Comprehensive analysis of prognostic predictors for patients with limited-stage small-cell lung cancer who underwent resection followed by adjuvant chemotherapy
Introduction
Lung cancer is one of the leading causes of cancer-related mortality and morbidity in China and around the world (1,2). Small-cell lung cancer (SCLC) is the most malignant subtype of lung cancer (3). Fifteen percent to 20% of lung cancer patients have SCLC (3). Further, nearly 70% of patients are diagnosed as being in the metastatic stage at their first visit (3). Under pathological classifications, SCLC has traditionally been categorized into the following two subtypes: (I) pure SCLC (p-SCLC); and (II) combined SCLC (c-SCLC). These categories are defined according to whether 1 or more other histological carcinomas, including lung adenocarcinoma, squamous cell carcinoma, or large cell carcinoma, are contained in the final section of the SCLC (4). Currently, a 2-stage classification system is used for the stage classification of SCLC. The two stages comprise limited-stage SCLC (LS-SCLC) and extensive-stage SCLC (ES-SCLC), and are defined by the extent status of the SCLC disease (5).
Accounting for almost 30% of SCLC cases, LS-SCLC is recognized as a disease confined to 1 hemithorax, and can be encompassed by a single radiation field (5,6). LS-SCLC is highly sensitive to chemotherapy, radiotherapy, and concurrent or sequential chemoradiotherapy; however, with a 5-year overall survival rate of only 20–25%, clinical survival outcomes are still disappointing (7,8). Chemoresistance and cancer metastasis frequently occur immediately after oncological treatment.
In recent years, surgical resection has become an important part of the multimodality of LS-SCLC treatments. Anatomic resection followed by chemotherapy or chemoradiotherapy has produced superior outcomes in the treatment of LS-SCLC when against surgery alone (9,10). However, previous research has focused on predicting the prognosis of patients with LS-SCLC who undergo resection followed by postoperative chemotherapy. With the widespread use of the next-generation sequencing (NGS) examinations with a 68-gene panel in resected LS-SCLC patients, we undertook a comprehensive analysis of the prognostic factors of resected LS-SCLC, and examined the clinicopathological characteristics in relation to genetic mutations detected by NGS. We present the following article in accordance with the STROBE reporting checklist (available at https://dx.doi.org/10.21037/atm-21-3353).
Methods
Patients
This retrospective study was approved by the Institutional Ethics Committee of Shanghai Chest Hospital (KS1992). The research process was conducted in accordance with the Helsinki Declaration (as revised in 2013). Written informed consent was not needed because of the retrospective nature of the research. All the patients received routine preoperative examinations, including enhanced chest computed tomography (CT) scanning, brain magnetic resonance imaging (MRI), abdominal ultrasound or enhanced abdominal CT scanning, and cervical ultrasound imaging. Positron emission tomography/computed tomography (PET/CT) and bronchoscopy were recommended as necessary. Only those patients classified as limited stage (using the preoperative examinations mentioned above) and diagnosed with SCLC (as confirmed by the final pathology results after the surgical operation) met the inclusion criteria for, and were thus enrolled in, this study. Conversely, patients were excluded from this study if they had a previous cancer that had been diagnosed within 5 years before the present surgical operation, had metastatic and multiple lesions (as confirmed by the final pathology results), were receiving neoadjuvant therapy or were not receiving ACT, or were suffering from palliative surgery or perioperative death (see Figure 1).
In accordance with the inclusion and exclusion criteria outlined above, the data of patients diagnosed as LS-SCLC who had undergone complete resection at Shanghai Chest Hospital from January 2018 to December 2019 were retrospectively collected. These patients were then divided into the following two subgroups: (I) the pure SCLC (p-SCLC) group; and (II) the combined SCLC (c-SCLC) group according to the final pathology results following the surgical resection.
Surgical resection and adjuvant chemotherapy
All the enrolled patients underwent surgery within 1 month of the completion of the preoperative examination. Both traditional open thoracotomy and minimally invasive surgery were used as surgical treatments. In relation to the surgical extension, either a sub-lobar resection (including a wedge resection or segmentectomy) or a lobectomy or another procedure (including a sleeve resection or pneumonectomy) were capable if a complete resection was conducted. Additionally, systemic lymph node dissection or sampling was performed to determine the correct lymph node status of the resected LS-SCLCs.
