Mechanism of action of resolvin D1 in inhibiting the progression of aortic dissection in mice
Introduction
Aortic dissection (AD) is the most common type of aortic disease, which can lead to a fatal cardiovascular emergency. It is characterized by a rapid onset and high mortality rate. Approximately 50–68% of patients with acute AD die within 2 d, and 90% die within 3 months.
Fundamental research on the pathogenesis of AD has revealed that the inflammatory response plays a key role in its development (1-4). A study (5) found that collagen cross-linking is inhibited and the expression of matrix metalloproteinases (MMPs), particularly MMP-9 and MMP-2, are significantly upregulated in mice with β-aminopropionitrile (BAPN)-induced AD. Inflammatory cell infiltration also occurs, and a large number of inflammatory factors, such as tumor necrosis factor-α (TNF-α) and interleukin-6 (IL-6), are released. TNF-α and IL-6 can activate some different inflammatory signaling pathways and promote inflammatory cell migration. And these inflammatory factors and cells increase the release of MMPs, contributes to aortic extracellular matrix degeneration and expansion in an AD setting. Resolvin D1 (RvD1) is a newly identified lipid molecule derived from ω-3 polyunsaturated fatty acids, which are important endogenous mediators that suppress inflammatory response. Docosahexaenoic acid (DHA) are metabolized to RvD by acetylated cyclooxygenase (COX)-2 and 5-lipoxygenase or through a COX-independent pathway involving cytochrome P-450 or 15-lipoxygenase. RvD1 signal via activation of GPR32 and ALX receptors. Resolvins decrease inflammatory cell migration in inflamed tissues, promote phagocytosis of apoptotic cells, and reduce the expression of inflammatory factors. It is widely found in animals and is expressed in the blood, adipose tissue, brain, lungs, and kidneys in response to inflammation (6).
Few studies have explored the role of RvD1 in AD. Whether RvD1 can further delay or inhibit the onset and development of AD by inhibiting inflammatory cell recruitment and activity remains unclear. We present the following article in accordance with the ARRIVE reporting checklist (available at https://dx.doi.org/10.21037/atm-21-3986).
Methods
Experimental animals
Wildtype C57BL/6 mice (male, 8±1 g, 3-week-old) were obtained from Beijing Huafukang Experimental Animal Technology Co., (Beijing, China). A protocol was prepared before the study without registration. The study was approved by the Animal Ethics Committee of Tianjin Chest Hospital, and the mice were housed in the animal housing facility of the Institute of Cardiovascular Diseases, Tianjin Chest Hospital, according to the guidelines of the Chinese Ethics Committee for the care and use of animal research. The mice were housed in the animal housing facility of the Institute of Cardiovascular Diseases, Tianjin Chest Hospital. The mice were fed a normal diet and administered freshly prepared BAPN (Sigma Aldrich, St. Louis, MO, USA) solution dissolved in their drinking water (0.4 g/100 mL).
AD modeling and grouping
Mice were randomly and equally divided into three groups: blank, model, and RvD1. Subsequently, each group was sacrificed at 5, 14, and 21 d, respectively, with 5 mice per group in the blank group and 14 mice per group in both the model and RvD1 groups, and their blood and tissue samples were collected for further analyses. The RvD1 and model groups were administered 0.4% BAPN solution, while the blank group was administered distilled water. In addition, according to the preliminary experiment result, the RvD1 group was administered 30 µg/kg RvD1 (Cayman, Ann Arbor, MI, USA) intraperitoneally, while the model and blank groups were administered 0.1 mL/10 g saline intraperitoneally. Daily water consumption was recorded at regular intervals. Mice were weighed every 3 d until they were sacrificed.
Echocardiography
Echocardiograms were obtained to measure the aortic diameter in mice prior to sacrifice. Each index was measured for three cardiac cycles and the mean was calculated.
Histopathological staining
After euthanasia, normal and dissected aortas were harvested from the ascending aorta to the iliac artery and were fixed in 10% buffered formalin for 24 h. Fixed, paraffin-embedded tissues were cut at 4 µm thickness. Hematoxylin and eosin (HE), Masson, and Verhoeff-Van Gieson (EVG) staining procedures were performed as previously described (7).
