Lipopolysaccharide-induced inflammation in human peritoneal mesothelial cells is controlled by ERK1/2-CDK5-PPARγ axis
Introduction
Peritonitis is a common complication of peritoneal dialysis (PD), accounting for approximately 15% of mortality in patients with PD (1-3). It refers to inflammation of the peritoneum. Increased episodes of peritonitis in patients with PD are correlated with or are predictive of death (1).
Typical causes of peritonitis include spontaneous bacterial or fungal infection, visceral perforation or damage, inflammation, appendicitis, inflammatory bowel diseases, and feline infectious peritonitis virus (4-7). Widely used animal models of peritonitis include intraperitoneal inoculation of the gut bacterium Bacteroidesfragilis (B. fragilis), which secretes lipopolysaccharide (LPS) and LPS promotes inflammation by stimulating the production of interleukins (ILs) such as IL-1β, IL-6 and IL-8, tumor necrosis factor (TNF)-α via Toll-like receptor 2 (TLR2) activation (5). Research using LPS-induced peritonitis models has suggested that peroxisome proliferator-activated receptor (PPAR)γ plays crucial anti-inflammatory roles (8,9), with the expression of PPARγ exerting a protective effect against LPS-induced inflammation injury.
The anti-inflammatory effect of PPARγ is related to the inhibition of various inflammatory pathways, including the nuclear factor-kappa B (NF-κB) pathway (8,10). Activation of extracellular signal-regulated kinases 1/2 (ERK1/2) is associated with LPS-induced mastitis (11,12), acute lung injury (13), and proinflammatory cytokine release (14). Some studies have suggested the possibility that LPS modulates both the ERK1/2 and PPARγ signaling pathways, although there is little information on the association or interaction between these pathways in LPS-induced inflammation and peritonitis.
The interaction between cyclin-dependent kinase (CDK)5 and ERK/PPARγ, however, is known to control and enrich the roles of PPARγ in cellular modulation (15,16). In Alzheimer’s disease, LPS has been reported to induce tau-phosphorylation in a CDK5-dependent manner (17). The present study was performed to investigate the association of the ERK/CDK5 axis and PPARγ in LPS-induced peritonitis in vitro, with the aim of providing novel insights into the pathogenesis of LPS-induced peritonitis.
We present the following article in accordance with the MDAR reporting checklist (available at http://dx.doi.org/10.21037/atm-21-1623).
Methods
Cell culture and LPS induction
The simian virus 40 (SV40)-immortalized human peritoneal mesothelial cell line HMrSV5 (ATCC, USA, ATCC16880) was maintained in Dulbecco’s Modified Eagle Medium (DMEM, Hyclone, Logan, UT, USA) supplemented with 10% fetal-bovine serum (FBS) and different concentrations of LPS (0, 0.01, 0.05, 0.1, 0.5, 1.0, 5.0, 10, and 100 µg/mL; Sigma-Aldrich; Merck KGaA, Darmstadt, Germany)at 37 °C with 5% CO2 for 12 hours. Further, cells were cultured with the optimal LPS concentration of 1 µg/mL for 3, 6, 12, 24, 36, 48, and 72 hours.
Cell transfection and treatment
CDK5 small interfering RNA (siRNA) and scrambled sequence were purchased from Genechem Co. Ltd. (Shanghai, China) and transfected into HPMCs using Lipofectamine 2000 reagent (Santa Cruz, CA, USA) according to the manufacturer’s instructions. The target sequence of CDK5-siRNAs was #266: 5'-AAUCUUUUCCAGUUUCUCGUA-3', #406: 5'-ACAGAAACAGGGUUUUCUCAU-3', #809: 5'-AAUAUCGUGCUGUAGUAUCAU-3'. Cells were incubated in DMEM with 5% CO2 at 37 °C for 24 hours.
