Sinus thrombosis—do animal models really cover the clinical syndrome?
Cerebral venous thrombosis in humans
While cerebral venous sinus thrombosis (CVST) affects children and young adults in particular, it can potentially occur in all age groups. Due to specific predispositions, such as oral contraceptives, pregnancy and the postpartum period, CVST predominantly occur in women with a sex ratio of 3:1 (1,2).
Other common risk factors include hereditary and acquired thrombophilia. The list of known causes or predispositions is long and summarized in Table 1. On the whole, the aetiology of CVST is often multi-factorial and despite a large number of manifest causes, it remains unexplained in approx. 20-30% of cases. In contrast to typical arterial thrombosis, which can suddenly lead to vascular occlusion and often causes clearly defined clinical symptoms for the vessels in question, one third of venous thromboses start off chronically, one third subacutely and one third acutely due to an imbalance in simultaneously occurring prothrombotic and thrombolytic processes.
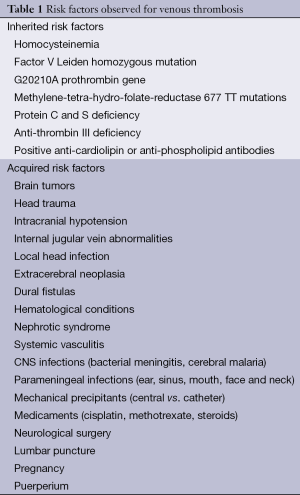
Full table
The “International Study on Cerebral Vein and Dural Sinus Thrombosis (ISCVT)”, published in 2004, with a cohort size of 624 patients, revealed that the mortality of the disease is clearly below 10%; in most cases caused by brain edema. At the end of the 16-month observation period 79% of patients made a complete recovery. Only a small percentage of patients remained disabled. The unfavourable prognostic signs identified were age >37 years, male gender, neuropsychological deficits on admission, clinically severe impaired levels of consciousness on admission (GCS <9) and thrombosis of the internal cerebral veins. Total mortality for the age group >65 years lay at around 27%, and was clearly higher than for the age group <65 years (7%) (1). The recurrence rate is very low; at around 2% per annum, it is comparable to the recurrence rate for deep vein thrombosis (1,3).
The clinical symptoms of CVST are extremely variable and exhibit a chronic to hyperacute dynamics. The symptoms range from asymptomatic cases or isolated headaches up to severe neurological deficits or coma (Table 2). Headaches are the most common symptom of CVST, occurring in approx. 90% of cases (4). In addition to headaches, seizures and focal neurological deficits occur; in 20-40% of cases the syndrome manifests as an isolated increase in intracranial pressure (ICP) with headaches, nausea and vomiting, and bilateral papilloedema (5). Finally, also consistent with thrombosis of the inner cerebral veins with bilateral involvement of the thalamus, symptoms ranging from the initial clouding of consciousness to coma may arise.
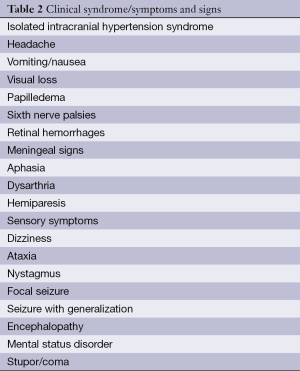
Full table
Systemic anticoagulation with unfractioned heparin is recommended in American and European Guidelines as first line treatment (6,7), based on a single randomized trial. Low-molecular-weight heparin (LMWH) was not superior to placebo in a Dutch trial (8), however, ISCVT data indicate that LMWH may be safer than unfractioned heparins. In spite of an optimum oral anticoagulation the rate of morbidity and mortality in patients suffered from CVST counts about 13.4% (1). Due to the high morbidity and mortality rate alternative aggressive treatment strategies such as endovascular thrombolysis or catheter-based mechanical thrombectomy become the further focus of clinical trials. Due to expected poor outcomes and increased ICP in patients with severe CVST such as multiple sinus occlusions including the jugular veins without associated hemorrhage endovascular thrombolysis may be an alternative primary treatment (9-11).