Enzyme-linked immunosorbent assay (ELISA) for cytokines and chemokines
Blood was drawn from the hearts of mice and centrifuged at 4 °C for 10 min at 3,000 ×g. The supernatant was harvested to determine MMP-2/9, IL-6, TNF-α, monocyte chemotactic protein-1 (MCP-1), macrophage inflammatory protein-1α (MIP-1α), MIP-1β, and keratinocyte-derived chemokine (KC) levels using an ELISA kit. Aorta tissues were homogenized and sonicated in normal saline to obtain a 2.5% homogenate. The homogenate was centrifugated at 3,000 ×g for 10 min. The supernatant was collected to measure IL-6 and TNF-α levels. Assays were performed following the manufacturer’s instructions.
Statistical analysis
The software packages SPSS 23.0b (IBM Corp., Armonk, NY, USA) and GraphPad Prism (version 5.0, GraphPad Software, San Diego, CA, USA) were used for statistical analysis. Normally distributed measurement data were expressed as mean ± standard deviation (), and comparisons between multiple groups were first subjected to one-way analysis of variance (ANOVA) followed by post hoc Newman-Keuls test. The categorical variables were compared using Pearson’s chi-square (χ2) test or Fisher’s exact test as appropriate. Differences were considered statistically significant at P<0.05.
Results
Body weight trends in different groups
The initial body weight of mice in each group ranged was 8–10 g, and the body weight of mice in all groups increased over time. The weight gain of mice in the model group decreased significantly after 15 d and was the slowest at 21 d. The blank group exhibited the most rapid weight gain. At 21 d, there was no statistically significant difference in body weight between the three groups (P>0.05) (Figure 1).
The survival rate was significantly increased in the RvD1 group
Whether mice had AD was determined by echocardiogram. After mice died, we further determined that mice had formed AD by sacrificing them. The incidence of AD in each group increased with prolonged feeding time, and the survival rate of mice with AD in both the model and RvD1 groups gradually decreased. At 5 d, no mice in the blank and RvD1 groups had developed AD, and 3 mice in model group had developed AD. At 21 d, the incidence of AD was 0 in the blank group, 71.43% in the model group, and 78.57% in the RvD1 group. The survival rate was 42.86% and 21.43% in the RvD1 and model groups, respectively, showing significant differences between the two groups (P<0.05) (Figure 2).
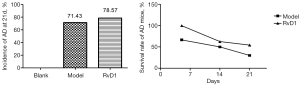
Aortic dilatation was significant in the model group
The mean diameter of the ascending aorta was measured at different time points by echocardiography in the different groups. In the blank, model, and RvD1 groups at 5 d, the mean diameter of the ascending aorta was 0.09±0.03, 0.10±0.03, and 0.10±0.04 cm, respectively. There was no significant difference between the model and RvD1 groups. At 14 d, the mean diameter of the ascending aorta of the blank, model, and RvD1 groups was 0.09±0.004, 0.11±0.003, and 0.09±0.003 cm. There was a significant difference among the three groups (P<0.05), suggesting that the aortic dilatation in model group was more severe than that in the other two groups. The mean diameter of the ascending aorta in the blank, model, and RvD1 groups at 21 d was 0.10±0.006, 0.12±0.006, and 0.11±0.005 cm, respectively. The model group had more notable aortic dilation than the blank group, and was similar to that in the RvD1 group. As time passed, the diameter of the aorta of the dissected mice in the model and RvD1 groups was significantly larger than that of the aorta of mice in the blank group, and the inner diaphragm fluctuated with blood flow (Figure 3).
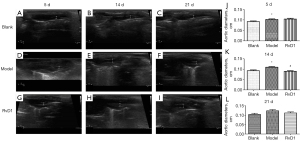
The RvD1 group exhibited lighter lesion and inflammatory cell infiltration than the model group
No AD was formed in the blank and RvD1 groups in 5 d; however, AD was present in the model group at the same time point. In 14 d, a significant thickening of the aortic middle layer was observed in the model group, a false lumen was seen in the middle layer, and erythrocytes were visible in the aortic wall. The elastic fibers were disturbed and inflammatory cell infiltration were observed. In the RvD1 group, the lesions were lighter than those in the model group, and the aortic wall structure was intact. In the 21 d model group, the destruction of the aortic wall structure and inflammatory cell infiltration were more noticeable than in the RvD1 group (Figure 4). Masson and EVG staining showed that at 14 d, the model group exhibited marked disintegration of elastic fibers in the middle layer, and blurred aortic structure was more significant than in the RvD1 group. At 21 d, a slight disintegration of elastic fibers and collagen in the aorta was observed in the RvD1 group, and the aortic structure was clear. At the same time point, aortic structure was destroyed in the model group (Figures 5,6).