To inhibit ERK signaling, HPMCs were treated with 1 µM AZD0364 (Sigma-Aldrich; Merck KGaA) (18) for 4 hours, followed by LPS treatment. Each experiment was performed with 3 independent replications.
Enzyme-linked immunosorbent assay
The contents of IL-1β, IL-6, and IL-12 in the cellular medium were determined using commercial ELISA kits purchased from Shanghai Enzyme-linked Biotechnology Co., Ltd., (Shanghai, China). A Thermo microplate reader (Thermo Fisher Scientific Inc., Rockford, IL, USA) was used to determine the optical density at 450 nm. Each experiment was performed with 3 independent replications.
Quantitative real-time PCR analysis
Total RNA extraction was performed with TRIzol reagent (TaKaRa, Tokyo, Japan), and was followed by the synthesis of first-strand complementary DNA (cDNA) using a Bestarq PCR RT Kit (DBI Bioscience, Ludwigshafen, Germany). For PCR amplification, 1 µg DNA template was used with primers purchased from Sangon (Shanghai, China) and DBIBestar® SYBRGreen qPCR master Mix (DBI Bioscience). PCR amplification was conducted on an Agilent Stratagene Mx3000P Real-time PCR machine (DBI Bioscience) with the following reaction cycles: 95 °C for 2 minutes; 94 °C for 20 seconds, 58 °C for 20 seconds, and 72 °C for 20 seconds, for 40 cycles. Relative expression levels were calculated using the 2−ΔΔCt method, with glyceraldehyde 3-phosphate dehydrogenase (GAPDH) serving as an internal control. The sequence of primers used was CDK5 forward: 5'-GGAAGGCACCTACGGAACTG-3', CDK5 reverse: 5'-GGCACACCCTCATCATCGT-3'.
Co-immunoprecipitation assay
Cells were treated with LPS for 12 hours. Interaction between CDK5 and ERK1/2 was detected by co-immunoprecipitation (co-IP) assay using a Pierce® Co-IP Kit (Thermo Scientific Inc.; #26149). Total protein (1 mg) from the lysate was mixed with 2 µg of ERK1/2 antibody in a column at 4 °C overnight. The immune complexes were analyzed by western blot with the indicated antibodies. Immunoglobulin G (IgG) was employed as the normal control.
Western blot analysis
Protein lysates were isolated, quantified, and electrophoretically fractionated by sodium dodecyl sulfate-polyacrylamide gel electrophoresis (SDS-PAGE) using 10% gel (Sangon), and then transferred onto Whatman polyvinylidene difluoride (PVDF) membranes (Millipore, Whatman, Germany) according to standard methods. The membranes were blocked with 5% skim milk and tris-buffered saline (Sigma-Aldrich; Merck KGaA) at 4 °C overnight. Then, the membranes were subjected to primary antibody incubation with antibodies against CDK5 (1:1,000; Cell Signaling Technology, CST, Danvers, MA, USA), ERK1/2 (1:1,000; CST), p-ERK1/2 (1:1,000; CST), PPARγ (1:1,000; CST), p-PPARγSer273 (1:1,000; CST), and GAPDH (1:10,000; Boster Biotechnology, Wuhan, China) at 4 °C overnight. Incubation with secondary antibody (horseradish peroxidase goat anti-rabbit/mouse IgG; 1:20,000; Boster Biotechnology) was conducted at room temperature for 40 minutes. An enhanced chemiluminescence (ECL) system (Millipore) was used for signal detection, and the Image-Pro Plus 6.0 software analysis system (Media Cybernetics, Inc., Bethesda, MD, USA) was used to analyze protein signals. Data were presented as fold change, meaning the expression of the detected protein relative to the control protein. Each detection was replicated for 3 times.
Statistical analysis
All data were expressed as mean ± standard deviation (SD) of 3 replicates. Statistical analyses were performed with SPSS 20.0 software (IBM, USA). The Student’s t-test was employed to analyze differences between groups. Statistical significance was recognized as P<0.05.
