MicroRNA-146a-5p alleviates lipopolysaccharide-induced NLRP3 inflammasome injury and pro-inflammatory cytokine production via the regulation of TRAF6 and IRAK1 in human umbilical vein endothelial cells (HUVECs)
Introduction
Accounting for 17.3 million deaths per year globally, cardiovascular disease is one of the leading causes of mortality worldwide. Along with population growth, an aging population, and lifestyle changes, it is estimated that the number of deaths from cardiovascular disease will exceed 23.6 million by 2030 (1). Atherosclerosis (AS) is the common pathological basis of many cardiovascular diseases, including coronary heart diseases, myocardial infarction, and peripheral vascular diseases. Exposure to atherosclerosis risk factors, such as dyslipidemia or pro-inflammatory mediator, in the context of disturbed flow, activates the inflammatory process and perturbs the homeostatic properties of the endothelial cells (ECs), which triggers the initial steps of atherosclerosis (2,3). Inflammatory activation and chronic endothelial damage are closely linked, and create a vicious cycle that leads to the processes that determine the initiation and progression of atherosclerosis. Thus, key molecules or the respective inflammatory signaling pathways responsible for the formation and development of atherosclerosis need to be identified, as they may subsequently provide new targeted treatment options that more effectively prevent cardiovascular disease (4,5).
The NOD-like receptor family pyrin domain-containing protein 3 (NLRP3) inflammasome is an intracellular complex that includes the NLRP3 protein, apoptosis-related speckle-like protein (ASC) and caspase-1 precursor, activates caspase-1 and facilitates the maturation and secretion of pro-inflammatory cytokines interleukin-1β (IL-1β) and IL-18, eventually induces inflammation (6,7). A number of endogenous pathogenic signals exist abundantly in atherosclerotic lesions, such as oxidized low-density lipoprotein (ox-LDL) and cholesterol crystals, activate the NLRP3 inflammasome (8-10), which participate in the endothelial inflammatory response and contribute significantly to the development and progression of atherosclerosis (11). Research has shown that a deficiency of NLRP3 inflammasome pathway proteins in bone marrow significantly reduces the development of atherosclerotic lesions compared to that in Ldlr−/− mice with wild-type bone marrow transplantation (8). However, the mechanistic relationship between inflammation and atherosclerosis induced by NLRP3 inflammasome is poorly understood.
Microribonucleic acids (miRNAs) are endogenous small non-coding RNAs (17–25 nucleotides in length) that negatively regulate the post-transcriptional gene by directly binding to the 3'-untranslated region (3'-UTR) of the target gene (12,13). A number of miRNAs that participate in the regulation of many cellular and developmental processes have been extensively studied (14,15). In humans, differential miRNA expression patterns have been implicated in various diseases or pathological states, and different clinical sample types, such as salivary glands or peripheral blood mononuclear cells (PBMCs), have been analyzed (16,17). Previous studies have suggested that miR-146a plays an important role in regulating the inflammatory response. For example, research has shown that miR-146a attenuates cytokine signaling by impairing the nuclear factor-κB (NF-κB) pathway in human hepatic stellate cell (18). Additionally, the expression level of miR-146a was significantly increased in human monocytes by lipopolysaccharide, tumor necrosis factor alpha (TNF-α), and IL-1β (19). In recent years, studies have shown that some miRNAs play a significant role in driving endothelial inflammation and may represent a promising therapeutic approach for atherosclerosis (20,21). Additionally, miR-146a-5p has been found to be involved in the regulation of inflammation and cardiac energy metabolism (22), which suggests that it may be a promising therapeutic approach for atherosclerosis. In the present study, we demonstrated that miR-146a-5p alleviated lipopolysaccharide (LPS) induced NLRP3 inflammasome injury and pro-inflammatory cytokine secretion by down-regulating tumor necrosis factor receptor associated factor 6 (TRAF6) and interleukin-1-receptor-associated kinase 1 (IRAK1) in human umbilical vein endothelial cells (HUVECs).
We present the following article in accordance with the MDAR reporting checklist (available at https://dx.doi.org/10.21037/atm-21-3903).
Methods
Patients
Peripheral blood monouclear cells (PBMCs) were collected from 40 patients, including 20 acute myocardial infarction (AMI) patients and 20 normal coronary artery (NCA) patients, who were admitted to Meizhou People’s Hospital (Huangtang Hospital) between June 2017 and December 2017. AMI was defined according to the 2012 Joint ESC/ACCF/AHA/WHF Task Force on the Third universal definition of myocardial infarction (23). NCA patients were defined as no stenosis in coronary arteries by coronary angiography and served as controls in this study. All procedures in this study were in accordance with the ethical guidelines outlined in the Helsinki Declaration (as revised in 2013) and complies with the Ethics Committee of Meizhou People’s Hospital (No. 2018-C-11). Signed informed consent was obtained from each participant at the time of enrollment.
Cell culture
HUVECs were obtained from the Chinese Academy of Sciences Cell Bank (Shanghai, China). The cells were maintained in endothelial cell medium (Sciencell Research Laboratories, 1001) supplemented with endothelial cell growth supplement, 5% fetal bovine serum, and 1% penicillin/streptomycin. The cells were incubated at 37 °C under 5% carbon dioxide (CO2) in Thermo Scientific™ Steri-Cycle CO2 incubators.
