Effects of N-Methyl-D-aspartate receptor (NMDAR) and Ca2+/calmodulin-dependent protein kinase IIα (CaMKIIα) on learning and memory impairment in depressed rats with different charge by modified electroconvulsive shock
Introduction
Depression is a highly prevalent psychological disease. According to the 2017 report of the World Health Organization (WHO), there are approximately 322 million people worldwide with depression, and its prevalence is 4.4% (1). The main manifestations of depression are increased negativity (such as amplified pessimism) and decreased positivity (such as a lack of sense of pleasure), which seriously affect the quality of life. Major depressive disorder (MDD) and treatment-resistant depression (TRD) have the tendency of self-mutilation and even suicide. Modified electroshock therapy (MECT) has a high effective rate and remission rate in a variety of treatment options for depression, especially for MDD. However, due to its damage to cognitive function, the clinical application of MECT is limited, especially for patients who required increased charge, such as the elderly, women, and patients who cannot achieve therapeutic effects with a low current (2-4). This memory impairment is mainly manifested as post-treatment confusion, anterograde amnesia, and retrograde amnesia. Our previous studies have found that high-energy electroconvulsive shock (MECS, an animal model of MECT) can affect synaptic plasticity and the long-term potentiation (LTP) induction threshold of depressed rats after MECS is significantly increased, which may be one of the mechanisms (5-7). However, the internal connection between MECS charge and learning ability impairment needs further study.
Synaptic plasticity refers to the ability of synapses to undergo adaptive changes in their morphological structure and transmission efficiency under the influence of various factors. The LTP in hippocampal CA1 region is a typical representative of excitatory synapses (8). It has been shown that MECS can cause “LTP-like synaptic efficacy changes” in the hippocampus and increase the potentiation degree of LTP in stronger high frequency stimulation (9,10). Morphologically, MECS induces the growth of nerve fibers and the increase of dendritic spines and synapses. From the perspective of synaptic function, it is shown as the enhancement of neurosynaptic transmission efficiency. It is considered one of the important mechanisms of the antidepressant effect of MECS, as well as the embodiment of its therapeutic efficacy (11). However, MECS can increase the LTP induction threshold, and thus it is necessary to increase the charge to reach the suprathreshold stimulation to maintain LTP, which is considered “LTP inhibition”, resulting in learning and memory damage (12). This change of the induced threshold is called “metaplasticity”, that is, the plasticity of synaptic plasticity, which is an important mechanism of synaptic homeostasis. Based on the relationship between the charge of MECS and the impairment of cognitive function, we hypothesized that the MECS stimulation intensity is different and that the threshold increase amplitude is also different: with the increase of MECS charge, the LTP induction threshold would further increase, and subsequently LTP would be more difficult to induce, that is, there was a “MECS charge-dependent LTP induction threshold regulation window”. It has not yet been determined how MECS current changes the threshold window. Plata et al. showed that Ca2+ channel and intracellular Ca2+ concentration play an important role in LTP (13). N-Methyl-D-aspartate receptor (NMDAR) is a ligand-gated and voltage-gated ion channel receptor. After activation, NMDAR can mediate the influx of Ca2+ and Na+, and can mediate neuronal excitatory transmission and synaptic plasticity. In the hippocampus, NMDAR is mainly composed of phospho-NMDA receptor 1 (GluN1) and GluN2, while GluN2A and GluN2B are the main regulatory subunits. Relevant research has shown that change in the ratio of GluN2A/GluN2B affects the LTP threshold and is called “NMDAR developmental switch” (14,15). The change of NMDAR structure affects the binding of Ca2+/calmodulin-dependent protein kinase IIα (CaMKIIα) and the formation of GluN2B/CaMKIIα complex can affect the induction and maintenance of LTP (16).
The purpose of this study was to investigate whether differences in MECS charge affects the expression and interaction of CaMKIIα and NMDAR, changes synaptic plasticity, and thereby affects learning and memory. We present the following article in accordance with the ARRIVE reporting checklist (available at https://dx.doi.org/10.21037/atm-21-3690).
Methods
Animals
Healthy male Sprague Dawley (SD) rats (2–3 months, 180–250 g), were purchased from the Animal Experimental Center of Chongqing Medical University and raised in a specific pathogen free (SPF) room (20–24 °C, 12 h/12 h light-dark alternate). All rats were reared adaptively for 7 d before experiments (Figure 1). Then, they were raised alone and established with the depression model. All experimental protocols were approved by the Ethics Committee of the First Affiliated Hospital of Chongqing Medical University and conducted according to National Institutes of Health Guide for the Care and Use of Laboratory Animals. A protocol was prepared before the study without registration.

Chronic unpredictable mild stresses (CUMS) procedure
According to the methods used in a previous study, the rat model of depression was established by CUMS procedure (17). For the next 28 d (Figure 1), modeling was started at 9 a.m. Except in group C, the following stimulation methods were randomly selected every day: cold water swim (4 °C) for 5 min, hot water swim (45 °C) for 5 min, continuous illumination for 24 h, damp sawdust for 24 h, water deprivation for 24 h, food deprivation for 24 h, tilt cage (45°) for 24 h, strobe for 4 h, forceps clipped on tail for 1 min, or shaking of the cage for 20 min. The same stimulus was not used on the same rat on any 2 consecutive stimulations.