Results
LPS induced dose-dependent inflammation and PPARγ phosphorylation
First, we determined whether LPS could dose-dependently induce the upregulation of inflammatory cytokines (IL-1β, IL-6, and IL-12) in HPMCs. ELISA assay demonstrated that treatment with more than 0.05 µg/mL LPS for 12 hours significantly elevated the contents of IL-1β, IL-6, and IL-12 compared with the control (0 µg/mL LPS; P<0.01, Figure 1A). No continuous or obvious increase in IL-1β and IL-6 was induced by 5 µg/mL LPS, while 10 µg/mL LPS reversed the increasing trend in the contents of the cytokines. Similar trends were observed for CDK5 expression detected by qRT-PCR (Figure 1B) and for PPARγSer273 phosphorylation detected by western blot (Figure 1C). Therefore, 1 µg/mL LPS induced the highest levels of inflammation, along with the highest levels of CDK5 expression and PPARγ phosphorylation.
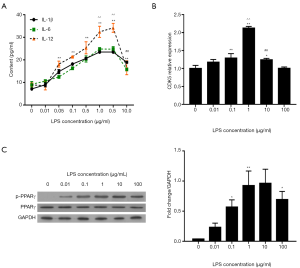
LPS induced time-dependent inflammation and PPARγ phosphorylation
Next, we tested whether LPS could induce the upregulation of inflammatory cytokines (IL-1β, IL-6, and IL-12) in HPMCs in a time-dependent manner. For this end, 1 µg/mL LPS was employed as the optimal concentration. Under treatment times up to 24 hours, LPS induced time-dependent increases in the expression levels of IL-1β, IL-6, and IL-12 in HPMC cells, as detected by ELISA (Figure 2A); however, treatment times longer than 24 hours resulted in comparative reductions in the contents of IL-1β, IL-6, and IL-12 to compare with 12 hours (P<0.01). LPS induced the highest levels of CDK5 mRNA expression (Figure 2B) and PPARγSer273 phosphorylation (Figure 2C) at 6 hours post stimulation. Interestingly, 3 hours of LPS treatment caused obvious increases in CDK5 expression and PPARγ phosphorylation, although a similar trend was not observed in the expression levels of IL-1β, IL-6, and IL-12 (Figure 2A,B). These data showed that CDK5 expression and PPARγ phosphorylation increased in the early stage of LPS stimulation, which was followed later by the elevated production of inflammatory factors. An long treatment time more than 12 hours significantly decreased the expression levels of CDK5 mRNA and PPARγ phosphorylation (P<0.01 for 12 vs. 6 hours; Figure 2B,C). However, we chose 12 hours as the treatment time for further experiments based on the inflammation profiles.
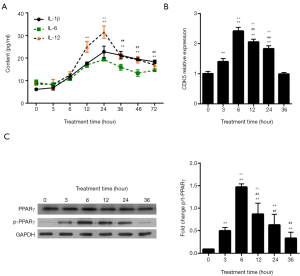
LPS activated ERK1/2 and increased interaction between ERK1/2 and CDK5
ERK1/2 activation has been reported to be related to LPS-induced inflammation (19). To identify the association between ERK1/2 and CDK5 in LPS-treated HPMC cells, we examined the effect of LPS on the activation of ERK1/2. The results showed that LPS (1 µg/mL, 12 hours) increased the mRNA and protein expression of CDK5, as well as ERK1/2 phosphorylation (Figure 3A,B). Furthermore, using a co-IP assay, we observed the interaction between CDK5 and ERK1/2 proteins, which was strengthened by the administration of LPS (Figure 3C). Together, these results suggested that LPS activated ERK1/2 signaling and interaction between ERK1/2 and CDK5 proteins.