Transfection
The HUVECs were seeded in a 12-well plate 1 day in advance. The 30 nM miR-146a-5p mimic and negative control (mimic NC) were transfected into cells using Lipofectamine™ 3000 Transfection Reagent (ThermoFisher, Invitrogen, L3000015) for 48 h in accordance with the manufacturer’s recommendations. 50 nM of TRAF6 and IRAK1 small-interfering RNA (siRNA), and a negative control were transfected into the cells in the same manner. No obvious cytotoxicity was observed in the transfected cells using Lipofectamine™ 3000 Transfection Reagent. The cells were then cultured for an additional 24 h with 100 ng/mL of LPS (Sigma-Aldrich) to collect RNA, protein, or cellular supernatant.
Quantitative real-time PCR
Total RNA was isolated from the cell lysates using TRIzol reagent (Tiangen, DP430) in accordance with the manufacturer’s instructions. A PrimeScript RT reagent Kit (TaKaRa, RR047) and Mir-X miRNA First-Strand Synthesis Kit (TaKaRa, 638313) were used to synthesize complementary deoxyribonucleic acid (cDNA) for the mRNA or miRNA analyses. TB Green Premix Ex Taq. II (TaKaRa, RR820) and TB Green Advantage qPCR Premix (TaKaRa, 639676) were used to amplify the desired gene or miRNA, respectively. Quantitative real-time polymerase chain reactions (qPCRs) were conducted using a 7500 Real-Time PCR System (Applied Biosystems). Glyceraldehyde-3-phosphate dehydrogenase (GAPDH) and U6 served as the internal controls for the mRNA and miRNA detection, respectively. Table 1 shows the primer sequences of all the detected genes. No amplifications of the non-specific products were observed in the dissociation curve of the reaction. The data were normalized to GAPDH or U6 expression levels, and the relative expression levels of the genes were calculated using 2−△△Ct method, where Ct represents the threshold cycle. Three independent experiments were conducted.
Table 1
Gene name | Sequences |
---|---|
|
Forward: 5'-ATGACATCAAGAAGGTGGTG-3' |
Reverse: 5'-CATACCAGGAAATGAGCTTG-3' | |
|
Forward: 5'-ATGCTCAGGAGCCCTGTAATG-3' |
Reverse: 5'-CCCCACACTTCAACAGGAGT-3' | |
|
Forward: 5'-GTGTTTCGAATCCCACTGTG-3' |
Reverse: 5'-TCTGCTTCTCACGTACTTTCTG-3' | |
|
Forward: 5'-TTGAAGGACAAACCGAAGG-3' |
Reverse: 5'-GGAAGAGCAGAAAGCGATAA-3' | |
|
Forward: 5'-ATGCACCTGTACGATCACTGA-3' |
Reverse: 5'-ACAAAGGACATGGAGAACACC-3' | |
|
Forward: 5'-TGCAATAACCACCCCTGACC-3' |
Reverse: 5'-ATTTGCCGAAGAGCCCTCAG-3' | |
|
Forward: 5'-TGCACTTTGGAGTGATCGGC-3' |
Reverse: 5'-ACTCGGGGTTCGAGAAGATG-3' | |
|
Forward: 5'-GGAACGATACAGAGAAGATTAGC-3' |
Reverse: 5'-TGGAACGCTTCACGAATTTGCG-3' | |
|
Forward: 5'-TGAGAACTGAATTCCATGG-3' |
Western blot
Protein samples (20 µg) were separated by sodium dodecyl-sulfate polyacrylamide gel electrophoresis (SDS-PAGE), and the proteins were then transferred into polyvinylidene fluoride blots. After being blocked by 5% milk, the blots were incubated with an antibody against target proteins at 4 °C overnight. Antibodies against p-NF-κB (CST, #3033) was obtained from Cell Signaling Technology (Danvers, MA). Antibodies against NF-κB antibody (ab16502), NLRP3 (ab214185), Caspase-1 (ab207802), IL-1β (ab229696), TRAF6 (ab40675), IRAK1 (ab238) were purchased from Abcam (Cambridge, UK). The α-Tubulin antibody (Sigma, T5168) was incubated as a loading control. These blots were further incubated with horseradish peroxidase (HRP)-conjugated secondary antibody (Abcam, ab6789 and ab6721), and exposed on a ChemiDoc MP imaging system (BIO-RAD) with an enhanced chemiluminescence (ECL) solution.
ELISA
After miRNA mimic or siRNA transfection, the HUVECs were stimulated with LPS (100 ng/mL) for 24 h, and cellular supernatant was then collected for the enzyme-linked immunoassay (ELISA). A human IL-6 ELISA kit (Dakewe Biotech, Shenzhen, China) was used to detect the IL-6 concentration. ELISA was performed in accordance with the manufacturer’s instructions. Three independent experiments were conducted, and a standard curve was included in each experiment.
Luciferase reporter assays
The HEK293 cells were seeded into a 12-well plate and incubated overnight to achieve approximately 80% confluence. A TRAF6/IRAK1-luciferase-reporter recombinant plasmid (psiCHECK2-TRAF6/IRAK1 3'-UTR vector), containing 2 miR-146a-5p binding motifs, was transfected into HEK293 cells together with miR-146a-5p mimic or mimic NC using Lipofectamine 3000 (Invitrogen). As the control, recombinant plasmid (psiCHECK2-TRAF6/IRAK1 3'-UTR mutant vector), without miR-146a-5p binding motifs, was transfected into the HEK293 cells in the same manner. After transfection for 24 h, the cell lysates were obtained to detect the luciferase activity using a Dual-Luciferase Reporter Assay System (Promega, E1910, Madison, WI). The results are presented as the relative luciferase activity (the ratio of Renilla luciferase to Firefly luciferase).