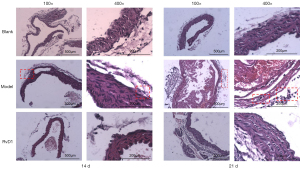
RvD1 inhibited inflammatory response in AD mice
The MMPs are a family of structurally related endopeptidases, they have the ability to degrade all components of extracellular matrix and basal lamina. Of the MMPs, MMP-2 and MMP-9 are most closely related to the occurrence and development of AD. In our study, serum MMP-2 and MMP-9 levels were significantly higher in the model group than in the blank and RvD1 groups (P<0.05), indicating that the aortic wall structure of the model group had more severely disintegrated than the other two groups. The levels of IL-6 and TNF-α in serum of the model group were significantly increased (P<0.05) compared with the other two groups. The levels of inflammatory factors in the RvD1 group, although higher than those in the blank group, were significantly decreased compared with the model group, indicating that RvD1 could effectively reduce the levels of inflammatory factors in the serum and prevent aortic wall destruction. The chemokine MCP-1 can enhance chemotaxis of monocytes and activate macrophages. The C-X-C motif chemokine ligand 1 (CXCL-1) is a kind of chemokine which is secreted by macrophages and can recruit neutrophils to aggregate to the inflammatory site. MIP-1α and MIP-1β are another two kinds of chemokines that can recruit multiple inflammatory cells to infiltration. The levels of various chemokines were significantly decreased in the RvD1 group compared with the model group, which suggested that the delayed progression of AD in mice following treatment with RvD1 may be related to the inhibition of inflammatory cell infiltration at the lesion site and reduced damage to the aortic wall by inflammatory factors (Figure 7).
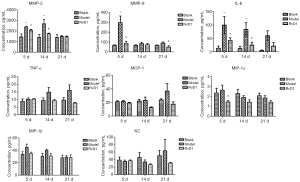
The ELISA results of AD tissue showed that at 21 d, IL-6 and TNF-α levels in the aorta were significantly decreased in the RvD1 group compared with the model group (P<0.05), indicating that RvD1 inhibited the release of inflammatory factors at the lesion site (Figure 8).

Discussion
The morbidity and mortality rates of AD are high, and complications following its emergency surgery lead to a poor prognosis. The 5-year survival rate of a conservative treatment is approximately 30%; therefore, finding new targets to delay the onset and progression of AD is an urgent challenge.
Several studies have reported infiltration by macrophages and neutrophils in aortas of patients with acute AD (8), and the onset and progression of AD may be related to local inflammation. In their study, by observing the inflammatory response in the aorta tissue of a rat model of AD, Cai et al. found that the expression of IL- 6 and interferon-γ (IFN-γ) was significantly higher in the AD model group than in the blank group, while the expression of smooth muscle protein 22-α (SM22α) in smooth muscles was significantly downregulated, indicating the crucial role of the inflammatory response in the development of AD (5). Therefore, the endogenous anti-inflammatory compound, RvD1, was used to inhibit the local inflammatory response to AD, thereby delaying the onset and progression of AD. Resolvins, as key endogenous mediators of inflammation, are widely found in animals and participate in the in vivo inflammatory response. A previous study (9) found that RvD1 could prevent oxidative stress-induced arterial injury by inhibiting the proliferation and migration of smooth muscle cells, and recruiting inflammatory cells infiltration.
In this study, BAPN solution was administered to C57BL/6 mice to establish an AD model. We found that the weight gain of the mice in each group, although different, was not significantly different (P<0.05); therefore, body weight cannot be used as an index for the incidence of AD. The incidence of AD in the model and RvD1 groups over 21 d was essentially the same, indicating that RvD1 was not effective in reducing the incidence of AD, which may have been related to the method for establishing the animal AD model used in this study. The mouse AD model was made with BAPN mainly via disruption of the cross-linking of collagen and elastin molecules within the aortic wall, while RvD1 as an anti-inflammatory mediator did not have an effect on this process. However, the mortality rate in the RvD1 group was significantly lower than that in the model group, suggesting that such anti-inflammatory mediators can delay the progression of AD and thus improve the prognosis.
