics at baseline are reported in Table 1. Seventeen (37%) patients failed ventilator liberation within 48 h after a successful SBT. Of these patients, 4 were reintubated and 13 were reconnected to the ventilator before extubation. Reasons for failing ventilator liberation within 48 h were postextubation upper airway obstruction (n=4), neurological deterioration (n=8), and respiratory-related issues (n=5). Clinical characteristics of patients failing ventilator liberation within 48 h were not different from patients with successful liberation (Table 1). The proportion of patients that remained liberated from mechanical ventilation decreased rapidly within 7 days after the first SBT and remained stable thereafter (Figure 2). In total, 28 patients were reconnected to the ventilator within 7 days after the first SBT. The median time until reconnection was 1.4 (0.4–3) days. Further patient details are presented in Table S3.
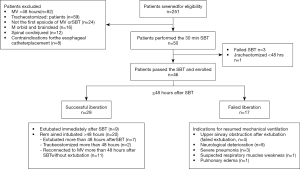
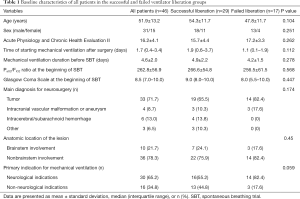
Full table
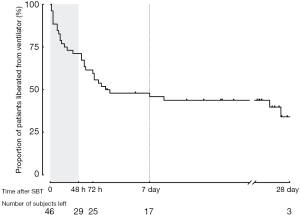
Breathing pattern during SBT in successful and failed liberation
Immediately after the transition from PSV to SBT, Vt decreased and RR increased in both groups, but remained stable during the rest of the SBT (Table 2). Minute ventilation decreased in the successful liberation group only [9.0 (7.7–10.4) to 7.8 (6.1–10.0) L/min, P<0.05]. There was no difference in respiratory pattern between the groups. Other respiratory physiological parameters are shown in Table S4.
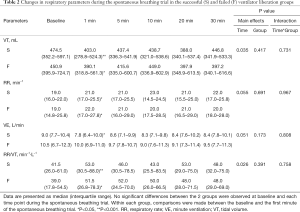
Full table
Respiratory muscle effort during SBT in successful and failed liberation
As shown in Figure 3, parameters of inspiratory muscle effort including ∆Pmus, PTPes,insp, and ∆EAdi increased after the transition from PSV to SBT in both groups, but remained stable during the SBT. There were no significant differences between groups.
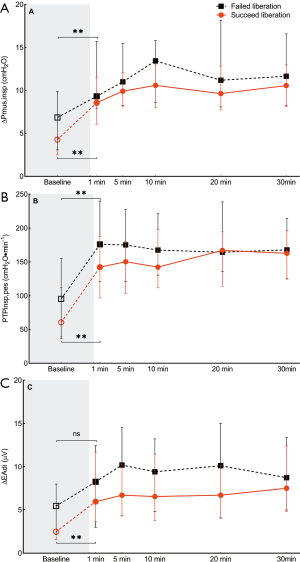
NMEoccl, a measure for neuromechanical efficiency of the diaphragm, did not change after the transition from PSV to the SBT and remained stable during the SBT. No difference was found in NMEoccl between the two groups (Figure 4).
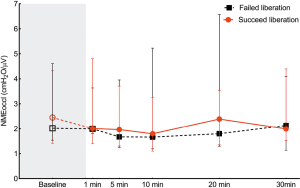
A subgroup analysis was performed to compare respiratory physiological parameters between patients that were successfully liberated from mechanical ventilation and patients failing ventilator liberation within 7 days. This, however, did not change the results compared with failure within 48 h after successful SBT (Table S5). Subgroup analysis was also performed in patients remaining intubated within 48 h. First, the rate of liberation failure was comparable to that of patients who were extubated (39.4% vs. 30.8%, P=0.74). Second, the respiratory physiological variables showed no difference between patients who failed and those were successfully liberated from the ventilator within 48 h (Table S6).