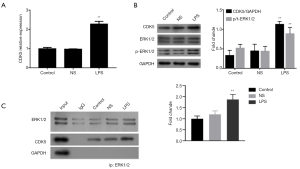
ERK1/2 inhibition prevented LPS-induced inflammation and PPARγ phosphorylation
Subsequently, we investigated whether ERK1/2 was involved in the LPS-induced changes in CDK5 expression and PPARγ phosphorylation in HPMC cells. For this purpose, ERK1/2 inhibitor AZD0364 (1 µM) (19) was used in combination with LPS treatment. Our results showed that AZD0364 significantly decreased the LPS-induced IL-1β, IL-6, and IL-12 elevation (P<0.01, Figure 4A), CDK5 expression and ERK1/2 phosphorylation (P<0.01, Figure 4B), and PPARγ phosphorylation (P<0.01, Figure 4C), suggesting that ERK1/2 functioned upstream of CDK5 and PPARγ during LPS-induced inflammation.
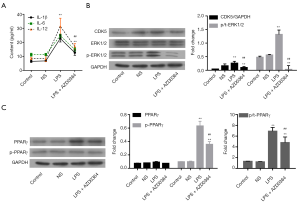
CDK5 siRNA prevented LPS-induced inflammation and PPARγ phosphorylation
To investigate the relationship of CDK5 with ERK1/2 and PPARγ in LPS-induced inflammation, we knocked down the expression of CDK5 in HPMC cells with its siRNAs (siRNA-266/809). As shown in Figure 5A, the expression of CDK5 was significantly inhibited in siRNA-transfected cells. Our subsequent results showed that siRNA-809 inhibited IL-1β, IL-6, and IL-12 production (P<0.01, Figure 5B), CDK5 expression (P<0.01, Figure 5C), and PPARγ phosphorylation (P<0.01, Figure 5D), but had no effect on ERK1/2 phosphorylation (Figure 5C). These results indicated that CDK5 acted as downstream of ERK1/2 and upstream of PPARγ during LPS-induced inflammation and that an ERK1/2-CDK5-PPARγ axis was implicated in this process.
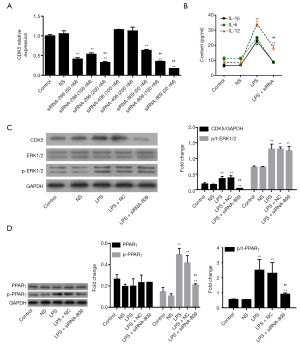
Discussion
The present study demonstrated that LPS induced inflammation in HPMCs in a dose- and time-dependent manner and that an axis involving ERK1/2, CDK5, and PPARγ was implicated in this process. Our results demonstrated the crucial role of an ERK/CDK5 axis in controlling LPS-induced inflammation via the modulation of PPARγ phosphorylation in HPMCs.
Peritonitis is an inflammatory condition which is commonly caused by spontaneous bacterial and fungal infections (4-7). Bacterial LPS typically causes inflammation via stimulating the production of proinflammatory cytokines such as IL-1β, IL-6, IL-8, and TNF-α (5). This axis is mediated or controlled by the TLR2, PPARγ, ERK1/2, and NF-κB pathways (5,8-10,14). The inhibition of LPS-induced production of TNF-α and IL-6, and inflammatory responses has been shown to have a potent anti-inflammatory effect (20,21). In rat model of peritonitis, the activation of PPARγ was found to ameliorate LPS-induced peritoneal deterioration (8). The activation of the ERK1/2 and NF-κB pathways is associated with LPS-induced mastitis and acute respiratory distress syndrome via the promotion of proinflammatory cytokine production (11,13,14). PPARγ activation contributes to reducing NF-κB and ERK1/2-mediated secretion of proinflammatory cytokines (22-25). In our present study, we demonstrated an association of LPS-induced inflammation with CDK5-mediated phosphorylation and activation of ERK1/2.