As time went on, echocardiography showed that the aortic diameter of the model group was significantly larger than that of the RvD1 and blank groups. Histopathological staining also demonstrated that although the elastic fibers of the aortic wall of AD mice in the RvD1 group were disorganized and disintegrated, the lesions were less severe than those in the model group, indicating that RvD1 effectively improved the severity of local lesions. We also found that inflammatory cell infiltration at the lesion site was reduced in the RvD1 group compared with the model group, suggesting that RvD1 delayed morphological progression of AD may be associated with reducing the inflammatory reaction and inhibition of recruiting inflammatory cells. Chatterjee et al. showed (9) that RvD1 inhibits the adhesion of inflammatory cells and TNF-α expression. If antibodies against the RvD1 receptor are administered, the adhesion of monocytes and inflammatory factors to the vessel wall is increased, suggesting a vital role for RvD1 in reducing the vascular inflammatory response, similar to the results of our study.
When the levels of serum inflammatory factors were assessed, we found that those of MMP-2 and MMP-9 were significantly reduced in the RvD1 group compared with the model group (P<0.05), suggesting that RvD1 reduces the structural disintegration of the aortic wall and decreases the mortality rate of AD mice (10). Lu et al. (11) showed that the levels of IL-6, TNF-α, and CC family chemokines were significantly elevated during the first and second weeks of murine models of AD, and Fashandi et al. (12) found that the expression of cytokines, including MCP-1, MIP-1α, and MIP-1β, was significantly increased in AngII/BAPN-induced AD. In the present study, the levels of cytokines and chemokines were significantly elevated in the model group, which validated the previous findings. In contrast, the levels of inflammatory factors in the RvD1 group, although higher than those in the blank group, were significantly decreased compared with the model group (P<0.05), indicating that RvD1 could effectively reduce the levels of serum inflammatory factors (13). The levels of chemokines in the RvD1 group were lower than those in the model group, which confirmed that the delay in AD progression induced by RvD1 might be related to the inhibition of inflammatory cell infiltration at the lesion site and less damage to the aortic wall caused by inflammatory factors. The ELISA result of IL-6 and TNF-α in AD tissue also indicated that RvD1 inhibited the release of inflammatory factors at the lesion site (14). Although the serum levels of TNF-α, MCP-1, MIP-1β, and CXCL-1 did not differ significantly among the three groups at some of the same points in time, these levels showed remarkable upward or downward trends with time. Upward trends were noted in the levels of TNF-α, MCP-1, and CXCL-1, while IL-6 and MIP trended downward with time, which suggested that maybe different cytokines, chemokines, and inflammatory cells play a part at different time points (15). Further research is needed to clarify this result. The unique advantage of this study was the sacrificing of mice at different time points, which allowed us to observe aorta morphological evolution trends after AD, and detect the change tendency of cytokines and chemokines in the lesion site. We analyzed the anti-inflammatory effect of RvD1 at different time points, and revealed a part of the mechanism of inhibiting AD progression by RvD1.
In conclusion, this study applied BAPN to establish an AD mouse model and observed the pathological changes, inflammatory cell infiltration, and inflammatory factor secretion in each group of AD mice at 5, 14, and 21 d to preliminarily explore the protective role of RvD1 in AD mice. The results showed that RvD1 significantly delayed aortic dilation and disintegration and inhibited local macrophage and neutrophil infiltration in the early stages of aortic injury. Moreover, RvD1 significantly downregulated the expression of serum cytokines and chemokines, significantly reduced the secretion of IL-6 and TNF-α in aortic tissues, inhibited the inflammatory response, and improved aortic remodeling. These results also suggest the potential value of RvD1 as an endogenous anti-inflammatory mediator in clinical applications. However, the molecular mechanism of action of RvD1 in AD requires further exploration.
Acknowledgments
Funding: This work was supported by the Major Projects of Disease Prevention and Cure of Tianjin Science and Technology Commission “Clinical diagnosis and treatment research of elderly patients with aortic dissecting aneurysm in Tianjin” (18ZXDBSY00160).
Footnote
Reporting Checklist: The authors have completed the ARRIVE reporting checklist. Available at https://dx.doi.org/10.21037/atm-21-3986
Data Sharing Statement: Available at https://dx.doi.org/10.21037/atm-21-3986
Conflicts of Interest: All authors have completed the ICMJE uniform disclosure form (available at https://dx.doi.org/10.21037/atm-21-3986). The authors report that this work was supported by Major Projects of Disease Prevention and Cure of Tianjin Science and Technology Commission (18ZXDBSY00160), payments to Tianjin Chest Hospital. The authors have no other conflicts of interest to declare.