Arterial blood gas variables and hemodynamic variables
Arterial blood gas and hemodynamic variables are shown in Table 3. The arterial HCO3– at the start of the SBT was significantly higher in the successful liberation group compared with the failed liberation group (28.4±2 vs. 26.1±2.7 mmol/L, P=0.001). There were no differences between groups in hemodynamic variables at baseline or during the SBT.
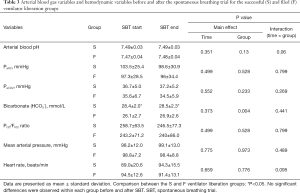
Full table
Clinical outcomes
Clinical outcomes are shown in Table 4. There were no significant differences between the failed liberation and successful liberation groups with regard to discharge status, ICU length of stay, and hospital length of stay. At the time of hospital discharge, 40 (87.0%) patients were liberated from the ventilator in a median of 3.4 (0–6.2) days after the first successful SBT. In these patients, the median number of SBTs before final ventilator liberation was 2 [1–5]. Two patients died before ventilator liberation and 4 patients were transferred to a local hospital for end-of-life care.
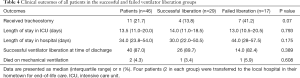
Full table
Discussion
In the current study, we demonstrated that, in patients with brain injury completing a 30-min SBT, 37% required invasive ventilator support within 48 h and 61% within 7 days. In-depth analysis of respiratory muscle effort and breathing patterns during the SBT did not provide any predictive information for ventilator liberation failure. Therefore, the clinical usefulness of a successful SBT in this patient category is debatable.
Successful SBT and failed ventilator liberation
Our study demonstrated that, in patients with brain injury, ventilator liberation failure within 48 h after a successful SBT was more common (37%) compared with general critically ill patients (24-27,44). In general critically ill patients, Subira et al. reported 12% of extubation failure within 48 h after a successful SBT (24), and Burns et al. reported that the reintubation rate was 13% in younger patients (age <65 years) and 10.8% in patients aged ≥65 years (25). The differences in ventilator reconnection may be related to underlying illness (45). Reasons for resuming ventilator support may be different between general critically ill patients and patients with brain injury. In general critically ill patients, reasons for resuming ventilator support are mainly related to cardiorespiratory function (24,25,46,47). In our study, 4 of 17 (24%) patients were reconnected to a ventilator because of upper airway obstruction and 8 of 17 (47%) patients for neurological reasons. These data are in line with previous studies on patients with brain injury (21,48). Karanjia et al. retrospectively investigated the cause of reintubation in 99 patients with brain injury reintubated within 72 h after a successful SBT (48). They found that the primary cause for reintubation was respiratory distress associated with decreased mental status, without signs of aspiration or pneumonia. Given that most patients with brain injury are intubated and mechanically ventilated for neurological reasons, these results are not surprising. Accordingly, in patients with brain injury, the predictive value of a successful SBT for ventilator liberation appears limited. Possibly, well-known parameters provided by an SBT may not be as relevant in patients with brain injury. Neurological dysfunction may not affect the ability to breathe (tested with SBT), but may affect ventilator liberation success.
We explored if more advanced respiratory physiological parameters collected during the SBT were helpful in predicting ventilator liberation outcome. Similar respiratory parameters have been systematically measured during a 30-min SBT in general critically ill patients (39,40). In these studies, variables of breathing pattern (RR, Vt, minute ventilation, and RR/Vt), EAdi and its derived parameters (neuromechanical and neuroventilatory efficiency) were reliable and early predictors for ventilator liberation outcome. In contrast, we did not find differences in physiological parameters related to breathing pattern or respiratory muscle effort between liberation success and failure patients with brain injury in our study. Interestingly, the values of the respiratory parameters assessed in our study were similar to the values earlier reported in successfully liberated general critically ill patients (39,40). Immediate changes occurred after the transition from PSV to the SBT, but remained relatively stable during the course of the SBT. This indicates that limited cardiorespiratory physiological reserve was not the main reason of ventilator liberation failure. Although the cardiorespiratory reserve and neurorespiratory drive can be assessed during an SBT, it is unlikely that neurological deterioration affects the course of an SBT. Therefore, the clinical usefulness of a successful SBT in patients with brain injury can be challenged or even considered misleading. In patients with brain injury, both neurological and non-neurological features should be evaluated before the decision of ventilator liberation. Therefore, other valid tools to assess underlying neurological conditions need to be developed and prospectively evaluated to predict the success of ventilator liberation.