Body weight
All rats were weighed at 9 a.m. on days 0, 7, 14, 21, and 28 of CUMS. The rats were placed in an empty cage and weighed with electrons.
Sucrose preference test (SPT)
According to a previous report with minor modifications (18), SPT was used to detect anhedonia performance (core symptoms of depression) in rats. Adaptive water drinking was conducted for 48 h (Figure 1): two bottles of 1% sucrose solution were provided in the first 24 h, and one bottle of sterile water and one bottle of 1% sucrose solution were provided in the other 24 h. The SPT process was as follows: two bottles of water, one bottle of 1% sucrose water and one bottle of sterile water are allowed to be consumed freely after fasting and water deprivation for 24 h. The water drinking period was 2 h, and the water bottle’s position was changed halfway through the test time. The weight of the 2 water bottles was recorded before and after drinking. The formula of sucrose preference percentage (SPP) was as follows: SPP (%) = sucrose water consumption (g) × [sucrose water consumption (g) + sterile water consumption (g)] × 100%. The SPT was performed 3 times, before CUMS, after CUMS, and after MECS, respectively. According to the report (19,20), SPP ≤65% was defined as the depression like behavior of rats with anhedonia.
Experimental animals and treatments
After SPP screening, rats were randomly allocated to 6 groups (n=12): group C was a healthy control group without any treatment; the remaining 5 groups were all depression models established by CUMS, and were randomly assigned into the group after screening by SPT. The depressed animals were divided into the following groups and treatments: the M0, M60, M120, M180, and M240 groups were treated with MECS at 0, 60, 120, 180, and 240 mC stimulation intensity, respectively, after propofol (GV461, AstraZeneca, Italy) pretreatment (80 mg/kg, i.p.), once a day for 7 consecutive days. The parameters of MECS were set as follows: bidirectional square wave pulses, 0.8 A in amplitude, 1.5 ms in width, 125 Hz in frequency, duration of 0.4, 0.8, 1.2, and 1.6 s (for 60, 120, 180, 240 mC).
Morris water maze (MWM)
MWM is often used to evaluate the spatial learning and memory function of rodents. The protocol was conducted according to Zhang et al. (21). Starting time was 9 a.m. each day to avoid changes in learning and memory caused by circadian rhythms. A round pool (150 cm diameter, 100 cm height) was filled with water (50 cm deep, 21–23 °C) and dyed black with ink and divided into 4 quadrants: SE, SW, NE, NW. An opaque circular platform (11 cm diameter, 2 cm below water surface) was placed in the middle of NW quadrant. Rats randomly entered the water from one of the 4 quadrants to find the hidden platform within 60 s, and each quadrant involved an experiment. If the rat did not board the platform within 60 sec, it was guided to the platform and remained there for 15 sec. The above operation lasted for 5 days. On the 6th day, the platform was removed and rats entered the water from the same quadrant to swim for 60 sec. A video tracking system (ZH0065, Zhenghua Instruments, Anhui, China) was installed directly above the water tank, setting a fixed swimming time of 60 sec, recording the swimming speed, path, landing on the target platform, and swimming distance in each quadrant. The escape latency (EL, average time to find the platform of 4 quadrants) of the first 5 d and the space exploration time (SET, the swimming time from the quadrant of the hidden platform) of the 6th d were recorded.
Open field test (OFT)
The OFT was used to detect the exploratory behavior of rats in a new environment and evaluate the degree of depression. As described in the report and modified slightly (22), a black square box (100×100×50 cm3) was used. The bottom was equally divided into 25 square cells (20×20 cm2), and dimly illuminated. The rats were placed in the center and their activity tracks were recorded. The amount of grid crossing and incidence of rearing in 3 min were recorded by video tracking software (ZH-ZFT, Zhenghua Instruments, Anhui, China).
Western blot assay
The rats were sacrificed the day after the behavioral experiment, the bilateral hippocampus was promptly removed, placed onto ice, and stored in the refrigerator at −80 °C. The hippocampus was homogenized after adding radioimmunoprecipitation assay (RIPA) lysate and phenylmethylsulfonyl fluoride (PMSF) enzyme inhibitor, and centrifuged at 12,000 rpm for 15 min at 4 °C to extract the supernatant. The BCA kit was used to measure the protein concentration. We prepared the appropriate concentration of SDS-PAGE according to molecular weight to separate proteins, which were then transferred onto a PVDF membrane. The cells were then blocked with 5% skim milk at 37 °C for 2 h, and incubated with antibody overnight at 4 °C: GluN1 (1:1,000, ab17345, Abcam, Cambridge, MA, USA), GluN2A (1:1,000, ab14596, Abcam), GluN2B (1:1,000, ab65783, Abcam), CaMKIIα (1:1,000, 11945S, Cell Signaling Technology, Danvers, MA, USA), p-T305-CaMKII (1:1,000, AF3492, Affinity, Cincinnati, OH, USA), postsynaptic densities-95 (PSD-95) (1:500, ab18258, Abcam) and glyceraldehyde 3-phosphate dehydrogenase (GAPDH, 1:5,000, AF7021, Affinity). Then, membranes were incubated with horseradish peroxidase (HRP)-conjoined immunoglobulin G (Ig-G, 1:5,000, BA1054, Boster, Pleasanton, CA, USA) for 1 h. Blots were visualized by chemiluminescence substrate (AR1171, Boster) and quantified with Bio-Rad imaging system (Bio-Rad, Hercules, CA, USA).