In the first course of ACT, etoposide plus carboplatin or cisplatin was administered to the LS-SCLC patients who had undergone resection within 1 to 2 months of their surgery. Next, the first-line ACT was repeated for 4 courses. Additional therapy, including postoperative radiotherapy or immune therapy, was performed as necessary. A second-line treatment was administered if cancer recurrence or distant metastasis occurred.
Next-generation sequencing examinations
Tissue deoxyribonucleic acid (DNA) was extracted from the resected SCLC specimens, and a NGS library was established. The relevant DNA fragmentations (200–400 bp in length) were prepared, and then underwent hybridization, hybrid selection, and PCR amplification. Next, all the qualified genetic profiles were subjected to capture-based sequencing with pair-end reads through the MiSeq instrument (Illumina, Inc., CA, USA). The llumina TruSeq Amplicon Cancer Panel kit (Burning Rock Biotech Ltd., China) was used to target the 68 gene mutations. Details of the 68-gene panel are provided in Table S1. After the capture-based NGS (mentioned above), the DNA rearrangement analysis was performed.
Study endpoints and follow-up strategies
The primary endpoint in this analysis was progression-free survival (PFS), which was defined as the period between the date of the surgery to the date of cancer recurrence or patient death, or the last follow-up appointment. The secondary endpoint in this analysis was overall survival (OS), which was defined as the period between the initial date of the surgery to the time of patient death or the last follow-up appointment.
In relation to the follow-up strategies, routine examinations at the outpatient department, including chest CT scans, cervical and abdominal ultrasound imaging, and serum tumor marker examinations, were recommended for each patient every 3 months in the first year of the surgery. Additional head MRI and bone scanning examinations were conducted for each patient every 6 months in the first year after the surgery. Next, the follow-up strategies were repeated each year. A PET/CT examination was conducted if needed. Telephone calls were conducted with patients who did not attend the routine follow-up visits at our outpatient department.
Statistical analysis
The categorical variables were analyzed using the Pearson χ2 or Fisher exact test, and the continuous variables were analyzed using the independent-samples t test. Kaplan-Meier curves were plotted to estimate the PFS and OS, and tested using the log-rank test. Correlation heatmaps were produced to evaluate the correlation between genetic mutations and clinicopathological characteristics. Univariable and multivariable Cox proportional hazard regression analyses were performed to investigate the independent prognostic factors for the LS-SCLC patients who underwent resection followed by postoperative chemotherapy. A subgroup analysis was also conducted according to the mutation and the wild-type RB1 gene status.
A two-sided P<0.05 was set for the statistical difference. The SPSS 22.0 (IBM Corp., Armonk, NY), R 4.0.4 (The R Foundation for Statistical Computing, Vienna, Austria), and GraphPad Prism 9 (GraphPad Software, La Jolla, CA) were performed for the related statistical analyses and figure plotting.
Results
The baseline characteristics of the primary cohort
Sixty-four LS-SCLC patients underwent surgical resection at the Shanghai Chest Hospital from January 2018 to December 2019. Patients were excluded from this study if a previous history of cancer was confirmed (n=1), or if a metastatic lesion (n=1) or multiple lesions (n=2) were confirmed by the final pathology results, or if they underwent palliative resection (n=2) or induction therapy (n=2), or did not receive ACT (n=1). A patient who died within 30 days of surgery was also excluded (n=1). Thus, in total, 51 LS-SCLC patients who underwent complete resection followed by ACT were enrolled in this study (see Figure 1).
The baseline characteristics of the LS-SCLC patients who underwent complete resections followed by ACT are set out in Table 1. Notably, there was no statistical difference between the clinical and pathological characteristics of the p-SCLC and c-SCLC groups.