By treating HPMCs with different concentrations of LPS, we found that treatment with 1 µg/mL LPS for 12 hours was the optimal experimental design in vitro. At 12 hours, 1 µg/mL LPS treatment induced the most severe inflammatory status in HPMCs of any treatment time. Overdose of LPS or an long treatment time decreased the production of the inflammatory cytokines IL-1β, IL-6, and IL-12, as well as the levels of CDK5 expression and PPARγ phosphorylation (Figures 2 and 3). Of note, it is possible that CDK5 and PPARγ functioned in the early stage of LPS-induced inflammation and contributed to the subsequent production of inflammatory cytokines (Figure 2). Previous studies have reported that PPARγ is an anti-inflammatory ligand (8,9). Together with its agonists, PPARγ inhibits the production of monocytic inflammatory cytokines, including IL-1β, IL-6, and TNF-α, thus preventing inflammatory injury (26,27). However, some researchers have arrived at a different conclusion, reporting that PPARγ does not inhibit LPS-induced IL-6 or TNF-α production in macrophages (28). Therefore, it is possible that the progression of LPS-induced inflammation is promoted by PPARγ phosphorylation.
Our study demonstrated that LPS-induced production of IL-1β, IL-6, and IL-12, promoted ERK1/2 activation, and increased the levels CDK5 expression and PPARγ phosphorylation. The administration of the ERK1/2 inhibitor AZD0364 suppressed the expression of ERK1/2, CDK5, and p-PPARγ, therefore preventing LPS-induced inflammation. The knockdown of CDK5 by its siRNA suppressed p-PPARγ and LPS-induced inflammation, but did not affect ERK1/2 activation (Figures 4 and 5). These results indicated that an ERK1/2-CDK5-PPARγ axis was involved in LPS-induced inflammation.
The involvement of the ERK1/2 pathway in LPS-induced IL-6 production has been revealed previously by Yu et al. (14), who showed that LPS activated the ERK1/2 pathway to induce IL-6 mRNA expression (14). It has also been reported that IL-6-mediated proliferation and maintenance of stemness in mesenchymal stem cells require the activation of ERK1/2 (29). However, the direct participation of PPARγ in ERK1/2-mediated IL-6 expression has not been evidenced before. Our results demonstrated that the inhibition of ERK1/2 or knockdown of CDK5 prevented the LPS-induced production of the inflammatory cytokines IL-1β, IL-6, and IL-12, partly through the regulation of PPARγ phosphorylation.
Conclusions
Our study has demonstrated that LPS-induced inflammation in HPMCs could be prevented by inhibiting an ERK1/2-CDK5-PPARγ axis. Further study should be carried out to validate the essentiality of PPARγ in ERK1/2/CDK5 axis-modulated inflammation.
Acknowledgments
Funding: This project was funded by the National Natural Science Foundation of China (No.81800675), the Natural Science Foundation of Guangdong Province of China (No. 2016A030313420), The Science and Technology Program of Guangzhou (No.201804010066), and Guangzhou Medical Key Subject Construction Project (2017-2019).
Footnote
Reporting Checklist: The authors have completed the MDAR reporting checklist. Available at http://dx.doi.org/10.21037/atm-21-1623
Data Sharing Statement: Available at http://dx.doi.org/10.21037/atm-21-1623
Conflicts of Interest: All authors have completed the ICMJE uniform disclosure form (available at http://dx.doi.org/10.21037/atm-21-1623). The authors have no conflicts of interest to declare.
Ethical Statement: The authors are accountable for all aspects of the work in ensuring that questions related to the accuracy or integrity of any part of the work are appropriately investigated and resolved.
Open Access Statement: This is an Open Access article distributed in accordance with the Creative Commons Attribution-NonCommercial-NoDerivs 4.0 International License (CC BY-NC-ND 4.0), which permits the non-commercial replication and distribution of the article with the strict proviso that no changes or edits are made and the original work is properly cited (including links to both the formal publication through the relevant DOI and the license). See: https://creativecommons.org/licenses/by-nc-nd/4.0/.