Co-immunoprecipitation (CO-IP) and immunoblotting (IB)
CO-IP was used to investigate the interaction between CaMKIIα and GluN2B subunits in the hippocampus after MECS. The protein extraction was prepared as described above (23). Briefly, the sample was precipitated by anti-CaMKIIα or anti-GluN2B antibody, then the protein was evaluated by western blots using the above 2 antibodies. An IB assay for CaMKIIα or GluN2B was used as control.
Statistical analysis
The software SPSS version 17.0 (SPSS Inc., IBM Corp., Armonk, NY, USA) was used to analyze the collected data. The mean values between the 2 groups were compared by analysis of variance (ANOVA) of 2 independent samples. The behavioral data of repeated measurements were analyzed by variance analysis of repeated measurements to determine the statistical significance. Differences in means among multiple groups were analyzed by 1-way ANOVA or 2-way ANOVA, followed by Bonferroni correction. Statistical significance was indicated when P<0.05.
Results
Body weight
On days 0, 7, 14, and 21 of CUMS (group C, n=6, modeling groups, n=12 each group), there was no significant difference in the average body weight between the CUMS groups and the control group; the difference in body weight between the 2 groups was statistically significant on day 28 (t=7.938, P<0.001, Figure 2A). Compared with the control group, the CUMS group weighed less.
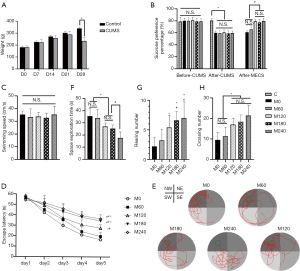
SPT
There was no significant difference in SPP before CUMS, but there was a statistical difference after CUMS. Compared with group C, the SPP of M0, M60, M120, M180, and M240 groups decreased significantly (F =24.02, P<0.001, Figure 2B), but there was no significant difference among the latter 5 groups. After MECS, compared with group M0 and M60, the SPP of the M120, M180, and M240 groups increased significantly (F =19.80, P<0.001), but there was no significant difference between the first 2 groups and the last 3 groups.
MWM
There was no significant difference in swimming speed among the groups (n=6, Figure 2C). With the extension of learning time, the EL of 5 consecutive days gradually shortened. Moreover, the EL was prolonged with the increase of charge, but there was no significant difference between M0 and M60, or between the M180 and M240 groups (F =313.07, P<0.05, Figure 2D). After removing the platform on the 6th day, it was observed that the SET shortened with the increase of charge (F =10.09, P<0.05, Figure 2E,2F) and there was no significant difference between M0 and M60, or between M120 and M180.
OFT
After MECS treatment, the differences in the amount of grid crossing and the incidence of rearing were statistically significant. For the incidence of rearing, M120, M180, and M240 increased compared with group M0, and M180 and M240 increased compared with M60 (F =5.54, P<0.05, Figure 2G). There was no significant difference between the other groups. Compared with group M0 and M60, the amount of grid crossing in the M120, M180, and M240 groups increased significantly (F =7.66, P<0.05, Figure 2H).
Western blot assay
The expression of CaMKIIα and p-T305-CaMKII
No significant alterations in the expression levels of CaMKIIα were detected (Figure 3A,3B); but for p-T305-CaMKII, compared with group M0 and M240, the expression was lower in M60, M120, and M180, and compared with group M180, the expression was lower in M60 and M120 (F =58.02, P<0.05, Figure 3A,3C). There was no significant difference between the other groups.
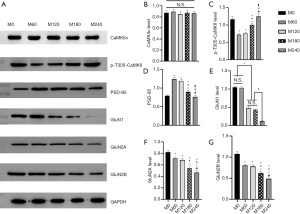
The expression of PSD-95
The expression of PSD-95 was significantly different between the groups (F =100.46, P<0.05, Figure 3A,3D). Compared with group M0 and M240, PSD-95 expression was higher in M60, M120, and M180, with that in M180 being lower than M60 and M120. There was no significant difference between the other groups.
The expression of GluN1, GluN2A, and GluN2B
There were statistical differences in GluN1 protein expression between groups (F =437.55, P<0.001, Figure 3A,3E). Compared with group M240, the expression of GluN1 was higher in the other 4 groups, with M120 and M180 having lower expression than M0 and M60. There were also significant differences in GluN2A among the groups (F =128.01, P<0.05, Figure 3A,3F). The expression level in the M0 group was significantly higher than that in the other 4 groups. With the increase of power, the expression level gradually decreased, but there was no statistically significant difference between the M60 and M120 groups. The expression of GluN2B was similar to that of GluN2A (F =99.57, P<0.001, Figure 3A,3G). The ratio of GluN2A/GluN2B in M240 was higher than M0 (F =2.30, P<0.05, Figure 4A), but the other groups showed no significant difference.