Neurological status is one of the major concerns before the decision of ventilator liberation is made for patients with brain injury. Investigators have used the GCS to decide on extubation in these patients (49,50). However, the role of the consciousness level on extubation outcome remains controversial (20,38,51,52). Whether a low level of consciousness affects the breathing pattern in brain-injured patients is unclear. In our study, the GCS at the time of ventilator disconnection was similar for successful and failed patients. Moreover, half of the patients with a GCS <8 were successfully liberated from the ventilator. Accordingly, the level of consciousness appears not a main predictor for ventilator liberation outcome in these patients. Studies have demonstrated that together with visual pursuit, adequate swallowing function may improve ventilator liberation outcome (50), although no recommendations on this topic are available in recent guidelines (28). Therefore, further studies are required to investigate more specific parameters, rather than the level of consciousness, to assess neurological status during the SBTs.
High rate of liberation failure
Compared with other studies in patients with brain injury, the liberation failure rate in our patients appeared rather high (20,21,50,53). This may be related to the anatomical site of the injury. In our study, 54% (25/46) of the patients had infratentorial lesions and 10 of these patients had lesions that involved the brainstem. Infratentorial lesions include lesions located in the cerebellum and/or brainstem, which puts patients at high risk of respiratory compromise by involving primary neural respiratory centers, lower cranial nerve nuclei, and reticular activating pathways (43,54).
In addition, the type of SBT used may affect extubation outcome. SBT with low inspiratory support reduces patient respiratory effort. However, this technique may overestimate patients’ ability to breathe without assistance. Conversely, an SBT with a T-piece provides no inspiratory (and expiratory) support, which more accurately reflects the physiological condition after extubation (55). However, the sudden drop in PEEP may induce cardiac dysfunction (56,57). It has been suggested that, as an inspiratory pressure support, SBT improves extubation outcomes compared with T-piece SBT (24,58). In our study we did not apply inspiratory support, but CPAP only. We chose this strategy as it better reflects breathing effort after extubation (55,57).
Strengths and limitations
The present study has several strengths, including the in-depth analysis of breathing pattern and respiratory muscle effort during an SBT. This has not been evaluated in earlier studies in patients with brain injury. In addition, half of the patients had an infratentorial lesion, 10 of which had brainstem involvement. This provided an opportunity to explore the impact of the anatomical location on the liberation outcome, especially brainstem injury. Our study suggests that, for patients completing an SBT, the anatomic location of the lesion is not associated with the rate of liberation failure.
However, several limitations should be acknowledged. First, this was a single-center study, possibly challenging the generalizability of the data. Nevertheless, the study was performed in the largest neurological ICU in China, admitting the full spectrum of patients with brain injury. Second, we did not perform a formal sample size analysis. This is reasonable given the explorative nature of the study, and the current sample size was comparable to earlier studies (39,40). The results of the current study are helpful for sample size calculation in future studies with this specific patient population. Third, although all patients completing the SBT were disconnected from the ventilator, not all patients were extubated directly after the SBT. Therefore, the difference between ventilator liberation and extubation should be acknowledged. However, our study showed that the liberation failure rate in patients not extubated was comparable to patients immediately extubated. Moreover, respiratory physiological parameters were not different between the liberation success and failure groups in these subgroups of patients. Breathing without ventilator assistance through an endotracheal tube after a successful SBT is common in our ICU, especially in patients with anatomical injury at the brainstem level. This strategy allows for the recovery of upper airway protective reflexes before the decision of early tracheostomy. The prerequisite for this decision-making period is that patients are awake and mobilized.