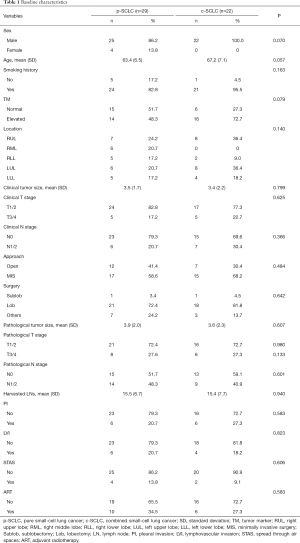
Full table
The correlation between genetic mutations and clinicopathological characteristics
In relation to the NGS genetic detection, a total of 42 genetic mutations were detected by our 68-gene panel. The TP53 (39/51, 76.5%) and RB1 (29/51, 56.9%) mutations were the 2 most common genetic mutations followed by FGFR2 (5/51, 9.8%), NOTCH1 (5/51, 9.8%), PTEN (4/51, 7.8%), PIK3CA (4/51, 7.8%), TSC2 (3/51, 5.9%), FGFR1 (3/51, 5.9%), EGFR (3/51, 5.9%), and ERBB4 (3/51, 5.9%). These mentioned mutations comprised the top 10 genetic mutations among our primary cohort (see Figure 2A). In relation to the pathological subtypes of LS-SCLC, we found that both p-SCLC and c-SCLC contained similar frequencies of genetic mutations, such as TP53, RB1, FGFR2, TSC2, NOTCH1, FGFR1, EGFR, and ERBB4, but demonstrated differences on the mutation specificity. In the p-SCLC group, there were genetic mutations, such as ROS1, BRCA1, PTCH1, ARAF, ERBB3, KRAS, and AXL, that were not detected among the c-SCLC group. However, the genetic mutations of thePI3K/AKT/mTOR singling pathway, such as PIK3CA (4/22, 18.2%), AKT1, mTOR, PTEN (4/22, 18.2%), and TSC1, and the genetic mutations of the WNT/β-catenin signaling pathway, such as APC, and MYC, along with other mutations, such as BRCA2, TOP2A, CCND1, NRAS, CDKN2A, NTRK3, BRAF, and STK11, and receptors, such as AR, ERBB2, FGFR3, and IGF1R, were detected among the c-SCLC group, which showed a unique genetic map when compared to that of the p-SCLC group (see Figure 2A).
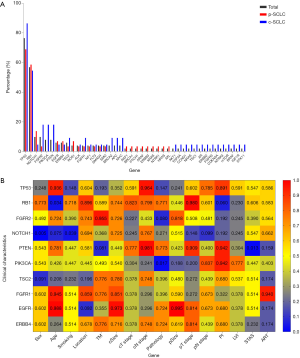
Further, we selected the top 10 mutations and analyzed the potential correlations of these most frequent genetic mutations with the clinicopathological characteristics. The RB1 mutation occurred among LS-SCLC patients of a statistically younger age (wild-type vs. mutation: 67.4±6.8 vs. 63.2±6.6; P=0.034). The NOTCH1 mutation was statistically less common in male patients than female patients (P=0.005) and those with a lower smoking burden (P=0.039). The PTEN mutation was relatively more frequent spread through air spaces (STAS) (P=0.005). The PIK3CA mutation strongly indicated the appearance of p-SCLC (P=0.017). However, TP53, and FGFR2, TSC2, FGFR1, EGFR, and ERBB4, did not show a statistical correlation with the clinical and pathological variables of resected LS-SCLC (see Figure 2B).
Oncological analysis
The median follow-up interval was 15.3 months. During this period, 17 patients suffered cancer recurrence (17/51, 33.3%), and 9 patients died (9/51, 17.6%) (7 of these patients died from cancer-related deaths and 2 from death related to other causes). The 2-year PFS rate and OS rate for those LS-SCLC patients who underwent resections followed by ACT were 48.9% and 72.3%, respectively (see Figure S1A,B). The 2-year PFS rates of p-SCLC and c-SCLC were 52.4% and 44.0%, respectively (P=0.145) (see Figure 3A). In addition, the 2-year OS rates of p-SCLC and c-SCLC were 89.6% and 51.9%, respectively (P=0.099; see Figure 3B).
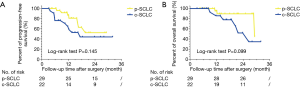
To identify the potential risk factors related to the clinical prognosis of resected LS-SCLC, univariable and multivariable Cox hazard regression analyses were performed. In the univariable Cox analysis, we found that a larger clinical tumor size (P=0.021) and a larger pathological tumor size (P=0.034), pathological lymph node (N) 1/2 stage (P=0.011), lymphovascular invasion (LVI) (P=0.005) and the wild-type RB1 (P=0.028) were potential prognostic factors related to poor PFS. Conversely, the results of the univariable Cox analysis of the clinical survival outcomes showed that an older age (P=0.028) and LVI (P=0.005) were potentially correlated with a worse OS rate (see Table 2).