Quantification and statistics
SPSS software 20.0 was used to conduct the statistical analysis. Image J software (NIH, Bethesda, MD) was used to analyze the exposure intensity of the targeted blots. Prizm software 6.0 (GraphPad Software) and Adobe Illustrator CS6 were used to draw and edit the charts. All the data are presented as the mean ± standard deviation (SD). The qPCR, Western blot, and ELISA data were analyzed by the non-parametrical Mann-Whitney U test (2 groups) or the Kruskal-Wallis test (more than 2 groups). A P<0.05 was considered statistically significant.
Results
LPS-induced inflammation injury and miR-146a-5p expression in HUVECs
First, we compared the expression of miR-146a-5p in PBMCs obtained from AMI patients and NCA patients by qPCRs. The mean age of the AMI patients was 65.2±7.6 years, 70% (n=14) patients were males, and the mean age of the NCA patients was 64.8±12.9 years, 75% (n=15) were males, respectively. The AMI patients had a higher expression level of miR-146a-5p than the HC patients (see Figure 1A). It is well known that inflammatory activation has a critical role in AS development and cardiovascular diseases. In the present study, LPS was used as an inflammatory stimulant, and the HUVECs were treated with different concentrations of LPS (0, 25, 50, 100, 200, or 500 ng/mL). We further investigated the expression of miR-146a-5p and pro-inflammatory cytokines (IL-6, IL-1β and TNF-α) in LPS-treated HUVECs. Compared to the untreated cells, the expression levels of miR-146a-5p and pro-inflammatory cytokine were elevated significantly following 25 ng/mL of LPS treatment for 24 h in the HUVECs (see Figure 1B,1C). The secretion of interleukin-6 (IL-6) from the HUVECs was significantly increased after LPS treatment for 24 h (see Figure 1D). Further, HUVECs were treated with 100 ng/mL LPS for 24 or 48 h, and the mRNA expression level of the pro-inflammatory cytokine were detected (see Figure 1E). LPS was shown to promote pro-inflammatory cytokine expression in a dose-dependent manner (with an optimum concentration of 100 ng/mL for 24 h).
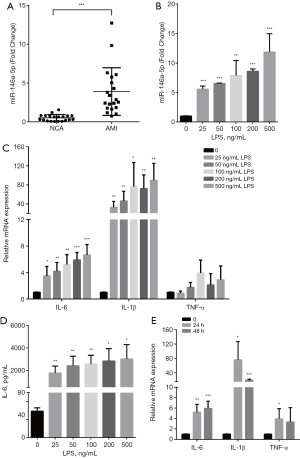
Regulation of the NF-κB/NLRP3 signaling pathway in HUVECs stimulated with LPS
Next, the HUVECs were stimulated with LPS for 24 h, the cell viability and NLRP3 inflammasome were then detected. No significant inhibition was observed in the cell viability of the HUVECs after LPS treatment (see Figure 2A). Further, the mRNA and the protein levels of p-NF-κB/ NF-κB, NLRP3, Caspase-1, and IL-1β were significantly increased in LPS-treated cells than untreated cells (see Figure 2B,2C). These results showed that LPS regulated the NF-κB/NLRP3 signaling pathway in HUVECs.
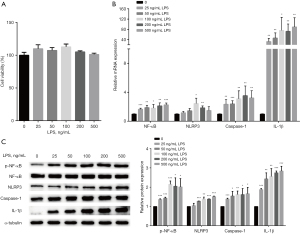
Overexpression of miR-146a-5p reduced LPS-induced pro-inflammatory cytokine expression and secretion
After transfection with a miR-146a-5p mimic for 48 h, the expression of miR-146a-5p was significantly up-regulated in the HUVECs (see Figure 3A). The overexpression of miR-146a-5p led to a significant decrease in the mRNA levels of IL-1β and IL-6 in the HUVECs regardless of whether the cells were subject to LPS treatment or not (see Figure 3B). The overexpression of miR-146a-5p also caused a significant decline in the secretion of IL-6 in HUVECs (see Figure 3C). The results showed that miR-146a-5p overexpression in HUVECs led to a significant decrease in pro-inflammatory cytokine production.
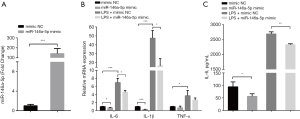
MiR-146a-5p inhibited cytokine production by down-regulating the NF-κB/NLRP3 signaling pathway
We then assessed whether miR-146a-5p overexpression inhibited pro-inflammatory cytokine expression by regulating the NF-κB/NLRP3 signaling pathway. After transfection with a miR-146a-5p mimic for 48 h, the HUVECs were treated with LPS. The mRNA expression of the NLRP3 inflammasome pathway genes were tested by qPCRs. The overexpression of miR-146a-5p led to a significant decrease in the mRNA levels of NF-κB, NLRP3, Caspase-1, and IL-1β regardless of whether the cells were subject to LPS treatment or not (see Figure 4A).The NF-κB, phospho-NF-κB, NLRP3, Caspase-1, and IL-1β protein levels were measured by western blot assays. We found that miR-146a-5p overexpression also down-regulated the protein levels of phospho-NF-κB, NLRP3, Caspase-1, and IL-1β in the HUCVCs (see Figure 4B). These results implied that miR-146a-5p inhibited cytokines production by down-regulating the NF-κB/NLRP3 signaling pathway.