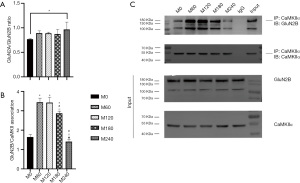
Co-expression of CaMKIIα with GluN2B subunits
We performed CO-IP to determine whether the binding amount of GluN2B and CaMKIIα was changed by different electric charges. The results showed that CaMKIIα binding to GluN2B was highest in the M60 and M120 groups, followed by M180, and the least binding amount was detected in M0 and M240 (F =100.46, P<0.05, Figure 4B,4C). These results showed that depression can hinder GluN2B and CaMKIIα binding, and the same effect can be found in MECS with high electric charge.
Discussion
This study found that as the charge of MECS increased, the antidepressant effect on depressed rats was optimized at 120 mC stimulation intensity. Although N-methyl-D-aspartate receptor (NMDAR) subunit, one of the indicators related to depression, was decreased under higher current, unfortunately the depressive behavior was not improved for the better. However, with the increase of stimulation intensity, the impairment of learning function in MWM was gradually aggravated, which may have been related to the changes of NMDAR subunit composition and the increase of CaMKIIα phosphorylation, as well as the decrease of the formation of CaMKIIα/GluN2B complex.
Depression is a kind of emotional disorder characterized by loss of pleasure and interest. At present, common depression models include environmental stimulation models, drug stimulation models, transgenic models, surgical preparation models, and postpartum depression models (24). The chronic unpredictable mild stress model is an environmental stimulus model that simulates the predisposing factors and pathogenesis of human depression. It is one of the classic models of depression, and has a history of more than 30 years (25). It has good effectiveness and reliability, and a suitable stimulation mode can be achieved according to the laboratory conditions. The results of this experiment showed that 28 d after modeling, the body weights of rats were lighter than the control group in the same period, and compared with the measurements at 21 d, there was a negative weight gain. After 28 d of modeling, the SPP was significantly lower than that of the control group, indicating that the modeling was successful. Some researchers believe that the efficacy of MECS is related to the intensity of stimulation, and the antidepressant effect can only be obtained by reaching suprathreshold stimulation (26-28). Our previous study found that the convulsion threshold of MECS treatment under propofol general anesthesia was about 40 mC (29). In this study, 0, 1.5, 3, 4.5, and 6 times the threshold were used for treatment. The experiment found that the amounts of grid crossing and SPP were increased in the medium (120 mC) and high power group (≥180 mC), but did not reach the statistical difference level, while the incidence of rearing increased in the high power group. It is suggested that the level of electric charge at 120 mC has a good antidepressant effect, which is consistent with the results reported by Zhang et al. (30). The results show that the charge level at 120 mC had reached the “ceiling” of the antidepressant effect of MECS for the majority of depressed rats, which is consistent with the results reported by Zhang et al. However, in MWM, the learning and memory ability of the high-power group was poor, which indicates that the learning disorder caused by MECS is aggravated with the increase of current.
The LTP has been recognized for nearly 50 years, and it has caused wide concern because of its correlation with learning and memory (7). In the hippocampal CA1 region, LTP is a typical representative of excitatory synapses, which depends on the activation of NMDAR. Activation of NMDAR causes Ca2+ influx and activates CaMKIIα, which transfers to the postsynaptic membrane and combines with NMDAR at the postsynaptic density to form a NMDAR/CaMKIIα complex (31). A bridging role is played by PSD-95, and the changes of PSD-95 content affect the excitatory postsynaptic current (EPSC) (32-34). The complex can regulate LTP by binding with different subunits of NMDAR. According to Liang et al., loss of GluN2B may inhibit LTP induction by destroying the formation of CaMKIIα-NMDAR (35). Additionally, CaMKII, especially the α subunit, plays a key role in human Ca2+ signal transduction and LTP formation (36). It is an indispensable serine/threonine protein kinase in learning and memory formation of the hippocampus, and is highly expressed in PSD. Relevant studies have shown that its phosphorylation level has a bidirectional regulatory effect (37). When thr286 is activated by self-phosphorylation, it has a positive effect on the formation of LTP, while phosphorylation at Thr305/306 can inhibit LTP (38). Pi et al. found that CaMKII affects LTP induction threshold depending on the phosphorylation status of CaMKII-Thr305/306 site (39). Our previous study found that MECS can up regulate the expression of p-T305-CaMKII, up regulate the baseline field excitatory postsynaptic potential (fEPSP), and damage LTP, leading to cognitive impairment (4). And our previous study found (10) that MECS can up-regulate baseline fEPSP, damage LTP, and lead to cognitive impairment, and LTP amplitude can be further increased in the ECS group by increasing the stimulus intensity to induce LTP, but it has not been tested in vivo. The results of this study found that the expression level of p-T305-CaMKII increased with the increase of electric charge of MECS, which indicated that increasing the stimulation amount of MECS might lead to the increase of the LTP induced threshold, which would injure the learning and memory ability.