In small case series, mechanical thrombectomy serves as potential alternative or supplement to endovascular thrombolysis (11).
In case of impending risk of transtentorial herniation or brainstem compression secondary to massively increased ICP decompressive craniectomy may be life-saving measure (12). Studies on decompressive craniotomy in CVST showed fairly good outcomes with more than 50% of patients remaining functionally independent.
Objectives of animal models in the CVST
The contribution of experimental research for a better knowledge of physiological and pathophysiological changes in cerebral vascular system is of high importance. Animal models are of great value for further investigation of cerebral venous thrombosis and for the experimental evaluation of treatment strategies.
The pathophysiological processes in the context of cerebral vein and sinus thrombosis are complex and they are not yet fully known. The severity of the clinical symptoms does not appear to be correlated with the severity of the CVST. As Röttger et al. successfully demonstrated in 2005, over half of patients with venous infarctions go into full remission or experience clear reduction in the volume of the lesion (13). While this recovery is faster in patients who exhibit a rapid recanalisation of the affected sinuses using MRI criteria, ultimately, it does not depend on recanalisation or on a persistent occlusion. Nor is there a clinical difference regarding outcome depending on recanalisation or persistent occlusion (14). This allows for the conclusion that obviously, collateral vessels are adapted during the process or angiogenesis takes place or both. The vessels or newly formed vessels studied here are of a magnitude that cannot be depicted by the usual imaging procedures in humans. Autopsies on patients who died from sinus thrombosis are performed far too seldom to provide systematic insights. Besides, the localisation and the stage of the venous thrombosis need to be standardised, in order to assess the changes to the vascular system caused by them in a scientific manner.
Pathophysiology
During the initial stage the intravascular thrombus exhibits a laminated structure, with layers of aggregated and degranulated thrombocytes, with infiltration of leukocytes and layers of fibrin. After 5-10 days the thrombus takes on a homogeneous, hyaline structure.
At a later stage (8-20 days) the thrombus will be organised with the growth of fibroblasts and capillaries and endothelium-coated gap junctions. The thrombus undergoes conversion into a vascularised, fibrous mass which may lead to partial recanalisation due to clot retraction (15,16). In symptomatic patients the superior sagittal sinus (SSS), transverse sinuses and the sigmoid sinuses are the most commonly affected structures, while thrombosis of the cortical veins and the inner cerebral veins occur less frequently (4). The formation of venous thrombi, especially if they spread into bridging veins and cortical veins, causes a drop in cerebral perfusion pressure, with a simultaneous increase in ICP (17). In deed venous infarcts have only been observed when either two or more cortical veins or the inner cerebral venous system have been affected (18). As a result, the local cerebral blood flow decreases, which lead to the formation of a cytotoxic oedema and also lead to consecutive venous infarction. After the blood-brain barrier has been disrupted and capillary filtration increases, a vasogenic oedema will arise, resulting in congestive bleeding.
Animal models
An animal model of spontaneously occurring CVST does not exist. Also, there are no animal strains which are CVST-prone. To study CVST in a standardized animal model precludes more or less invasive induction of sinus or cortical vein thrombosis with chances of collateral damage due to operation or intervention the smaller the animal model chosen.
With only a few exemptions, most models did not study long-term effects of CVST, but animals were euthanised after a short investigation period of only a few hours.
In contrast to ischemic cerebral infarction, there are only a few animal experiment studies into CVST (Table 3). Those few studies were carried out on rats, pigs, cats, gerbils and rabbits (17,20,22,39-46). For the most part, these methods were invasive interventions and they often led to iatrogenic parenchymal defects in the brain. Furthermore, the transfer of results to the clinical situation of these studies was limited, because it was not possible to influence the duration of the thrombosis or the recanalisation. The efficacy of therapeutic options and their assessment could only be investigated to a limited degree. The purpose of the studies was the modification of local cerebral perfusion due to CVST, using laser Doppler flowmetry or the hydrogen clearance method, oximetric measurements of local oxygen saturation, measuring ICP using parenchymal and ventricular probes and fluorescein angiography to identify disruptions of the blood-brain barrier.