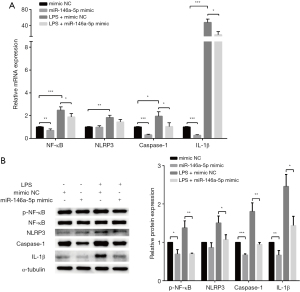
MiR-146a-5p down-regulated the NF-κB/NLRP3 signaling pathway by targeting the TRAF6 and IRAK1 gene
We sought to confirm the potential mechanism by which miR-146a-5p alleviates LPS-induced NLRP3 inflammasome injury and pro-inflammatory cytokine secretion. TargetScan software (http://www.targetscan.org/) was used to predict the miR-146a-5p target. The prediction results suggested that miR-146a-5p has 3'-UTR binding sites on TRAF6 and IRAK1, both upstream regulators of the NF-κB signaling pathway. The mRNA and protein levels of TRAF6 and IRAK1 in the HUVECs transfected with the miR-146-5p mimic or NC were evaluated by qPCRs and western blot assays, respectively. The results showed that TRAF6 and IRAK1 in HUVECs were both down-regulated after transfection with a miR-146-5p mimic compared to the cells transfected with a mimic NC (see Figure 5A,5B). Luciferase reporter assays were used to verify whether miR-146-5p directly targets the 3'-UTR regions of TRAF6 and IRAK1 to regulate their expression. The results revealed that luciferase activity was significantly reduced in cells co-transfected with a miR-146-5p mimic and wild-type TRAF6 or IRAK1 3'-UTR compared to luciferase activity in the mimic NC co-transfected cells; however, luciferase activity was not affected by co-transfection with a miR-146-5p mimic and the mutant TRAF6 or IRAK1 3'-UTR (see Figure 5C,5D).
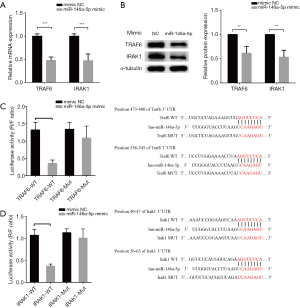
Knockdown of TRAF6- and IRAK1-suppressed LPS-induced NF-κB/NLRP3 signaling pathway up-regulation and pro-inflammatory cytokine production
Finally, we explored the molecular mechanism by which miR-146-5p regulates the NF-κB/NLRP3 signaling pathways. The above-mentioned results confirmed that the overexpression of miR-146-5p down-regulated the NF-κB/NLRP3 signaling pathways in HUVECs. Next, the cells were transfected with si-TRAF6 or si-IRAK1 for 48 h. The mRNA and protein expression levels of TRAF6 and IRAK1 were significantly reduced after transfection (see Figure 6A,6B). We observed that the mRNA expression levels of NF-κB, NLRP3, Caspase-1, and pro-inflammatory cytokine (IL-6, IL-1β, and TNF-α) were all down-regulated after TRAF6 or IRAK1 knockdown in the HUVECs regardless of whether the cells were subject to LPS treatment or not (see Figure 6C). The secretion of IL-6 from the HUVECs was found to decline significantly after transfection with si-TRAF6 or si-IRAK1 (see Figure 6D). The western blot analysis revealed that the protein levels of p-NF-κB, NLRP3, Caspase-1, and IL-1β also were significantly reduced by TRAF6 or IRAK1 knockdown (see Figure 6E). These data indicate that miR-146-5p appears to suppress NF-κB/NLRP3 signaling pathway and pro-inflammatory cytokine production by regulating TRAF6 and IRAK1 expression in HUVECs.
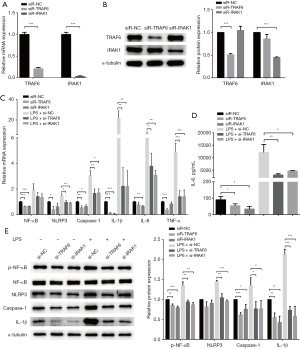
Discussion
Endothelial inflammatory activation in the arterial vasculature is an initiator of the pathobiology of atherosclerotic cardiovascular disease (3). In lesion-prone regions of the artery, the effects of pro-inflammatory agonists, oxidized lipoproteins (ox-LDL), and biomechanical stimulation by disturbed blood flow result in endothelial pro-inflammatory activation. Following by the expression of adhesion molecules, membrane-associated chemokines and prothrombotic mediators are driven in ECs (24-26). The monocytes and lymphocytes then migrate into vessel walls to differentiate into macrophages (27,28). A complex immunoregulatory network is established by the synergistic interaction of cells (including activated endothelium, macrophages, lymphocytes, and smooth muscle cells) in the developing atherosclerotic lesions. Finally, the production of inflammatory cytokines, growth factors, and reactive oxygen species (ROS) in the lining of the arterial vasculature perpetuates a chronic pro-inflammatory state and promotes the progression of atherosclerosis (3,29). Thus, reducing the endothelial pro-inflammatory activation could serve as a therapeutic strategy for atherosclerosis. In this study, we constructed an inflammatory response in HUVECs by LPS stimulation to mimic the inflammatory injury that occurs during AS. Further experiments revealed that miR-146a-5p overexpression repressed LPS-mediated pro-inflammatory cytokine production by regulating the activation of the NF-κB and NLRP3 signaling pathways. Finally, we confirmed that miR-146a-5p suppressed the NF-κB/NLRP3 signaling pathway by targeting TRAF6 and IRAK1 3'-UTR. The results revealed that increasing miR-146a-5p levels might be a novel strategy for the prevention and treatment of AS.