The NMDAR is both a ligand-controlled ion channel receptor and a voltage-controlled ion channel receptor. It plays an important role in the excitatory transmission of the central nervous system and maintains central physiological functions, such as learning, memory, and synaptic plasticity (40). Its structural changes can regulate the maintenance and induction of LTP by affecting the formation of the NMDAR/CaMKIIα complex. In addition, GluN2A and GluN2B, the major regulatory subunits in mammalian hippocampus, play different roles in function (41). Erreger et al. found that the relative expression of GluN2A and GluN2B can affect spontaneous synaptic transmission, as well as activity-dependent synaptic plasticity and its direction (42). Activation and deactivation of GluN2B happens slowly, it a high calcium ion permeability, and a high affinity with CaMKIIα, making it easier to form NMDAR/CaMKIIα complexes (43). Related studies have shown that reducing the combination of GluN2B and CaMKII will damage LTP (44,45). In this study, we found that with higher electric charge, the combined amount of the 2 decreases, and the expression levels of GluN1 and GluN2B in the hippocampus show a decreasing trend, while GluN2A/GluN2B ratio only increases significantly under the condition of high stimulation electricity. It is suggested that one of the mechanisms of impairment of learning and memory in MECS is to damage LTP by reducing the binding amount of GluN2B and CaMKIIα, and the damage may also be related to the subunit composition of NMDAR under high electric charge.
Currently, in clinical practice, there are 2 commonly used methods to determine the electroconvulsive therapy (ECT) stimulus dose, one is based on the patient’s age (5× or 2.5× age in years, in mC), the other is titration method (5–6× ST in right unilateral or 1.5–2× ST in bilateral, ST is defined as the minimum current dose required to induce a generalized seizure lasting 20–25 sec) (46-49). It can be seen that some patients require more than 120 mC for treatment, which will incur more serious cognitive impairment. In addition to looking for the lowest possible stimulation charge to avoid cognitive side effects, it is also possible to explore new compound therapy with protective effects. This has been the pursuit of many researchers in the past 80 years since the development of ECT (50-53). In this study, we found that higher current MECS caused the continuous decrease of GluN1, GluN2A, and GluN2B, and the increase of p-T306-CaMKII, which damages LTP and leads to impairment of learning and memory. Therefore, NMDAR may be a target for the protection of cognitive function in the process of MECS. It has been found in some animal and clinical studies that NMDAR inhibitors can reduce the excessive induction of LTP by ECT, thus alleviating the memory damage (54,55). A review suggested that ketamine may be a candidate drug for the prevention of retrograde amnesia caused by ECT (56). In addition, propofol is not only a GABA receptor agonist, but also an NMDAR inhibitor, and can regulate calcium influx (57-59). In our previous study, propofol was shown to reduce the down-regulation of NMDAR and the up-regulation of p-T305-CaMKII by MECS, thus improving spatial learning and memory ability (5,60). Bauer et al. found that propofol can significantly reduce the duration of seizure caused by MECT without affecting its antidepressant effect (61). Thus, we have reason to believe that propofol dose can be increased to achieve better protection of cognitive function when there is need to increase the charge of MECS to achieve antidepressant effect. This study provides new evidence for the improvement of spatial learning lesion by ECT combined with NMDAR antagonist. However, the specific medication measures require further exploration.
The limitation of this study is that the same electric quantity was used for MECS treatment for 7 consecutive days, the electricity quantity was not adjusted according to the evaluation results of depression state improvement after clinical treatment, and there was no analysis of the individual differences in curative effect, which may have led to the reasoning that there was no statistical difference between the therapeutic effect of high electric quantity and that of medium electric quantity. At the same time, this study did not observe the change of fEPSP after different charge treatments to verify the change of LTP induction threshold.
Conclusions
To sum up, this study found that with the increase of the MECS stimulation electricity, the depressive state of rats was not shown to be significantly improved at high electricity, but there was a significant decrease in the level of learning and memory. We consider that the proportion of NMDAR subunits and the decrease in the amount of binding to CaMKIIα leads to an increase in the LTP induction threshold. To optimize the selection of charge, more research should be conducted in combination with age, gender, and other factors. However, it should be noted that NMDAR antagonists may be effective combination drugs to protect cognitive function in the process of ECT.
Acknowledgments
Funding: This study received funding support from the National Natural Science Foundation of China grant (81873798 and 81901377); National Key Clinical Specialty Construction Project (2011-170); Chongqing Medical Key Discipline Construction Project (2007-2); and the Foundation of Postgraduate Innovation Project of Chongqing province, China (CYS19189), for which the authors are greatly appreciative.
Footnote
Reporting Checklist: The authors have completed the ARRIVE reporting checklist. Available at https://dx.doi.org/10.21037/atm-21-3690
Data Sharing Statement: Available at https://dx.doi.org/10.21037/atm-21-3690
Conflicts of Interest: All authors have completed the ICMJE uniform disclosure form (available at https://dx.doi.org/10.21037/atm-21-3690). The authors report that this study received funding support from the National Natural Science Foundation of China grant (81873798 and 81901377); National Key Clinical Specialty Construction Project (2011-170); Chongqing Medical Key Discipline Construction Project (2007-2); and the Foundation of Postgraduate Innovation Project of Chongqing province, China (CYS19189). The authors have no other conflicts of interest to declare.