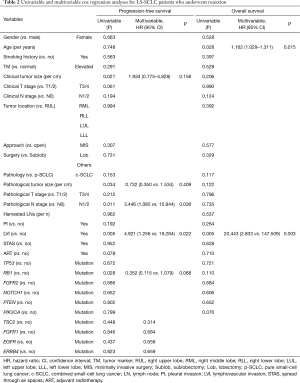
Full table
When putting the univariable Cox results into the multivariable analysis, we identified the independent risk factors using the cancer recurrence and survival outcomes of our primary candidates. Under the multivariable model of PFS, pathological N stage (Hazard ratio (HR)=3.446, 95% CI =1.085–10.944; P=0.036) and LVI (HR =4.921, 95% CI =1.256–19.284; P=0.022) were independently related to a poor PFS rate. Conversely, under the multivariable model of OS, an older age (HR =1.162, 95% CI =1.029–1.311, P=0.015) and LVI (HR =20.443, 95% CI =2.833–147.509; P=0.003) were independently correlated with worse clinical survival outcomes (see Table 2).
Subgroup analysis
The results of the multivariable Cox analysis did not show any independent relationship between the RB1 mutation and the LS-SCLC prognostic outcomes; however, we still plotted the PFS and OS curves using a Kaplan-Meier analysis, which showed that the RB1 the mutation had statistically better PFS and OS than those of the wild-type RB1 LS-SCLC mutation (see Figure S2A,B).
We then conducted a subgroup analysis according to the status of RB1 gene. In the wild-type RB1 subgroup, p-SCLC demonstrated a comparable 2-year PFS rate (P=0.856) and 2-year OS rate (P=0.091) when compared to c-SCLC (see Figure 4A,B). Conversely, in the RB1 mutation subgroup, p-SCLC had a statistically better PFS rate that that of c-SCLC (P=0.040). Notably, no p-SCLC or c-SCLC patients died during our follow-up period (see Figure 4C,D).
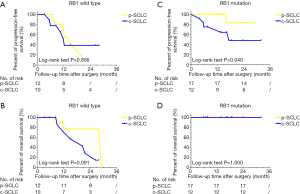
Discussion
Currently, LS-SCLC (a disease extension limited to 1 hemithorax and regional lymph nodes without extra-thoracic disease) is recommended for surgical resection under the National Comprehensive Cancer Network (NCCN) guidelines (5,11,12). However, to date, very little research has been conducted on prognostic predictions for LS-SCLC patients who undergo resection followed by postoperative ACT. In addition, the NGS with 68-gene panel was routinely performed in patients underwent surgical resections, which were targeted the most frequent genetic mutations in lung cancer. Nevertheless, no research appears to have been conducted that combines clinicopathological variables of LS-SCLC with the NGS genetic detections of 68-gene panel. According to our analysis, among the SCLC patients who underwent resection followed by ACT treatment, the proportion of c-SCLC was almost comparable to that of p-SCLC. However, our figure was obviously larger than the figure of 28% found in a previous study (4). Notably, there were no statistical differences in relation to the clinical characteristics of the p-SCLC and c-SCLC groups, which supports the findings of Guo’s and Zhang’s previous research (13,14). In relation to the survival outcomes, Zhang’s research revealed that c-SCLC patients had a worse OS rate than that of p-SCLC patients (14). However, our results showed comparable cancer recurrence and survival outcomes between those two pathological subtypes; thus, the difference of SCLC subtypes did not appear to affect the survival of LS-SCLC patients who underwent resection followed by ACT treatment.