A number of pathophysiologic stimuli signal for pro-inflammatory activation in the arterial endothelium are predominantly through activating the pleiotropic transcription factor NF-κB, which leads to the expression of multiple pro-inflammatory and pro-adhesive genes in ECs (30-32). NF-κB signaling is thought to play a vital role in the activation of endothelial inflammation during atherosclerosis. The activity of NF-κB is mainly dependent on its phosphorylation and nuclear translocation (33). Research suggests that the selective inhibition of NF-κB in ECs reduces and stabilizes atherosclerotic plaques in mice models. The mechanism is associated with the decreased recruitment of macrophages, which occurs by reducing the adhesion molecules and pro-inflammatory cytokine expression (32,34). MiR-146a has been shown to inhibit NF-κB phosphorylation to negatively regulate LPS-induced inflammatory cytokine production in ECs (35). Similarly, we found miR-146a-5p overexpression repressed the level of phospho-NF-κB in HUVECs, resulting in a significant decrease in pro-inflammatory cytokine expression and secretion. The results revealed that miR-146a-5p can reduce the expression of pro-inflammatory factors by inhibiting NF-κB activation, and that enhancing the expression of miR-146a-5p might antagonize atherosclerosis.
NLRP3 inflammasome, an intracellular complex, which cleave pro-caspase-1 to active caspase-1, and then converts pro-IL-1β and pro-IL-18 to its mature forms (36). NLRP3 inflammasome can be activated by many danger signals, such as oxidative stress, mitochondrial dysfunction, lysosome rupture, and ox-LDL, which are linked to an increased risk of AS. Studies had suggested that NLRP3 is up-regulated in the atherosclerotic plaques of AS patients, and is related to the severity and prognosis of AS (37,38). The inhibition of NLRP3 signaling reduced pro-inflammatory cytokine production and led to plaque stabilization in mice with AS (39). Those researches revealed that NLRP3 inflammasome contributes to the progression of atherosclerosis, and silencing it results in the stabilization of atherosclerotic plaque. Several miRNA had been implicated in regulating the expression and activation of the NLRP3 inflammasome. Zhou et al. confirmed that miR-495 suppressed the NLRP3 inflammasome signaling pathway by targeting NLRP3 3'-UTR (40). MiR-223 and miR-374a-5p were also found to have a negative regulatory effect on the activation of NLRP3 inflammatory signals (41,42). In addition, the expression of NLRP3 and IL-1β has proven to be regulated by NF-κB activation (43,44). In the present study, we observed that miR-146a-5p repressed the NLRP3 inflammasome signaling pathway and pro-inflammatory cytokine production by decreasing the phospho-NF-κB level in ECs.
TRAF6 and IRAK1, which are multifunctional signal transduction molecules, are thought to play an essential role in the activation of the NF-κB signaling pathway and the regulation of inflammation. NF-κB activation depends on the recruitment of TRAF6 to IRAK1 and subsequent complex formation (31,45). Studies have confirmed that TRAF6 and IRAK1 were regulated by multiple miRNAs. Overexpressed miR-2187 was found to reduce inflammatory factor production and antiviral genes by targeting TRAF6 to avoid an excessive inflammatory response (46). Elevated miR-223 inhibited the pro-inflammatory response by down-regulating IRAK-1 in macrophages (47). Studies had shown that miR-146a-5p inhibited the proliferation, invasion, and migration of human breast cancer cells and suppressed the epithelial–mesenchymal transition of trophoblasts by directly reducing IRAK1 and TRAF6 expression at the post-transcriptional level (48,49). However, there have been rare reports of miR-146a-5p regulating the down-stream signaling pathways by directly targeting TRAF6 and IRAK1 in vascular ECs. Thus, luciferase reporter assays and molecular biology experiments were performed in this study, and similar to previous findings, we found that miR-146a-5p directly targeted TRAF6 and IRAK1 genes to reduce NF-κB activation and the NLRP3 inflammasome level in HUVECs.
To confirm the contribution of TRAF6 and IRAK1 regulation on the activation of the NF-κB/NLRP3 inflammasome signaling pathway, si-TRAF6 and si-IRAK1 were transfected into HUVECs to knockdown the expression of TRAF6 and IRAK1, respectively. We further observed that the expression levels of p-NF-κB, NLRP3, Caspase-1, pro-inflammatory cytokine (IL-6, IL-1β, and TNF-α), and the secretion of IL-6 were all down-regulated following TRAF6 or IRAK1 knockdown in HUVECs regardless of whether the cells were subject to LPS treatment or not. Previous research has indicated that TRAF6 or IRAK1 knockdown inhibited pro-inflammatory cytokine secretion by limiting the levels of phospho-NF-κB (18), and our results were consistent with those previous studies. Our data indicated that miR-146-5p appeared to alleviate NF-κB/NLRP3 signaling pathway and pro-inflammatory cytokine production by regulating TRAF6 and IRAK1 expression in HUVECs.
It is well known that miRNAs are important regulators of the pathophysiological cellular effects and molecular signaling pathways involved in atherosclerosis. Numerous studies have shown that miRNAs alter the balance of atherosclerotic plaque progression by regulating the cellular adhesion, proliferation, lipid uptake, and efflux, and the generation of inflammatory mediators (50). Studies have highlighted that miR-181b and miR-146a, as cytokine-responsive and atheroprotective miRNAs, regulate EC inflammation and plaque progression by targeting NF-κB signaling (51). MiR-126 has been shown to inhibit the expression of vascular adhesion molecule-1 (VCAM-1) to limit leukocyte adhesion in ECs (52). MiR-33 has been shown to target the cholesterol transporter ABC transporter A1 (ABCA1) to repress cholesterol efflux and promote the progress of AS (53). Our findings revealed that miR-146a-5p overexpression in HUVECs repressed LPS-mediated NF-κB/NLRP3 signaling pathway and pro-inflammatory cytokines production by regulating the IRAK1 and TRAF6. Similarly, a previous study showed that miR-146a-5p repressed chronic constriction injury-induced mechanical and thermal hyperalgesia by targeting IRAK1 and TRAF6 in neurons (54). MiR-146a-5p has also been reported to suppress LPS-mediated pro-inflammatory cytokine production and cell activation of human hepatic stellate cells by down-regulating TRAF6 expression (18).