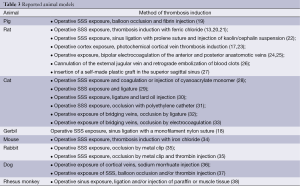
Full table
All of these studies used the option of histopathological regeneration. Angiogenesis was studied in animal models of arteriovenous fistula which were, for example, created by operative anastomosis of the carotid artery and the jugular vein, partly with the induction of a sinus thrombosis. Various forms of thrombosis induction were used (47,48). Wang et al. produced sinus thrombosis in pigs using thrombin injections and balloon dilatation of SSS (45), Stracke et al. used human blood clots injected into the SSS of pigs and studied them using MR imaging (49), Schaller et al. induced sinus thrombosis by way of a ligature on the posterior section of the SSS in cats (29), Röttger et al. using a topical application of Iron (III) chloride in rats (20). Furthermore, other models with occlusion of the SSS or cortical veins by local injections of thrombogenic materials such as cyanoacrylate (21) or kaolin cephalin (40,42) were used. All of the above-mentioned models are reversible models which revealed that the clinic, diagnostics and use of therapeutic options could only be assessed to a limited extent.
A major drawback of the above mentioned models is that the thrombus does not spontaneously propagate into the cortical veins causing larger venous infarcts. Nakase et al. (17) induced cortical vein thrombosis by direct illumination in a photothrombotic dye model with Bengal rose. However, concerns exist that direct illumination of the cortical surface may cause cortical damage by simply activating the dye in arterioles rather than by pure venous circulation compromise.
Currently, Li et al. (46) developed a new model of SSS using a combination of Ligation, injection of thrombin into the SSS and temporary carotid artery occlusion. Li et al. demonstrated, beside SSS thrombosis, affected cortical venous thrombosis and intraparenchymal hemorrhage. The main point of this model is a more accurately demonstration of the pathophysiologic circumstances in CVST than previous studies. Similar to human subjects this model creates cortical vein thrombosis, reveals thrombus, inflammatory cells, infarction and intraparenchymal haemorrhage. The limitation of this model is that the study of therapeutic treatment is in case of permanent ligation of the SSS is difficult. The permanent occlusion by ligation of the SSS leading to permanent alteration of blood flow is not physiologic. Furthermore temporary carotid artery ligation leads to cerebral hypoperfusion and changes in the regional CBF.
Conclusions
Animal models contributed significantly to our understanding of the pathophysiology of CVST.
There are currently a number of established animal models for studying the pathophysiological processes involved in CVST (Table 3). In contrast to ischemic cerebral infarction, there are only a few animal experiment studies into cerebral veins and sinus thrombosis. Various forms of thrombosis induction were used. Wang et al. induced the sinus thrombosis with invasive, catheter-based thrombin injections and balloon dilatation in pigs and observed the effects of these thrombi over seven days, during which time one animal died and 4 exhibited occlusion of the SSS (45). Complications in the sense of a cerebral oedema, haemorrhage infarction could be depicted using MRT. Stracke et al. used human blood clots in pigs and studied then using MR imaging (49), Schaller et al. induced sinus thrombosis by way of a ligature on the posterior section of SSS in cats (29), Röttger et al. with topical application of Iron (III) chloride in rats (20). Iron (III) chloride was first used by Kurz et al. and described in connection with models of arterial thrombosis formation (21). All of the above-mentioned models are reversible models which revealed that the clinic, diagnostics and use of therapeutic options could only be assessed to a limited extent.
In these models the changes in the regional cerebral blood flow (rCBF) were determined using laser Doppler flowmetry or the hydrogen clearance method. Other techniques used in these models include oximetric measurement of local oxygen saturation, measurement of ICP using parenchymal and ventricular probes and fluorescein angiography to identify disruptions of the blood-brain barrier. All previous studies used the option of histopathological regeneration.