In examining the genetic level and the use of NGS examinations, we found both similarities and differences in the genetic mutations of the p-SCLC and c-SCLC patients. TP53 and RB1 were the 2 most frequent mutations in both p-SCLC and c-SCLC patients, which reflects the results of a previous study (4). Additionally, both pathological subtypes shared some other similar mutations; for example, FGFR2, NOTCH1, TSC2, EGFR, and ERBB4 were highly expressed in the total SCLC cohort. However, the genetic mutations related to the PI3K/AKT/mTOR signaling pathway, such as PIK3CA, AKT1, mTOR, PTEN, and TSC1, and genetic mutations related to the WNT/β-catenin signaling pathway, such as APC and MYC, and some other genetic mutations showed the peculiarity of c-SCLC in contrast to p-SCLC, and strongly indicated differences in tumor origin and carcinogenesis among the two subtypes. Further, in the correlation analysis of the clinicopathological variables and genetic mutations, we also found that PIK3CA was strongly related to the c-SCLC subtype, and PTEN was closely related with STAS appearance in c-SCLC, which shows the particularity of c-SCLC compared to p-SCLC. Thus, while both p-SCLC and c-SCLC represent two different diseases in LS-SCLC patients, no statistical difference was found in the clinicopathological and survival outcomes of patients. Other variables should be considered in future studies with larger sample sizes. In relation to the survival outcomes for the genetic mutations, previous studies have shown that LS-SCLC and extensive-stage SCLC patients with the RB1 mutation had better survival outcomes compared with wide-type RB1 (15,16). Accordingly, we analyzed the prognostic effect of the RB1 mutation in patients who underwent resection followed by ACT, and found that patients with the RB1 mutation had much better PFS and OS than patients with the wild-type RB1. However, the results of the univariable and multivariable Cox analyses showed that the RB1 mutation was not an independent prognostic predictor of either cancer recurrence or clinical survival outcomes. Apart from the RB1 mutation, the other 10 top genetic mutations had no statistical effect on the clinical survival outcomes of the LS-SCLC patients who underwent resection followed by ACT; rather, it was the traditional clinicopathological characteristics, such as age, pathological N stage, and LVI, that were strongly correlated with LS-SCLC patients’ clinical outcomes. Notably, when analyzing the prognostic effect of the RB1 mutation in 2 pathological subtypes, we found that p-SCLC patients with the RB1 mutation had critically better PFS compared to patients with c-SCLC. This might be due to the genetic peculiarity of c-SCLC whereby the PI3K/AKT/mTOR and WNT/β-catenin and some other signaling pathways strongly contribute to cancer cell growth, differentiation, proliferation, and therapeutic resistance (17,18). Thus, targeting the PI3K/AKT/mTOR or WNT/β-catenin signaling pathways could represent a potential therapeutic strategy for c-SCLC patients (19,20).
Apart from pathological classification, we also found that surgical operations did not affect survival outcomes; however, these results counter those of Raman who found that LS-SCLC patients who underwent a wedge resection had worse survival outcomes than those who underwent a lobectomy (21). This difference may have arisen because the patients in our study all received postoperative chemotherapy, but those in Raman’s did not. In relation to lymph node dissection, a previous study found that there was no difference in survival outcomes when the number of dissected lymph nodes for LS-SCLC increased (22). Our research also indicated that the number of lymph nodes dissected or sampled during the LS-SCLC operation did not affect cancer recurrence or survival outcomes.
Previous research has shown that concurrent chemoradiation resulted in better survival outcomes than sequential chemoradiation (23). Concurrent chemoradiation plus pembrolizumab is also a promising strategy for treating LS-SCLC (24). However, the question arises as to whether postoperative radiotherapy is necessary to the comprehensive therapy of LS-SCLC patients who undergo resection followed by ACT. Zhou indicated that the clinical survival outcomes of LS-SCLC patients were not affected by mediastinal postoperative radiotherapy (PORT) or prophylactic cranial irradiation (PCI)treatment (25). In our research, we also found that LS-SCLC patients who underwent resection followed by ACT did not benefit from adjuvant radiotherapy. It is still unknown whether adjuvant radiotherapy is necessary. However, it is our view that an initial surgical operation followed by complete ACT are both essential steps in the multimodality treatment of patients with operable LS-SCLC.
This study had several limitations. First, the sample size was relatively small, as only a limited number of LS-SCLC patients received surgical resection and postoperative ACT treatment at the hospital during the study period. The low sample size of eligible patients might have produced statistical biases in our research analysis. Second, due to the small sample size and short follow-up period, the genetic mutations detected by NGS might not be representative or universal. Thus, future studies need to be conducted with larger sample sizes. Third, in recent years, a new kind of SCLC classification has been proposed according to the expression of 4 specific genes (26,27). In near future, we intend to add those four genes to our previous gene panel to enhance our NGS.