In conclusion, this study showed that miR-146a-5p, as a regulator of TRAF6 and IRAK1 expression, alleviated the LPS-induced activation of the NF-κB/NLRP3 inflammasome signaling pathway, and reduced the production of pro-inflammatory cytokines in HUVECs. The findings of this study have been depicted in a schematic illustration (see Figure 7). Overall, our results increase understandings of miR-146a-5p function, and provide insights into the regulatory network of miR-146a-5p-mediated immune responses against endothelial pro-inflammatory activation and dysfunction. Further animal model investigations examining the anti-inflammatory and anti-atherosclerotic effects of miR-146a-5p in regulating activation of NF-κB/NLRP3 inflammasome should be conducted in the future.
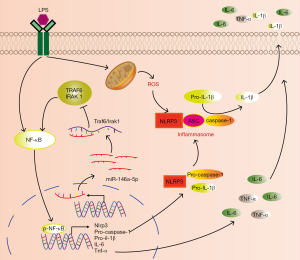
Acknowledgments
The authors would like to thank other colleagues whom were not listed in the authorship of Research Experimental Center, Meizhou People’s Hospital (Huangtang Hospital), for their helpful comments on the manuscript.
Funding: This work was supported by grants from the National Natural Science Foundation for Young Scientists of China (82002216 and 82000410), the Medical Scientific Research Foundation of Guangdong Province (A2020418 and A2021144), the Guangdong Provincial Key Laboratory of Precision Medicine and Clinical Translation Research of Hakka Population (2018B030322003), and the Scientific Research and Cultivation Project of Meizhou People’s Hospital (PY-A2019001, PY-C2019013, and PY-C2021010).
Footnote
Reporting Checklist: The authors have completed the MDAR reporting checklist. Available at https://dx.doi.org/10.21037/atm-21-3903
Data Sharing Statement: Available at https://dx.doi.org/10.21037/atm-21-3903
Conflicts of Interest: All authors have completed the ICMJE uniform disclosure form (available at https://dx.doi.org/10.21037/atm-21-3903). ZZ reports support for the present manuscript from Scientific Research and Cultivation Project of Meizhou People’s Hospital (grants no. PY-A2019001). SL reports support for the present manuscript from National Natural Science Foundation for Young Scientists of China (grants no. 82000410). QD reports support for the present manuscript from Medical Scientific Research Foundation of Guangdong Province (grants no. A2021144) and Scientific Research and Cultivation Project of Meizhou People’s Hospital (grants no. PY-C2021010). JH reports support for the present manuscript from National Natural Science Foundation for Young Scientists of China (grants no. 82002216), Medical Scientific Research Foundation of Guangdong Province (grants no. A2020418), and Scientific Research and Cultivation Project of Meizhou People’s Hospital (grants no. C2019013). The other authors have no conflicts of interest to declare.
Ethical Statement: The authors are accountable for all aspects of the work in ensuring that questions related to the accuracy or integrity of any part of the work are appropriately investigated and resolved. The present study was approved by the Ethics Committee for Research of Meizhou People’s Hospital (No. 2018-C-11) and was conducted in accordance with the Declaration of Helsinki (as revised in 2013). All patients signed an informed consent before participating in the study.
Open Access Statement: This is an Open Access article distributed in accordance with the Creative Commons Attribution-NonCommercial-NoDerivs 4.0 International License (CC BY-NC-ND 4.0), which permits the non-commercial replication and distribution of the article with the strict proviso that no changes or edits are made and the original work is properly cited (including links to both the formal publication through the relevant DOI and the license). See: https://creativecommons.org/licenses/by-nc-nd/4.0/.