Ethical Statement: The authors are accountable for all aspects of the work in ensuring that questions related to the accuracy or integrity of any part of the work are appropriately investigated and resolved. The study was approved by the Animal Ethics Committee of Tianjin Chest Hospital, and the mice were housed in the animal housing facility of the Institute of Cardiovascular Diseases, Tianjin Chest Hospital, according to the guidelines of the Chinese Ethics Committee for the care and use of animal research.
Open Access Statement: This is an Open Access article distributed in accordance with the Creative Commons Attribution-NonCommercial-NoDerivs 4.0 International License (CC BY-NC-ND 4.0), which permits the non-commercial replication and distribution of the article with the strict proviso that no changes or edits are made and the original work is properly cited (including links to both the formal publication through the relevant DOI and the license). See: https://creativecommons.org/licenses/by-nc-nd/4.0/.
References
- Michel JB, Martin-Ventura JL, Egido J, et al. Novel aspects of the pathogenesis of aneurysms of the abdominal aorta in humans. Cardiovasc Res 2011;90:18-27. [Crossref] [PubMed]
- Fan X, Peng J, Lei L, et al. Integrated analysis of immunocyte infiltration and differential gene expression in tricuspid aortic valve-associated thoracic aortic aneurysms. Ann Transl Med 2020;8:285. [Crossref] [PubMed]
- Correction to. Heart disease and stroke statistics-2018 update: a report from the American Heart Association. Circulation 2018;137:e493. Erratum for Circulation. 2018;137:e67-492. [PubMed]
- Joyce DP, Walsh SR, Chong TT, et al. Lost in translation: do animal studies add value to the management of abdominal aortic aneurysms? J Thorac Dis 2019;11:S1277-8. [Crossref] [PubMed]
- Cai YL, Wang ZW. The expression and significance of IL-6, IFN-γ, SM22α, and MMP-2 in rat model of aortic dissection. Eur Rev Med Pharmacol Sci 2017;21:560-8. [PubMed]
- Calder PC, Waitzberg DL, Klek S, et al. Lipids in parenteral nutrition: biological aspects. JPEN J Parenter Enteral Nutr 2020;44:S21-7. [Crossref] [PubMed]
- Jia LX, Zhang WM, Zhang HJ, et al. Mechanical stretch-induced endoplasmic reticulum stress, apoptosis and inflammation contribute to thoracic aortic aneurysm and dissection. J Pathol 2015;236:373-83. [Crossref] [PubMed]
- Wu D, Choi JC, Sameri A, et al. Inflammatory cell infiltrates in acute and chronic thoracic aortic dissection. Aorta (Stamford) 2013;1:259-67. [Crossref] [PubMed]
- Chatterjee A, Komshian S, Sansbury BE, et al. Biosynthesis of proresolving lipid mediators by vascular cells and tissues. FASEB J 2017;31:3393-402. [Crossref] [PubMed]
- Spinosa M, Su G, Salmon MD, et al. Resolvin D1 decreases abdominal aortic aneurysm formation by inhibiting NETosis in a mouse model. J Vasc Surg 2018;68:93S-103S. [Crossref] [PubMed]
- Lu G, Su G, Davis JP, et al. A novel chronic advanced stage abdominal aortic aneurysm murine model. J Vasc Surg 2017;66:232-42.e4. [Crossref] [PubMed]
- Fashandi AZ, Hawkins RB, Salmon MD, et al. A novel reproducible model of aortic aneurysm rupture. Surgery 2018;163:397-403. [Crossref] [PubMed]
- Rey C, Nadjar A, Buaud B, et al. Resolvin D1 and E1 promote resolution of inflammation in microglial cells in vitro. Brain Behav Immun 2016;55:249-59. [Crossref] [PubMed]
- Ijaz T, Tilton RG, Brasier AR. Cytokine amplification and macrophage effector functions in aortic inflammation and abdominal aortic aneurysm formation. J Thorac Dis 2016;8:E746-54. [Crossref] [PubMed]
- Nienaber CA, Clough RE, Sakalihasan N, et al. Aortic dissection. Nat Rev Dis Primers 2016;2:16053. [Crossref] [PubMed]