In conclusion, c-SCLC had similar clinical and pathological characteristics to p-SCLC. TP53 and RB1 were the two major genetic mutations present in both p-SCLC and c-SCLC. c-SCLC had unique genetic profiles related to the PI3K/AKT/mTOR and WNT/β-catenin signaling pathways. No prognostic difference was found between c-SCLC and p-SCLC. However, the pathological N stage of LVI was correlated with PFS and age, and LVI was corelated with OS. Neither the pathological subtypes nor genetic mutations affected the survival outcomes. Notably, of the LS-SCLC patients who underwent resection followed by ACT, RB1 mutated c-SCLC patients had poorer DFS than p-SCLC patients.
Acknowledgments
Funding: This research was supported by the Basic Cultivation Project of Shanghai Chest Hospital (2018YNJCM01).
Footnote
Reporting Checklist: The authors have completed the STROBE reporting checklist. Available at https://dx.doi.org/10.21037/atm-21-3353
Data Sharing Statement: Available at https://dx.doi.org/10.21037/atm-21-3353
Conflicts of Interest: All authors have completed the ICMJE uniform disclosure form (available at https://dx.doi.org/10.21037/atm-21-3353). The authors have no conflicts of interest to declare.
Ethical Statement: The authors are accountable for all aspects of the work in ensuring that questions related to the accuracy or integrity of any part of the work are appropriately investigated and resolved. This study was approved by the Institutional Ethics Committee of Shanghai Chest Hospital (KS1992), and the research process was conducted in accordance with the Helsinki Declaration (as revised in 2013). Written informed consent was not needed because of the retrospective nature of the research.
Open Access Statement: This is an Open Access article distributed in accordance with the Creative Commons Attribution-NonCommercial-NoDerivs 4.0 International License (CC BY-NC-ND 4.0), which permits the non-commercial replication and distribution of the article with the strict proviso that no changes or edits are made and the original work is properly cited (including links to both the formal publication through the relevant DOI and the license). See: https://creativecommons.org/licenses/by-nc-nd/4.0/.
References
- Chen W, Zheng R, Baade PD, et al. Cancer statistics in China, 2015. CA Cancer J Clin 2016;66:115-32. [Crossref] [PubMed]
- Wei S, Wei B, Tian J, et al. Comparison of treatment strategies for patients with limited-stage small cell lung cancer who received chemotherapy. Transl Cancer Res 2020;9:818-26. [Crossref]
- Rudin CM, Ismaila N, Hann CL, et al. Treatment of small-cell lung cancer: American society of clinical oncology endorsement of the American college of chest physicians guideline. J Clin Oncol 2015;33:4106-11. [Crossref] [PubMed]
- Raso MG, Bota-Rabassedas N, Wistuba II. Pathology and Classification of SCLC. Cancers (Basel) 2021;13:820. [Crossref] [PubMed]
- Nesbit EG, Leal TA, Kruser TJ. What is the role of radiotherapy for extensive-stage small cell lung cancer in the immunotherapy era? Transl Lung Cancer Res 2019;8:S153-62. [Crossref] [PubMed]
- Kalemkerian GP, Akerley W, Bogner P, et al. Small cell lung cancer. J Natl Compr Canc Netw 2013;11:78-98. [Crossref] [PubMed]
- Aisner J, Goutsou M, Maurer LH, et al. Intensive combination chemotherapy, concurrent chest irradiation, and warfarin for the treatment of limited-disease small- cell lung cancer: a Cancer and Leukemia Group B pilot study. J Clin Oncol 1992;10:1230-6. [Crossref] [PubMed]
- Pietanza MC, Byers LA, Minna JD, et al. Small cell lung cancer: will recent progress lead to improved outcomes? Clin Cancer Res 2015;21:2244-55. [Crossref] [PubMed]