For the most part, these methods were invasive interventions and they often led to iatrogenic parenchymal defects in the brain. Furthermore, the transferability of these methods was very limited, as it was not possible to influence the duration of the thrombosis or the recanalisation. None of these models could account for permanent occlusion under clinical observation or changes to vascular structure beyond seven days. In a further development of the animal model of Röttger et al. (20), it was possible to demonstrate a sustained occlusion of the SSS occlusion for a period of 6 weeks in the living animal and allows for the study of pathophysiological conditions following permanent occlusion over longer periods of time (19). In this study we were able to demonstrate neoangiogenesis 6 weeks after thrombus induction within the lumen of the thrombosed SSS as a network of small venules which connect to cortical veins. These newly formed vessels coincide with the locally strong VEGF expression in the organized thrombus consistent with neoangiogenesis. The most important finding was an increase of the cortical venous volume fraction above control levels after 6 weeks, although only traces of VEGF expression could be found in the parasagittal region. This study examines angiogenesis in a standardized, non-lethal rat model of persistent SSS thrombosis. This model allows for standardization of the time of onset and control of physiological variables not possible in human case series. Micro- and nano-CT was used to study the venous vascular changes and demonstrated Neoangiogenesis as the main reason for the good clinical outcome and the reduced final infarct volume (19).
All of the animal models encountered complications such as venous infarctions and cerebral oedema. The efficacy of therapeutic options and their assessment could only be investigated to a limited degree.
All animal models with above mentioned Limitations do not carry the morbidity and mortality associated with cortical vein thrombosis seen in human patients.
The creation of an ideal and reliable model comprises simultaneously inducted cortical venous thrombosis, infarct and hemorrhage with consecutive clinical symptoms illustrating the pathophysiologic changings in CVST in humans allowing options for the study of therapeutic strategies.
Acknowledgements
Disclosure: The authors declare no conflict of interest.
References
- Ferro JM, Canhão P, Stam J, et al. Prognosis of cerebral vein and dural sinus thrombosis: results of the International Study on Cerebral Vein and Dural Sinus Thrombosis (ISCVT). Stroke 2004;35:664-70. [PubMed]
- Cantú C, Barinagarrementeria F. Cerebral venous thrombosis associated with pregnancy and puerperium. Review of 67 cases. Stroke 1993;24:1880-4. [PubMed]
- Gosk-Bierska I, Wysokinski W, Brown RD Jr, et al. Cerebral venous sinus thrombosis: Incidence of venous thrombosis recurrence and survival. Neurology 2006;67:814-9. [PubMed]
- Bousser MG, Ferro JM. Cerebral venous thrombosis: an update. Lancet Neurol 2007;6:162-70. [PubMed]
- Biousse V, Ameri A, Bousser MG. Isolated intracranial hypertension as the only sign of cerebral venous thrombosis. Neurology 1999;53:1537-42. [PubMed]
- Einhäupl K, Stam J, Bousser MG, et al. EFNS guideline on the treatment of cerebral venous and sinus thrombosis in adult patients. Eur J Neurol 2010;17:1229-35. [PubMed]
- Saposnik G, Barinagarrementeria F, Brown RD Jr, et al. Diagnosis and management of cerebral venous thrombosis: a statement for healthcare professionals from the American Heart Association/American Stroke Association. Stroke 2011;42:1158-92. [PubMed]