Ethical Statement: The authors are accountable for all aspects of the work in ensuring that questions related to the accuracy or integrity of any part of the work are appropriately investigated and resolved. All experimental protocols were approved by the Ethics Committee of the First Affiliated Hospital of Chongqing Medical University (No. 2020-10) and conducted according to National Institutes of Health Guide for the Care and Use of Laboratory Animals.
Open Access Statement: This is an Open Access article distributed in accordance with the Creative Commons Attribution-NonCommercial-NoDerivs 4.0 International License (CC BY-NC-ND 4.0), which permits the non-commercial replication and distribution of the article with the strict proviso that no changes or edits are made and the original work is properly cited (including links to both the formal publication through the relevant DOI and the license). See: https://creativecommons.org/licenses/by-nc-nd/4.0/.
References
- World Health Organization (2017). Depression and other common mental disorders: global health estimates. World Health Organization. Available online: https://apps.who.int/iris/handle/10665/254610. License: CC BY-NC-SA 3.0 IGO.
- Ingram A, Saling MM, Schweitzer I. Cognitive side effects of brief pulse electroconvulsive therapy: a review. J ECT 2008;24:3-9. [Crossref] [PubMed]
- Rasmussen KG. Clinical applications of recent research on electroconvulsive therapy. Bull Menninger Clin 2003;67:18-31. [Crossref] [PubMed]
- Salvador Sánchez J, David MD, Torrent Setó A, et al. Electroconvulsive therapy clinical database: Influence of age and gender on the electrical charge. Rev Psiquiatr Salud Ment 2017;10:143-8. [PubMed]
- Ren L, Zhang F, Min S, et al. Propofol ameliorates electroconvulsive shock-induced learning and memory impairment by regulation of synaptic metaplasticity via autophosphorylation of CaMKIIa at Thr 305 in stressed rats. Psychiatry Res 2016;240:123-30. [Crossref] [PubMed]
- Li W, Liu L, Liu YY, et al. Effects of electroconvulsive stimulation on long-term potentiation and synaptophysin in the hippocampus of rats with depressive behavior. J ECT 2012;28:111-7. [Crossref] [PubMed]
- Zhang F, Luo J, Min S, et al. Propofol alleviates electroconvulsive shock-induced memory impairment by modulating proBDNF/mBDNF ratio in depressive rats. Brain Res 2016;1642:43-50. [Crossref] [PubMed]
- Nicoll RA. A Brief History of Long-Term Potentiation. Neuron 2017;93:281-90. [Crossref] [PubMed]
- Stewart C, Jeffery K, Reid I. LTP-like synaptic efficacy changes following electroconvulsive stimulation. Neuroreport 1994;5:1041-4. [Crossref] [PubMed]
- Ren L, Hao X, Min S, et al. Anesthetics alleviate learning and memory impairment induced by electroconvulsive shock by regulation of NMDA receptor-mediated metaplasticity in depressive rats. Neurobiol Learn Mem 2018;155:65-77. [Crossref] [PubMed]
- Liu W, Ge T, Leng Y, et al. The Role of Neural Plasticity in Depression: From Hippocampus to Prefrontal Cortex. Neural Plast 2017;2017:6871089 [Crossref] [PubMed]
- Reid IC, Stewart CA. Seizures, memory and synaptic plasticity. Seizure 1997;6:351-9. [Crossref] [PubMed]
- Plata A, Lebedeva A, Denisov P, et al. Astrocytic Atrophy Following Status Epilepticus Parallels Reduced Ca2+ Activity and Impaired Synaptic Plasticity in the Rat Hippocampus. Front Mol Neurosci 2018;11:215. [Crossref] [PubMed]
- Cercato MC, Vázquez CA, Kornisiuk E, et al. GluN1 and GluN2A NMDA Receptor Subunits Increase in the Hippocampus during Memory Consolidation in the Rat. Front Behav Neurosci 2016;10:242. [PubMed]
- McKay S, Ryan TJ, McQueen J, et al. The Developmental Shift of NMDA Receptor Composition Proceeds Independently of GluN2 Subunit-Specific GluN2 C-Terminal Sequences. Cell Rep 2018;25:841-851.e4. [Crossref] [PubMed]
- Incontro S, Díaz-Alonso J, Iafrati J, et al. The CaMKII/NMDA receptor complex controls hippocampal synaptic transmission by kinase-dependent and independent mechanisms. Nat Commun 2018;9:2069. [Crossref] [PubMed]
- Han Y, Jia Y, Tian J, et al. Urine metabolomic responses to aerobic and resistance training in rats under chronic unpredictable mild stress. PLoS One 2020;15:e0237377 [Crossref] [PubMed]