References
- Boudville N, Kemp A, Clayton P, et al. Recent peritonitis associates with mortality among patients treated with peritoneal dialysis. J Am Soc Nephrol Jasn 2012;23:1398. [Crossref] [PubMed]
- Brown MC, Simpson K, Kerssens JJ, et al. Peritoneal dialysis-associated peritonitis rates and outcomes in a national cohort are not improving in the post-millennium (2000-2007). Perit Dial Int 2011;31:639-50. [Crossref] [PubMed]
- Li PK, Szeto CC, Piraino B, et al. ISPD Peritonitis Recommendations: 2016 Update on Prevention and Treatment. Perit Dial Int 2016;36:481-508. [Crossref] [PubMed]
- Thévenot T, Bureau C, Oberti F, et al. Effect of albumin in cirrhotic patients with infection other than spontaneous bacterial peritonitis. A randomized trial. J Hepatol 2015;62:822-30. [Crossref] [PubMed]
- Lobo LA, Benjamim CF, Oliveira AC. The interplay between microbiota and inflammation: lessons from peritonitis and sepsis. Clin Transl Immunology 2016;5:e90 [Crossref] [PubMed]
- Vennema H, Poland A, Foley J, et al. Feline infectious peritonitis viruses arise by mutation from endemic feline enteric coronaviruses. Virology 1998;243:150-7. [Crossref] [PubMed]
- Fiore M, Leone S. Spontaneous fungal peritonitis: Epidemiology, current evidence and future prospective. World J Gastroenterol 2016;22:7742-7. [Crossref] [PubMed]
- Zhang Y, Feng J, Qi W, et al. PPAR-γ agonist rosiglitazone ameliorates peritoneal deterioration in peritoneal dialysis rats with LPS-induced peritonitis through up-regulation of AQP-1 and ZO-1. Biosci Rep 2018;38:BSR20180009 [Crossref] [PubMed]
- Zhang YF, Zou XL, Wu J, et al. Rosiglitazone, a Peroxisome Proliferator-Activated Receptor (PPAR)-γ Agonist, Attenuates Inflammation Via NF-κB Inhibition in Lipopolysaccharide-Induced Peritonitis. Inflammation 2015;38:2105-15. [Crossref] [PubMed]
- Lin MH, Chen MC, Chen TH, et al. Magnolol ameliorates lipopolysaccharide-induced acute lung injury in rats through PPAR-γ-dependent inhibition of NF-kB activation. Int Immunopharmacol 2015;28:270-8. [Crossref] [PubMed]
- Li Y, Gong Q, Guo W, et al. Farrerol Relieve Lipopolysaccharide (LPS)-Induced Mastitis by Inhibiting AKT/NF-κB p65, ERK1/2 and P38 Signaling Pathway. Int J Mol Sci 2018;19:1770. [Crossref]
- Gong Q, Li Y, Ma H, et al. Peiminine protects against lipopolysaccharide-induced mastitis by inhibiting the AKT/NF-κB, ERK1/2 and p38 signaling pathways. Int J Mol Sci 2018;19:2637. [Crossref]
- Li Y, Zeng Z, Cao Y, et al. Angiotensin-converting enzyme 2 prevents lipopolysaccharide-induced rat acute lung injury via suppressing the ERK1/2 and NF-κB signaling pathways. Sci Rep 2016;6:27911. [Crossref] [PubMed]
- Yu K, Ma Y, Li X, et al. Lipopolysaccharide increases IL-6 secretion via activation of the ERK1/2 signaling pathway to up-regulate RANKL gene expression in MLO-Y4 cells. Cell Biol Int 2017;41:84. [Crossref] [PubMed]
- Banks AS, Mcallister FE, Camporez JOPG, et al. An ERK/Cdk5 axis controls the diabetogenic actions of PPARγ. Nature 2015;517:391-5. [Crossref] [PubMed]