References
- Roth GA, Forouzanfar MH, Moran AE, et al. Demographic and epidemiologic drivers of global cardiovascular mortality. N Engl J Med 2015;372:1333-41. [Crossref] [PubMed]
- Libby P, Buring JE, Badimon L, et al. Atherosclerosis. Nat Rev Dis Primers 2019;5:56. [Crossref] [PubMed]
- Gimbrone MA Jr, García-Cardeña G. Endothelial Cell Dysfunction and the Pathobiology of Atherosclerosis. Circ Res 2016;118:620-36. [Crossref] [PubMed]
- Mai W, Liao Y. Targeting IL-1β in the Treatment of Atherosclerosis. Front Immunol 2020;11:589654 [Crossref] [PubMed]
- He L, Weng H, Li Q, et al. Lactucopicrin Inhibits Cytoplasmic Dynein-Mediated NF-κB Activation in Inflammated Macrophages and Alleviates Atherogenesis in Apolipoprotein E-Deficient Mice. 2021;65:e2000989
- He Y, Hara H, Núñez G. Mechanism and Regulation of NLRP3 Inflammasome Activation. Trends Biochem Sci 2016;41:1012-21. [Crossref] [PubMed]
- Stutz A, Golenbock DT, Latz E. Inflammasomes: too big to miss. J Clin Invest 2009;119:3502-11. [Crossref] [PubMed]
- Duewell P, Kono H, Rayner KJ, et al. NLRP3 inflammasomes are required for atherogenesis and activated by cholesterol crystals. Nature 2010;464:1357-61. [Crossref] [PubMed]
- Samstad EO, Niyonzima N, Nymo S, et al. Cholesterol crystals induce complement-dependent inflammasome activation and cytokine release. J Immunol 2014;192:2837-45. [Crossref] [PubMed]
- Rhoads JP, Lukens JR, Wilhelm AJ, et al. Oxidized Low-Density Lipoprotein Immune Complex Priming of the Nlrp3 Inflammasome Involves TLR and FcγR Cooperation and Is Dependent on CARD9. J Immunol 2017;198:2105-14. [Crossref] [PubMed]
- Takahashi M. NLRP3 inflammasome as a key driver of vascular disease. Cardiovasc Res 2021; Epub ahead of print. [Crossref] [PubMed]
- Bartel DP. MicroRNAs: target recognition and regulatory functions. Cell 2009;136:215-33. [Crossref] [PubMed]
- Krol J, Loedige I, Filipowicz W. The widespread regulation of microRNA biogenesis, function and decay. Nat Rev Genet 2010;11:597-610. [Crossref] [PubMed]
- Constantin L, Constantin M, Wainwright BJ. MicroRNA Biogenesis and Hedgehog-Patched Signaling Cooperate to Regulate an Important Developmental Transition in Granule Cell Development. Genetics 2016;202:1105-18. [Crossref] [PubMed]
- Katsaraki K, Karousi P, Artemaki PI, et al. MicroRNAs: Tiny Regulators of Gene Expression with Pivotal Roles in Normal B-Cell Development and B-Cell Chronic Lymphocytic Leukemia. Cancers (Basel) 2021;13:593. [Crossref] [PubMed]
- Mehta A, Baltimore D. MicroRNAs as regulatory elements in immune system logic. Nat Rev Immunol 2016;16:279-94. [Crossref] [PubMed]
- Fridrichova I, Zmetakova I. MicroRNAs Contribute to Breast Cancer Invasiveness. Cells 2019;8:1361. [Crossref] [PubMed]
- Chen Y, Zeng Z, Shen X, et al. MicroRNA-146a-5p Negatively Regulates Pro-Inflammatory Cytokine Secretion and Cell Activation in Lipopolysaccharide Stimulated Human Hepatic Stellate Cells through Inhibition of Toll-Like Receptor 4 Signaling Pathways. Int J Mol Sci 2016;17:1076. [Crossref] [PubMed]
- Taganov KD, Boldin MP, Chang KJ, et al. NF-kappaB-dependent induction of microRNA miR-146, an inhibitor targeted to signaling proteins of innate immune responses. Proc Natl Acad Sci U S A 2006;103:12481-6. [Crossref] [PubMed]
- Menghini R, Casagrande V, Marino A, et al. MiR-216a: a link between endothelial dysfunction and autophagy. Cell Death Dis 2014;5:e1029 [Crossref] [PubMed]
- Schober A, Weber C. Mechanisms of MicroRNAs in Atherosclerosis. Annu Rev Pathol 2016;11:583-616. [Crossref] [PubMed]
- Demkes CJ, van Rooij E. MicroRNA-146a as a Regulator of Cardiac Energy Metabolism. Circulation 2017;136:762-4. [Crossref] [PubMed]
- Thygesen K, Alpert JS, Jaffe AS, et al. Third universal definition of myocardial infarction. Circulation 2012;126:2020-35. [Crossref] [PubMed]
- Steinberg D, Witztum JL. Oxidized low-density lipoprotein and atherosclerosis. Arterioscler Thromb Vasc Biol 2010;30:2311-6. [Crossref] [PubMed]
- Zhong T, Li Y, He X, et al. Adaptation of endothelial cells to shear stress under atheroprone conditions by modulating internalization of vascular endothelial cadherin and vinculin. Ann Transl Med 2020;8:1423. [Crossref] [PubMed]
- Noguchi N, Jo H. Redox going with vascular shear stress. Antioxid Redox Signal 2011;15:1367-8. [Crossref] [PubMed]
- Eid RE, Rao DA, Zhou J, et al. Interleukin-17 and interferon-gamma are produced concomitantly by human coronary artery-infiltrating T cells and act synergistically on vascular smooth muscle cells. Circulation 2009;119:1424-32. [Crossref] [PubMed]
- Escárcega RO, Lipinski MJ, García-Carrasco M, et al. Inflammation and atherosclerosis: Cardiovascular evaluation in patients with autoimmune diseases. Autoimmun Rev 2018;17:703-8. [Crossref] [PubMed]