- Stolz E, Trittmacher S, Rahimi A, et al. Influence of recanalization on outcome in dural sinus thrombosis: a prospective study. Stroke 2004;35:544-7. [PubMed]
- Dentali F, Squizzato A, Gianni M, et al. Safety of thrombolysis in cerebral venous thrombosis. A systematic review of the literature. Thromb Haemost 2010;104:1055-62. [PubMed]
- Qiu Z, Sang H, Dai Q, et al. Endovascular treatments for cerebral venous sinus thrombosis. J Thromb Thrombolysis 2015. [Epub ahead of print]. [PubMed]
- Viegas LD, Stolz E, Canhão P, et al. Systemic thrombolysis for cerebral venous and dural sinus thrombosis: a systematic review. Cerebrovasc Dis 2014;37:43-50. [PubMed]
- Raza E, Shamim MS, Wadiwala MF, et al. Decompressive surgery for malignant cerebral venous sinus thrombosis: a retrospective case series from Pakistan and comparative literature review. J Stroke Cerebrovasc Dis 2014;23:e13-22. [PubMed]
- Röttger C, Trittmacher S, Gerriets T, et al. Reversible MR imaging abnormalities following cerebral venous thrombosis. AJNR Am J Neuroradiol 2005;26:607-13. [PubMed]
- Stolz E, Trittmacher S, Rahimi A, et al. Influence of recanalization on outcome in dural sinus thrombosis: a prospective study. Stroke 2004;35:544-7. [PubMed]
- Cervós-Navarro J, Kannuki S, Matsumoto K. Neuropathological changes following occlusion of the superior sagittal sinus and cerebral veins in the cat. Neuropathol Appl Neurobiol 1994;20:122-9. [PubMed]
- Kannuki S, Cervós-Navarro J, Matsumoto K, et al. Brain edema in experimental superior sagittal sinus occlusion. Adv Neurol 1990;52:546. [PubMed]
- Nakase H, Heimann A, Kempski O. Alterations of regional cerebral blood flow and oxygen saturation in a rat sinus-vein thrombosis model. Stroke 1996;27:720-7; discussion 728. [PubMed]
- Miyamoto K, Heimann A, Kempski O. Microcirculatory alterations in a Mongolian gerbil sinus-vein thrombosis model. J Clin Neurosci 2001;8 Suppl 1:97-105. [PubMed]
- Stolz E, Yeniguen M, Kreisel M, et al. Angioarchitectural changes in subacute cerebral venous thrombosis. A synchrotron-based micro- and nano-CT study. Neuroimage 2011;54:1881-6. [PubMed]
- Röttger C, Bachmann G, Gerriets T, et al. A new model of reversible sinus sagittalis superior thrombosis in the rat: magnetic resonance imaging changes. Neurosurgery 2005;57:573-80; discussion 573-80. [PubMed]
- Kurz KD, Main BW, Sandusky GE. Rat model of arterial thrombosis induced by ferric chloride. Thromb Res 1990;60:269-80. [PubMed]
- Frerichs KU, Deckert M, Kempski O, et al. Cerebral sinus and venous thrombosis in rats induces long-term deficits in brain function and morphology--evidence for a cytotoxic genesis. J Cereb Blood Flow Metab 1994;14:289-300. [PubMed]
- Kimura R, Nakase H, Tamaki R, et al. Vascular endothelial growth factor antagonist reduces brain edema formation and venous infarction. Stroke 2005;36:1259-63. [PubMed]
- Cokluk C, Aydin K, Korkmaz A, et al. A model of unilateral cerebral anterior and posterior anastomotic vein occlusion in the rat. Minim Invasive Neurosurg 2005;48:149-53. [PubMed]
- Cokluk C, Aydin K, Yemisci M, et al. Cortical anastomotic veins occlusion in the rat including the assessment of cerebral swelling. J Neurosci Methods 2006;156:203-10. [PubMed]
- Kurokawa Y, Hashi K, Okuyama T, et al. Regional ischemia in cerebral venous hypertension due to embolic occlusion of the superior sagittal sinus in the rat. Surg Neurol 1990;34:390-5. [PubMed]
- Yang H, Meng Z, Zhang C, et al. Establishing a new rat model of central venous sinus thrombosis and analyzing its pathophysiological and apoptotic changes. J Neurosci Methods 2012;203:130-5. [PubMed]
- Gotoh M, Ohmoto T, Kuyama H. Experimental study of venous circulatory disturbance by dural sinus occlusion. Acta Neurochir (Wien) 1993;124:120-6. [PubMed]