Conclusions
A successful SBT does not predict ventilator liberation in patients with brain injury, indicating that factors other than respiratory dysfunction are involved in this specific population. Given the high rate of ventilator liberation failure in patients with brain injury, further studies focusing on nonrespiratory parameters to predict liberation success are warranted.
Acknowledgments
Part of the data have been reported as an abstract at the European Society of Intensive Care Medicine conference, Berlin, 2019.
Funding: None.
Footnote
Reporting Checklist: The authors have completed the STROBE reporting checklist Available at http://dx.doi.org/10.21037/atm-20-6407
Data Sharing Statement: Available at http://dx.doi.org/10.21037/atm-20-6407
Conflicts of Interest: All authors have completed the ICMJE uniform disclosure form (available at http://dx.doi.org/10.21037/atm-20-6407). AHJ reports personal fees from Liberate Medical (USA), outside the submitted work. LMH reports personal fees from Liberate Medical and Getinge, outside the submitted work. The other authors have no conflicts of interest to declare.
Ethical Statement: The authors are accountable for all aspects of the work in ensuring that questions related to the accuracy or integrity of any part of the work are appropriately investigated and resolved. The study was conducted in accordance with the Declaration of Helsinki (as revised in 2013) and was approved by the institutional ethics board of Beijing Tiantan Hospital (No. KY2016-018-02). Informed consent was obtained from all patients.
Open Access Statement: This is an Open Access article distributed in accordance with the Creative Commons Attribution-NonCommercial-NoDerivs 4.0 International License (CC BY-NC-ND 4.0), which permits the non-commercial replication and distribution of the article with the strict proviso that no changes or edits are made and the original work is properly cited (including links to both the formal publication through the relevant DOI and the license). See: https://creativecommons.org/licenses/by-nc-nd/4.0/.
References
- Zauner A, Daugherty WP, Bullock MR, et al. Brain Oxygenation and Energy Metabolism: Part I—Biological Function and Pathophysiology. Neurosurgery 2002;51:289-301; discussion 302. [PubMed]
- Rastogi P, Popp A. Management of Respiratory Dysfunction in the Neurosurgical Patient. Contemporary Neurosurgery 1995;17:1-5. [Crossref]
- Frisvold SK, Robba C, Guerin C. What respiratory targets should be recommended in patients with brain injury and respiratory failure? Intensive Care Med 2019;45:683-6. [Crossref] [PubMed]
- Carney N, Totten AM, O'Reilly C, et al. Guidelines for the Management of Severe Traumatic Brain Injury, Fourth Edition. Neurosurgery 2017;80:6-15.