- Bai X, Hou X, Tian J, et al. CDK5 promotes renal tubulointerstitial fibrosis in diabetic nephropathy via ERK1/2/PPARÎ3 pathway. Oncotarget 2016;7:36510-28. [Crossref] [PubMed]
- Kitazawa M, Oddo S, Yamasaki TR, et al. Lipopolysaccharide-induced inflammation exacerbates tau pathology by a cyclin-dependent kinase 5-mediated pathway in a transgenic model of Alzheimer’s disease. J Neurosci 2005;25:8843-53. [Crossref] [PubMed]
- Iain S, Mark JA, David MA, et al. Abstract 1647: Discovery of AZD0364, a potent and selective oral inhibitor of ERK1/2 that is efficacious in both monotherapy and combination therapy in models of NSCLC. Cancer Res 2018; [Crossref]
- Gu C, Song A, Zhang X, et al. Cloning of chrysanthemum high-affinity nitrate transporter family (CmNRT2) and characterization of CmNRT2.1. Sci Rep 2016;6:23462. [Crossref] [PubMed]
- Xie C, Kang J, Li Z, et al. The açaí flavonoid velutin is a potent anti-inflammatory agent: blockade of LPS-mediated TNF-α and IL-6 production through inhibiting NF-κB activation and MAPK pathway. J Nutr Biochem 2012;23:1184-91. [Crossref] [PubMed]
- Kumari A, Dash D, Singh R. Curcumin inhibits lipopolysaccharide (LPS)-induced endotoxemia and airway inflammation through modulation of sequential release of inflammatory mediators (TNF-α and TGF-β1) in murine model. Inflammopharmacology 2017;25:329-41. [Crossref] [PubMed]
- Carpentier C, Barbeau X, Azelmat J, et al. Lobaric acid and pseudodepsidones inhibit NF-κB signaling pathway by activation of PPAR-γ. Bioorg Med Chem 2018;26:5845-51. [Crossref] [PubMed]
- Shen Y, Sun Z, Guo X. Citral inhibits lipopolysaccharide-induced acute lung injury by activating PPAR-γ. Eur J Pharmacol 2015;747:45-51. [Crossref] [PubMed]
- Ge Z, Zhang M, Deng X, et al. Persimmon tannin promoted macrophage reverse cholesterol transport through inhibiting ERK1/2 and activating PPARγ both in vitro and in vivo. J Functional Foods 2017;38:338-48. [Crossref]
- Wu W, Wang S, Xu Z, et al. Betaine promotes lipid accumulation in adipogenic-differentiated skeletal muscle cells through ERK/PPARγ signalling pathway. Mol Cell Biochem 2018;447:137-49. [Crossref] [PubMed]
- Jiang C, Ting AT, Seed B. PPAR-gamma agonists inhibit production of monocyte inflammatory cytokines. Nature 1998;391:82-6. [Crossref] [PubMed]
- Cortez M, Carmo LS, Rogero MM, et al. A high-fat diet increases IL-1, IL-6, and TNF-α production by increasing NF-κB and attenuating PPAR-γ expression in bone marrow mesenchymal stem cells. Inflammation 2013;36:379-86. [Crossref] [PubMed]
- Thieringer R, Fenyk-Melody JE, Le GC, et al. Activation of peroxisome proliferator-activated receptor gamma does not inhibit IL-6 or TNF-alpha responses of macrophages to lipopolysaccharide in vitro or in vivo. J Immunol 2000;164:1046. [Crossref] [PubMed]
- Pricola KL, Kuhn NZ, Hana HS, et al. Interleukin-6 maintains bone marrow-derived mesenchymal stem cell stemness by an ERK1/2-dependent mechanism. J Cell Biochem 2009;108:577-88. [Crossref] [PubMed]
(English Language Editor: J. Reynolds)