- Fan J, Watanabe T. Inflammatory reactions in the pathogenesis of atherosclerosis. J Atheroscler Thromb 2003;10:63-71. [Crossref] [PubMed]
- Collins T, Cybulsky MI. NF-kappaB: pivotal mediator or innocent bystander in atherogenesis? J Clin Invest 2001;107:255-64. [Crossref] [PubMed]
- Ben J, Jiang B, Wang D, et al. Major vault protein suppresses obesity and atherosclerosis through inhibiting IKK-NF-κB signaling mediated inflammation. Nat Commun 2019;10:1801. [Crossref] [PubMed]
- Gareus R, Kotsaki E, Xanthoulea S, et al. Endothelial cell-specific NF-kappaB inhibition protects mice from atherosclerosis. Cell Metab 2008;8:372-83. [Crossref] [PubMed]
- Jayakumar T, Hou SM, Chang CC, et al. Columbianadin Dampens In Vitro Inflammatory Actions and Inhibits Liver Injury via Inhibition of NF-κB/MAPKs: Impacts on ∙OH Radicals and HO-1 Expression. Antioxidants (Basel) 2021;10:553. [Crossref] [PubMed]
- Song D, Fang G, Mao SZ, et al. Selective inhibition of endothelial NF-κB signaling attenuates chronic intermittent hypoxia-induced atherosclerosis in mice. Atherosclerosis 2018;270:68-75. [Crossref] [PubMed]
- Xiao Q, Zhu X, Yang S, et al. LPS induces CXCL16 expression in HUVECs through the miR-146a-mediated TLR4 pathway. Int Immunopharmacol 2019;69:143-9. [Crossref] [PubMed]
- Grebe A, Hoss F, Latz E. NLRP3 Inflammasome and the IL-1 Pathway in Atherosclerosis. Circ Res 2018;122:1722-40. [Crossref] [PubMed]
- Afrasyab A, Qu P, Zhao Y, et al. Correlation of NLRP3 with severity and prognosis of coronary atherosclerosis in acute coronary syndrome patients. Heart Vessels 2016;31:1218-29. [Crossref] [PubMed]
- Shi X, Xie WL, Kong WW, et al. Expression of the NLRP3 Inflammasome in Carotid Atherosclerosis. J Stroke Cerebrovasc Dis 2015;24:2455-66. [Crossref] [PubMed]
- Zheng F, Xing S, Gong Z, et al. Silence of NLRP3 suppresses atherosclerosis and stabilizes plaques in apolipoprotein E-deficient mice. Mediators Inflamm 2014;2014:507208 [Crossref] [PubMed]
- Zhou T, Xiang DK, Li SN, et al. MicroRNA-495 Ameliorates Cardiac Microvascular Endothelial Cell Injury and Inflammatory Reaction by Suppressing the NLRP3 Inflammasome Signaling Pathway. Cell Physiol Biochem 2018;49:798-815. [Crossref] [PubMed]
- Xu D, Zhang X, Chen X, et al. Inhibition of miR-223 attenuates the NLRP3 inflammasome activation, fibrosis, and apoptosis in diabetic cardiomyopathy. Life Sci 2020;256:117980 [Crossref] [PubMed]
- Chen Z, Hu Y, Lu R, et al. MicroRNA-374a-5p inhibits neuroinflammation in neonatal hypoxic-ischemic encephalopathy via regulating NLRP3 inflammasome targeted Smad6. Life Sci 2020;252:117664 [Crossref] [PubMed]
- Abais JM, Xia M, Zhang Y, et al. Redox regulation of NLRP3 inflammasomes: ROS as trigger or effector? Antioxid Redox Signal 2015;22:1111-29. [Crossref] [PubMed]
- Qiao Y, Wang P, Qi J, et al. TLR-induced NF-κB activation regulates NLRP3 expression in murine macrophages. FEBS Lett 2012;586:1022-6. [Crossref] [PubMed]
- Deng L, Wang C, Spencer E, et al. Activation of the IkappaB kinase complex by TRAF6 requires a dimeric ubiquitin-conjugating enzyme complex and a unique polyubiquitin chain. Cell 2000;103:351-61. [Crossref] [PubMed]
- Gao W, Chang R, Sun Y, et al. MicroRNA-2187 Modulates the NF-κB and IRF3 Pathway in Teleost Fish by Targeting TRAF6. Front Immunol 2021;12:647202 [Crossref] [PubMed]
- Wang J, Wu J, Cheng Y, et al. Over-expression of microRNA-223 inhibited the proinflammatory responses in Helicobacter pylori-infection macrophages by down-regulating IRAK-1. Am J Transl Res 2016;8:615-22. [PubMed]
- Long JP, Dong LF, Chen FF, et al. miR-146a-5p targets interleukin-1 receptor-associated kinase 1 to inhibit the growth, migration, and invasion of breast cancer cells. Oncol Lett 2019;17:1573-80. [PubMed]
- Ding J, Zhang Y, Cai X, et al. Extracellular vesicles derived from M1 macrophages deliver miR-146a-5p and miR-146b-5p to suppress trophoblast migration and invasion by targeting TRAF6 in recurrent spontaneous abortion. Theranostics 2021;11:5813-30. [Crossref] [PubMed]
- Feinberg MW, Moore KJ. MicroRNA Regulation of Atherosclerosis. Circ Res 2016;118:703-20. [Crossref] [PubMed]
- Sun X, Belkin N, Feinberg MW. Endothelial microRNAs and atherosclerosis. Curr Atheroscler Rep 2013;15:372. [Crossref] [PubMed]
- Harris TA, Yamakuchi M, Ferlito M, et al. MicroRNA-126 regulates endothelial expression of vascular cell adhesion molecule 1. Proc Natl Acad Sci U S A 2008;105:1516-21. [Crossref] [PubMed]
- Rayner KJ, Sheedy FJ, Esau CC, et al. Antagonism of miR-33 in mice promotes reverse cholesterol transport and regression of atherosclerosis. J Clin Invest 2011;121:2921-31. [Crossref] [PubMed]
- Wang Z, Liu F, Wei M, et al. Chronic constriction injury-induced microRNA-146a-5p alleviates neuropathic pain through suppression of IRAK1/TRAF6 signaling pathway. J Neuroinflammation 2018;15:179. [Crossref] [PubMed]