- Rosenfeld JV, Maas AI, Bragge P, et al. Early management of severe traumatic brain injury. Lancet 2012;380:1088-98. [Crossref] [PubMed]
- McConville JF, Kress JP. Weaning patients from the ventilator. N Engl J Med 2012;367:2233-9. [Crossref] [PubMed]
- Boles JM, Bion J, Connors A, et al. Weaning from mechanical ventilation. Eur Respir J 2007;29:1033-56. [Crossref] [PubMed]
- Pelosi P, Ferguson ND, Frutos-Vivar F, et al. Management and outcome of mechanically ventilated neurologic patients. Crit Care Med 2011;39:1482-92. [Crossref] [PubMed]
- Mascia L, Zavala E, Bosma K, et al. High tidal volume is associated with the development of acute lung injury after severe brain injury: an international observational study. Crit Care Med 2007;35:1815-20. [Crossref] [PubMed]
- Kahn JM, Caldwell EC, Deem S, et al. Acute lung injury in patients with subarachnoid hemorrhage: incidence, risk factors, and outcome. Crit Care Med 2006;34:196-202. [Crossref] [PubMed]
- MacIntyre NR, Cook DJ, Ely EW Jr, et al. Evidence-based guidelines for weaning and discontinuing ventilatory support: a collective task force facilitated by the American College of Chest Physicians; the American Association for Respiratory Care; and the American College of Critical Care Medicine. Chest 2001;120:375S-95S. [Crossref] [PubMed]
- Schmidt GA, Girard TD, Kress JP, et al. Liberation From Mechanical Ventilation in Critically Ill Adults: Executive Summary of an Official American College of Chest Physicians/American Thoracic Society Clinical Practice Guideline. Chest 2017;151:160-5. [Crossref] [PubMed]
- Ouellette DR, Patel S, Girard TD, et al. Liberation From Mechanical Ventilation in Critically Ill Adults: An Official American College of Chest Physicians/American Thoracic Society Clinical Practice Guideline: Inspiratory Pressure Augmentation During Spontaneous Breathing Trials, Protocols Minimizing Sedation, and Noninvasive Ventilation Immediately After Extubation. Chest 2017;151:166-80. [Crossref] [PubMed]
- Esteban A, Frutos F, Tobin MJ, et al. A comparison of four methods of weaning patients from mechanical ventilation. Spanish Lung Failure Collaborative Group. N Engl J Med 1995;332:345-50. [Crossref] [PubMed]
- Ely EW, Baker AM, Dunagan DP, et al. Effect on the duration of mechanical ventilation of identifying patients capable of breathing spontaneously. N Engl J Med 1996;335:1864-9. [Crossref] [PubMed]
- Nyquist P, Stevens RD, Mirski MA. Neurologic injury and mechanical ventilation. Neurocrit Care 2008;9:400-8. [Crossref] [PubMed]
- Stevens RD, Lazaridis C, Chalela JA. The role of mechanical ventilation in acute brain injury. Neurol Clin 2008;26:543-63. x. [Crossref] [PubMed]
- Demling R, Riessen R. Pulmonary dysfunction after cerebral injury. Crit Care Med 1990;18:768-74. [Crossref] [PubMed]
- Namen AM, Ely EW, Tatter SB, et al. Predictors of successful extubation in neurosurgical patients. Am J Respir Crit Care Med 2001;163:658-64. [Crossref] [PubMed]
- Asehnoune K, Seguin P, Lasocki S, et al. Extubation Success Prediction in a Multicentric Cohort of Patients with Severe Brain Injury. Anesthesiology 2017;127:338-46. [Crossref] [PubMed]
- Godet T, Chabanne R, Marin J, et al. Extubation Failure in Brain-injured Patients: Risk Factors and Development of a Prediction Score in a Preliminary Prospective Cohort Study. Anesthesiology 2017;126:104-14. [Crossref] [PubMed]
- Kutchak FM, Rieder MM, Victorino JA, et al. Simple motor tasks independently predict extubation failure in critically ill neurological patients. J Bras Pneumol 2017;43:183-9. [Crossref] [PubMed]
- Ibrahim AS, Aly MG, Abdel-Rahman KA, et al. Semi-quantitative Cough Strength Score as a Predictor for Extubation Outcome in Traumatic Brain Injury: A Prospective Observational Study. Neurocrit Care 2018;29:273-9. [Crossref] [PubMed]