- Combs SE, Hancock JG, Boffa DJ, et al. Bolstering the case for lobectomy in stages I, II, and IIIA small-cell lung cancer using the National Cancer Data Base. J Thorac Oncol 2015;10:316-23. [Crossref] [PubMed]
- Lanuti M. Commentary: Wedge resection for limited-stage small cell lung cancer: Not an acceptable modality for curative intent. J Thorac Cardiovasc Surg 2021;161:1493-4. [Crossref] [PubMed]
- Daly ME, Ismaila N, Decker RH, et al. Radiation Therapy for Small-Cell Lung Cancer: ASCO Guideline Endorsement of an ASTRO Guideline. J Clin Oncol 2021;39:931-9. [Crossref] [PubMed]
- Kalemkerian GP, Loo BW, Akerley W, et al. NCCN Guidelines Insights: Small Cell Lung Cancer, Version 2.2018. J Natl Compr Canc Netw 2018;16:1171-82. [Crossref] [PubMed]
- Guo Y, Yang L, Liu L, et al. Comparative study of clinicopathological characteristics and prognosis between combined and pure small cell lung cancer (SCLC) after surgical resection. Thorac Cancer 2020;11:2782-92. [Crossref] [PubMed]
- Zhang J, Zhang L, Luo J, et al. Comprehensive genomic profiling of combined small cell lung cancer. Transl Lung Cancer Res 2021;10:636-650. [Crossref] [PubMed]
- Udagawa H, Umemura S, Murakami I, et al. Genetic profiling-based prognostic prediction of patients with advanced small-cell lung cancer in large scale analysis. Lung Cancer 2018;126:182-8. [Crossref] [PubMed]
- Dowlati A, Lipka MB, McColl K, et al. Clinical correlation of extensive-stage small-cell lung cancer genomics. Ann Oncol 2016;27:642-7. [Crossref] [PubMed]
- Makinoshima H, Umemura S, Suzuki A, et al. Metabolic Determinants of Sensitivity to Phosphatidylinositol 3-Kinase Pathway Inhibitor in Small-Cell Lung Carcinoma. Cancer Res 2018;78:2179-90. [Crossref] [PubMed]
- Wagner AH, Devarakonda S, Skidmore ZL, et al. Recurrent WNT pathway alterations are frequent in relapsed small cell lung cancer. Nat Commun 2018;9:3787. [Crossref] [PubMed]
- Umemura S, Mimaki S, Makinoshima H, et al. Therapeutic priority of the PI3K/AKT/mTOR pathway in small cell lung cancers as revealed by a comprehensive genomic analysis. J Thorac Oncol 2014;9:1324-31. [Crossref] [PubMed]
- Paripati A, Kingsley C, Weiss GJ. Pathway targets to explore in the treatment of small cell and large cell lung cancers. J Thorac Oncol 2009;4:1313-21. [Crossref] [PubMed]
- Raman V, Jawitz OK, Yang CJ, et al. The effect of extent of resection on outcomes in patients with limited stage small cell lung cancer. J Thorac Cardiovasc Surg 2021;161:1484-1492.e5. [Crossref] [PubMed]
- Rucker AJ, Raman V, Jawitz OK, et al. Effect of Lymph Node Assessment on Outcomes in Surgery for Limited Stage Small Cell Lung Cancer. Ann Thorac Surg 2020;110:1854-60. [Crossref] [PubMed]
- Takada M, Fukuoka M, Kawahara M, et al. Phase III Study of Concurrent Versus Sequential Thoracic Radiotherapy in Combination With Cisplatin and Etoposide for Limited-Stage Small-Cell Lung Cancer: Results of the Japan Clinical Oncology Group Study 9104. J Clin Oncol 2002;20:3054-60. [Crossref] [PubMed]
- Welsh JW, Heymach JV, Guo C, et al. Phase I/II Trial of Pembrolizumab and Concurrent Chemoradiation Therapy for Limited-Stage Small Cell Lung Cancer. J Thorac Oncol 2020;15:1919-27. [Crossref] [PubMed]
- Zhou N, Bott M, Park BJ, et al. Predictors of survival following surgical resection of limited-stage small cell lung cancer. J Thorac Cardiovasc Surg 2021;161:760-771.e2. [Crossref] [PubMed]
- Rudin CM, Poirier JT, Byers LA, et al. Molecular subtypes of small cell lung cancer: A synthesis of human and mouse model data. Nat Rev Cancer 2019;19:289-97. [Crossref] [PubMed]
- Baine MK, Hsieh MS, Lai WV, et al. SCLC Subtypes Defined by ASCL1, NEUROD1, POU2F3, and YAP1: A Comprehensive Immunohistochemical and Histopathologic Characterization. J Thorac Oncol 2020;15:1823-35. [Crossref] [PubMed]
(English Language Editor: L. Huleatt)