- Schaller B, Graf R, Wienhard K, et al. A new animal model of cerebral venous infarction: ligation of the posterior part of the superior sagittal sinus in the cat. Swiss Med Wkly 2003;133:412-8. [PubMed]
- Woolf AL. Experimentally produced cerebral venous obstruction. J Pathol Bacteriol 1954;67:1-16. [PubMed]
- Kanaiwa H, Kuchiwaki H, Inao S, et al. Changes in the cerebrocortical capillary network following venous sinus occlusion in cats. Surg Neurol 1995;44:172-9; discussion 179-80. [PubMed]
- Romansky K, Stamenov B. Ultrastructural study of cerebral cortex and subcortical white matter following ligation of bridging veins in cats. Zentralbl Neurochir 1995;56:111-6. [PubMed]
- Sakaki T, Kakizaki T, Takeshima T, et al. Importance of prevention of intravenous thrombosis and preservation of the venous collateral flow in bridging vein injury during surgery: an experimental study. Surg Neurol 1995;44:158-62. [PubMed]
- BECK DJ, Russell DS. Experiments on thrombosis of the superior longitudinal sinus. J Neurosurg 1946;3:337-47. [PubMed]
- Kim DE, Schellingerhout D, Jaffer FA, et al. Near-infrared fluorescent imaging of cerebral thrombi and blood-brain barrier disruption in a mouse model of cerebral venous sinus thrombosis. J Cereb Blood Flow Metab 2005;25:226-33. [PubMed]
- Heinz ER, Geeter D, Gabrielsen TO. Cortical vein thrombosis in the dog with a review of aseptic intracranial venous thrombosis in man. Acta Radiol Diagn (Stockh) 1972;13:105-14. [PubMed]
- Sarwar M, Virapongse C, Carbo P. Experimental production of superior sagittal sinus thrombosis in the dog. AJNR Am J Neuroradiol 1985;6:19-22. [PubMed]
- Owens G, Stahlman G, Capps J, et al. Experimental occlusion of dural sinuses. J Neurosurg 1957;14:640-7. [PubMed]
- Ito K, Tsugane R, Ikeda A, et al. Cerebral hemodynamics and histological changes following acute cerebral venous occlusion in cats. Tokai J Exp Clin Med 1997;22:83-93. [PubMed]
- Röther J, Waggie K, van Bruggen N, et al. Experimental cerebral venous thrombosis: evaluation using magnetic resonance imaging. J Cereb Blood Flow Metab 1996;16:1353-61. [PubMed]
- Fries G, Wallenfang T, Kempski O, et al. Brain oedema and intracranial pressure in superior sagittal sinus balloon occlusion. An experimental study in pigs. Acta Neurochir Suppl (Wien) 1990;51:231-2. [PubMed]
- Ungersböck K, Heimann A, Kempski O. Cerebral blood flow alterations in a rat model of cerebral sinus thrombosis. Stroke 1993;24:563-9; discussion 569-70. [PubMed]
- Fries G, Wallenfang T, Hennen J, et al. Occlusion of the pig superior sagittal sinus, bridging and cortical veins: multistep evolution of sinus-vein thrombosis. J Neurosurg 1992;77:127-33. [PubMed]
- Gotoh M, Ohmoto T, Kuyama H. Experimental study of venous circulatory disturbance by dural sinus occlusion. Acta Neurochir (Wien) 1993;124:120-6. [PubMed]
- Wang J, Tan HQ, Li MH, et al. Development of a new model of transvenous thrombosis in the pig superior sagittal sinus using thrombin injection and balloon occlusion. J Neuroradiol 2010;37:109-15. [PubMed]
- Li G, Zeng X, Ji T, et al. A new thrombosis model of the superior sagittal sinus involving cortical veins. World Neurosurg 2014;82:169-74. [PubMed]
- Rothbart D, Awad IA, Lee J, et al. Expression of angiogenic factors and structural proteins in central nervous system vascular malformations. Neurosurgery 1996;38:915-24; discussion 924-5. [PubMed]
- Shin Y, Nakase H, Nakamura M, et al. Expression of angiogenic growth factor in the rat DAVF model. Neurol Res 2007;29:727-33. [PubMed]
- Stracke CP, Katoh M, Wiethoff AJ, et al. Molecular MRI of cerebral venous sinus thrombosis using a new fibrin-specific MR contrast agent. Stroke 2007;38:1476-81. [PubMed]