- Subira C, Hernandez G, Vazquez A, et al. Effect of Pressure Support vs T-Piece Ventilation Strategies During Spontaneous Breathing Trials on Successful Extubation Among Patients Receiving Mechanical Ventilation: A Randomized Clinical Trial. JAMA 2019;321:2175-82. [Crossref] [PubMed]
- Burns KEA, Wong JTY, Dodek P, et al. Frequency of Screening for Weaning From Mechanical Ventilation: Two Contemporaneous Proof-of-Principle Randomized Controlled Trials. Crit Care Med 2019;47:817-25. [Crossref] [PubMed]
- Jaber S, Quintard H, Cinotti R, et al. Risk factors and outcomes for airway failure versus non-airway failure in the intensive care unit: a multicenter observational study of 1514 extubation procedures. Crit Care 2018;22:236. [Crossref] [PubMed]
- Fernandez MM, Gonzalez-Castro A, Magret M, et al. Reconnection to mechanical ventilation for 1 h after a successful spontaneous breathing trial reduces reintubation in critically ill patients: a multicenter randomized controlled trial. Intensive Care Med 2017;43:1660-7. [Crossref] [PubMed]
- Robba C, Poole D, McNett M, et al. Mechanical ventilation in patients with acute brain injury: recommendations of the European Society of Intensive Care Medicine consensus. Intensive Care Medicine 2020; [Crossref] [PubMed]
- Vaschetto R, Frigerio P, Sommariva M, et al. Evaluation of a systematic approach to weaning of tracheotomized neurological patients: an early interrupted randomized controlled trial. Ann Intensive Care 2015;5:54. [Crossref] [PubMed]
- He X, Sun XM, Chen GQ, et al. Use of Cardiac Cycle Locating to Minimize the Influence of Cardiac Artifacts on Esophageal Pressure Measurement During Dynamic Occlusion Test. Respir Care 2018;63:169-76. [Crossref] [PubMed]
- Jansen D, Jonkman AH, Roesthuis L, et al. Estimation of the diaphragm neuromuscular efficiency index in mechanically ventilated critically ill patients. Crit Care 2018;22:238. [Crossref] [PubMed]
- Baydur A, Behrakis PK, Zin WA, et al. A simple method for assessing the validity of the esophageal balloon technique. Am Rev Respir Dis 1982;126:788-91. [PubMed]
- Schellekens WM, Heunks LMA. Appropriate positioning of the NAVA catheter. Intensive Care Med 2016;42:633-4. [Crossref] [PubMed]
- Doorduin J, Roesthuis LH, Jansen D, et al. Respiratory Muscle Effort during Expiration in Successful and Failed Weaning from Mechanical Ventilation. Anesthesiology 2018;129:490-501. [Crossref] [PubMed]
- Doorduin J, Sinderby CA, Beck J, et al. The calcium sensitizer levosimendan improves human diaphragm function. Am J Respir Crit Care Med 2012;185:90-5. [Crossref] [PubMed]
- Wu YX, Chen H, Zhou JX. Short-term use of remifentanil during endotracheal extubation for prophylactic analgesia in neurosurgical patients after craniotomy (SURE after Craniotomy Study): a study protocol and statistical analysis plan for a randomised controlled trial. BMJ Open 2014;4:e005635 [Crossref] [PubMed]
- Cai YH, Zeng HY, Shi ZH, et al. Factors influencing delayed extubation after infratentorial craniotomy for tumour resection: a prospective cohort study of 800 patients in a Chinese neurosurgical centre. J Int Med Res 2013;41:208-17. [Crossref] [PubMed]
- Coplin WM, Pierson DJ, Cooley KD, et al. Implications of extubation delay in brain-injured patients meeting standard weaning criteria. Am J Respir Crit Care Med 2000;161:1530-6. [Crossref] [PubMed]
- Dres M, Schmidt M, Ferre A, et al. Diaphragm electromyographic activity as a predictor of weaning failure. Intensive Care Med 2012;38:2017-25. [Crossref] [PubMed]
- Liu L, Liu H, Yang Y, et al. Neuroventilatory efficiency and extubation readiness in critically ill patients. Crit Care 2012;16:R143. [Crossref] [PubMed]