- Keugong Wado E, Kubicki M, Ngatanko AHH, et al. Anxiolytic and antidepressant effects of Ziziphus mucronata hydromethanolic extract in male rats exposed to unpredictable chronic mild stress: Possible mechanisms of actions. J Ethnopharmacol 2020;260:112987 [Crossref] [PubMed]
- Kala M, Nivsarkar M. Role of cortisol and superoxide dismutase in psychological stress induced anovulation. Gen Comp Endocrinol 2016;225:117-24. [Crossref] [PubMed]
- Mesripour A, Alhimma F, Hajhashemi V. The effect of vitamin B6 on dexamethasone-induced depression in mice model of despair. Nutr Neurosci 2019;22:744-9. [Crossref] [PubMed]
- Zhang QH, Hao JW, Li GL, et al. Long-lasting neurobehavioral alterations in burn-injured mice resembling post-traumatic stress disorder in humans. Exp Neurol 2020;323:113084 [Crossref] [PubMed]
- Niiyama T, Kuroiwa M, Yoshioka Y, et al. Sex Differences in Dendritic Spine Formation in the Hippocampus and Animal Behaviors in a Mouse Model of Hyperthyroidism. Front Cell Neurosci 2020;14:268. [Crossref] [PubMed]
- Liang T, Ma C, Wang T, et al. Galectin-9 Promotes Neuronal Restoration via Binding TLR-4 in a Rat Intracerebral Hemorrhage Model. Neuromolecular Med 2021;23:267-84. [Crossref] [PubMed]
- Harro J. Animal models of depression: pros and cons. Cell Tissue Res 2019;377:5-20. [Crossref] [PubMed]
- Willner P, Towell A, Sampson D, et al. Reduction of sucrose preference by chronic unpredictable mild stress, and its restoration by a tricyclic antidepressant. Psychopharmacology (Berl) 1987;93:358-64. [Crossref] [PubMed]
- Weiner RD, Rogers HJ, Davidson JR, et al. Effects of stimulus parameters on cognitive side effects. Ann N Y Acad Sci 1986;462:315-25. [Crossref] [PubMed]
- Mankad MV, Beyer JL, Krystal A, et al. Clinical Manual of Electroconvulsive Therapy. Washington, DC: American Psychiatric Publishing, Inc., 2010.
- Beale MDJPA. Stimulus Dosing Methods in Electroconvulsive Therapy. Psychiatr Ann 1998;28:510-2. [Crossref]
- Luo J, Min S, Wei K, et al. Propofol interacts with stimulus intensities of electroconvulsive shock to regulate behavior and hippocampal BDNF in a rat model of depression. Psychiatry Res 2012;198:300-6. [Crossref] [PubMed]
- Zhang F, Huang G, Zhu X. Effect of different charges of modified electroconvulsive seizure on the cognitive behavior in stressed rats: Effects of GluR1 phosphorylation and CaMKIIα activity. Exp Ther Med 2019;17:748-58. [PubMed]
- Earl DE, Das P, Gunning WT 3rd, et al. Regulation of Ca2+/calmodulin-dependent protein kinase II signaling within hippocampal glutamatergic postsynapses during flurazepam withdrawal. Neural Plast 2012;2012:405926 [Crossref] [PubMed]
- Schnell E, Sizemore M, Karimzadegan S, et al. Direct interactions between PSD-95 and stargazin control synaptic AMPA receptor number. Proc Natl Acad Sci U S A 2002;99:13902-7. [Crossref] [PubMed]
- Béïque JC, Andrade R. PSD-95 regulates synaptic transmission and plasticity in rat cerebral cortex. J Physiol 2003;546:859-67. [Crossref] [PubMed]
- Zhang Y, Matt L, Patriarchi T, et al. Capping of the N-terminus of PSD-95 by calmodulin triggers its postsynaptic release. EMBO J 2014;33:1341-53. [Crossref] [PubMed]
- Zalcman G, Federman N, Romano A. CaMKII Isoforms in Learning and Memory: Localization and Function. Front Mol Neurosci 2018;11:445. [Crossref] [PubMed]
- Liang J, Kulasiri D, Samarasinghe S. Computational investigation of Amyloid-β-induced location- and subunit-specific disturbances of NMDAR at hippocampal dendritic spine in Alzheimer's disease. PLoS One 2017;12:e0182743 [Crossref] [PubMed]
- O'Leary H, Liu WH, Rorabaugh JM, et al. Nucleotides and phosphorylation bi-directionally modulate Ca2+/calmodulin-dependent protein kinase II (CaMKII) binding to the N-methyl-D-aspartate (NMDA) receptor subunit GluN2B. J Biol Chem 2011;286:31272-81. [Crossref] [PubMed]
- Shonesy BC, Jalan-Sakrikar N, Cavener VS, et al. CaMKII: a molecular substrate for synaptic plasticity and memory. Prog Mol Biol Transl Sci 2014;122:61-87. [Crossref] [PubMed]
- Pi HJ, Otmakhov N, Lemelin D, et al. Autonomous CaMKII can promote either long-term potentiation or long-term depression, depending on the state of T305/T306 phosphorylation. J Neurosci 2010;30:8704-9. [Crossref] [PubMed]
- Sanz-Clemente A, Nicoll RA, Roche KW. Diversity in NMDA receptor composition: many regulators, many consequences. Neuroscientist 2013;19:62-75. [Crossref] [PubMed]
- Yang Q, Zhu G, Liu D, et al. Extrasynaptic NMDA receptor dependent long-term potentiation of hippocampal CA1 pyramidal neurons. Sci Rep 2017;7:3045. [Crossref] [PubMed]
- Erreger K, Dravid SM, Banke TG, et al. Subunit-specific gating controls rat NR1/NR2A and NR1/NR2B NMDA channel kinetics and synaptic signalling profiles. J Physiol 2005;563:345-58. [Crossref] [PubMed]
- Zhou Y, Takahashi E, Li W, et al. Interactions between the NR2B receptor and CaMKII modulate synaptic plasticity and spatial learning. J Neurosci 2007;27:13843-53. [Crossref] [PubMed]
- Barria A, Malinow R. NMDA receptor subunit composition controls synaptic plasticity by regulating binding to CaMKII. Neuron 2005;48:289-301. [Crossref] [PubMed]
- Barcomb K, Hell JW, Benke TA, et al. The CaMKII/GluN2B Protein Interaction Maintains Synaptic Strength. J Biol Chem 2016;291:16082-9. [Crossref] [PubMed]
- Abrams R. Stimulus titration and ECT dosing. J ECT 2002;18:3-9; discussion 14-5. [Crossref] [PubMed]
- Ittasakul P, Likitnukul A, Pitidhrammabhorn U, et al. Stimulus intensity determined by dose-titration versus age-based methods in electroconvulsive therapy in Thai patients. Neuropsychiatr Dis Treat 2019;15:429-34. [Crossref] [PubMed]
- Petrides G, Fink M. The "half-age" stimulation strategy for ECT dosing. Convuls Ther 1996;12:138-46. [PubMed]
- Landry M, Lafrenière S, Patry S, et al. The clinical relevance of dose titration in electroconvulsive therapy: A systematic review of the literature. Psychiatry Res 2020;294:113497 [Crossref] [PubMed]
- Erchinger VJ, Miller J, Jones T, et al. Anterior cingulate gamma-aminobutyric acid concentrations and electroconvulsive therapy. Brain Behav 2020;10:e01833 [Crossref] [PubMed]
- Sarraf N, Badri T, Keshvari N, et al. Comparison of the efficacy and safety of melatonin and memantine in the alleviation of cognitive impairments induced by electroconvulsive therapy: A randomized clinical trial. J Clin Neurosci 2020;74:146-50. [Crossref] [PubMed]
- Yao Z, Guo Z, Yang C, et al. Phenylbutyric acid prevents rats from electroconvulsion-induced memory deficit with alterations of memory-related proteins and tau hyperphosphorylation. Neuroscience 2010;168:405-15. [Crossref] [PubMed]
- Gao X, Zhuang FZ, Qin SJ, et al. Dexmedetomidine protects against learning and memory impairments caused by electroconvulsive shock in depressed rats: Involvement of the NMDA receptor subunit 2B (NR2B)-ERK signaling pathway. Psychiatry Res 2016;243:446-52. [Crossref] [PubMed]
- Brun VH, Ytterbo K, Morris RG, et al. Retrograde amnesia for spatial memory induced by NMDA receptor-mediated long-term potentiation. J Neurosci 2001;21:356-62. [Crossref] [PubMed]
- McDaniel WW, Sahota AK, Vyas BV, et al. Ketamine appears associated with better word recall than etomidate after a course of 6 electroconvulsive therapies. J ECT 2006;22:103-6. [Crossref] [PubMed]
- Gregory-Roberts EM, Naismith SL, Cullen KM, et al. Electroconvulsive therapy-induced persistent retrograde amnesia: could it be minimised by ketamine or other pharmacological approaches? J Affect Disord 2010;126:39-45. [Crossref] [PubMed]
- Ito Y, Izumi H, Sato M, et al. Suppression of parasympathetic reflex vasodilatation in the lower lip of the cat by isoflurane, propofol, ketamine and pentobarbital: implications for mechanisms underlying the production of anaesthesia. Br J Anaesth 1998;81:563-8. [Crossref] [PubMed]
- Kingston S, Mao L, Yang L, et al. Propofol inhibits phosphorylation of N-methyl-D-aspartate receptor NR1 subunits in neurons. Anesthesiology 2006;104:763-9. [Crossref] [PubMed]
- Kotani Y, Shimazawa M, Yoshimura S, et al. The experimental and clinical pharmacology of propofol, an anesthetic agent with neuroprotective properties. CNS Neurosci Ther 2008;14:95-106. [Crossref] [PubMed]
- Hao X, Zhu X, Li P, et al. NMDA receptor antagonist enhances antidepressant efficacy and alleviates learning-memory function impairment induced by electroconvulsive shock with regulating glutamate receptors expression in hippocampus. J Affect Disord 2016;190:819-27. [Crossref] [PubMed]
- Bauer J, Hageman I, Dam H, et al. Comparison of propofol and thiopental as anesthetic agents for electroconvulsive therapy: a randomized, blinded comparison of seizure duration, stimulus charge, clinical effect, and cognitive side effects. J ECT 2009;25:85-90. [Crossref] [PubMed]
(English Language Editor: J. Jones)