- Brochard L. Intrinsic (or auto-) PEEP during controlled mechanical ventilation. Intensive Care Med 2002;28:1376-8. [Crossref] [PubMed]
- Jubran A, Tobin MJ. Pathophysiologic basis of acute respiratory distress in patients who fail a trial of weaning from mechanical ventilation. Am J Respir Crit Care Med 1997;155:906-15. [Crossref] [PubMed]
- Del Negro CA, Funk GD, Feldman JL. Breathing matters. Nat Rev Neurosci 2018;19:351-67. [Crossref] [PubMed]
- Chittawatanarat K, Orrapin S, Jitkaroon K, et al. An Open Label Randomized Controlled Trial to Compare Low Level Pressure Support and T-piece as Strategies for Discontinuation of Mechanical Ventilation in a General Surgical Intensive Care Unit. Med Arch 2018;72:51-7. [Crossref] [PubMed]
- Vallverdu I, Calaf N, Subirana M, et al. Clinical characteristics, respiratory functional parameters, and outcome of a two-hour T-piece trial in patients weaning from mechanical ventilation. Am J Respir Crit Care Med 1998;158:1855-62. [Crossref] [PubMed]
- Epstein SK, Ciubotaru RL. Independent effects of etiology of failure and time to reintubation on outcome for patients failing extubation. Am J Respir Crit Care Med 1998;158:489-93. [Crossref] [PubMed]
- Thille AW, Harrois A, Schortgen F, et al. Outcomes of extubation failure in medical intensive care unit patients. Crit Care Med 2011;39:2612-8. [Crossref] [PubMed]
- Karanjia N, Nordquist D, Stevens R, et al. A clinical description of extubation failure in patients with primary brain injury. Neurocrit Care 2011;15:4-12. [Crossref] [PubMed]
- dos Reis HF, Almeida ML, da Silva MF, et al. Association between the rapid shallow breathing index and extubation success in patients with traumatic brain injury. Rev Bras Ter Intensiva 2013;25:212-7. [Crossref] [PubMed]
- Asehnoune K, Mrozek S, Perrigault PF, et al. A multi-faceted strategy to reduce ventilation-associated mortality in brain-injured patients. The BI-VILI project: a nationwide quality improvement project. Intensive Care Med 2017;43:957-70. [Crossref] [PubMed]
- Anderson CD, Bartscher JF, Scripko PD, et al. Neurologic Examination and Extubation Outcome in the Neurocritical Care Unit. Neurocritical Care 2011;15:490-7. [Crossref] [PubMed]
- McCredie VA, Ferguson ND, Pinto RL, et al. Airway Management Strategies for Brain-injured Patients Meeting Standard Criteria to Consider Extubation. A Prospective Cohort Study. Ann Am Thorac Soc 2017;14:85-93. [Crossref] [PubMed]
- Navalesi P, Frigerio P, Moretti MP, et al. Rate of reintubation in mechanically ventilated neurosurgical and neurologic patients: evaluation of a systematic approach to weaning and extubation. Crit Care Med 2008;36:2986-92. [Crossref] [PubMed]
- Qureshi AI, Suarez JI, Parekh PD, et al. Prediction and timing of tracheostomy in patients with infratentorial lesions requiring mechanical ventilatory support. Crit Care Med 2000;28:1383-7. [Crossref] [PubMed]
- Sklar MC, Burns K, Rittayamai N, et al. Effort to Breathe with Various Spontaneous Breathing Trial Techniques. A Physiologic Meta-analysis. Am J Respir Crit Care Med 2017;195:1477-85. [Crossref] [PubMed]
- Lindqvist J, van den Berg M, van der Pijl R, et al. Positive End-Expiratory Pressure Ventilation Induces Longitudinal Atrophy in Diaphragm Fibers. Am J Respir Crit Care Med 2018;198:472-85. [Crossref] [PubMed]
- Pinsky MR, Summer WR, Wise RA, et al. Augmentation of cardiac function by elevation of intrathoracic pressure. J Appl Physiol Respir Environ Exerc Physiol 1983;54:950-5. [Crossref] [PubMed]
- Burns KEA, Soliman I, Adhikari NKJ, et al. Trials directly comparing alternative spontaneous breathing trial techniques: a systematic review and meta-analysis. Crit Care 2017;21:127. [Crossref] [PubMed]
(English Language Editor: R. Scott)