MiR-29c inhibits the metastasis of oral squamous cell carcinoma and promotes its cell cycle arrest by targeting SERPINH1
Introduction
In 2018, there were an estimated 800,000 new cases of head and neck squamous cell carcinoma (HNSCC) and more than 450,000 deaths. Classification of HNSCC originating from the mucosa of the upper respiratory tract is made according to location: laryngeal cancer, hypopharyngeal cancer, tonsil cancer, oropharyngeal cancer, and oral cancer (1). Among them, oral cancer is a major public health problem across the world, ranking sixth among human malignancies, with a 5-year mortality rate of about 50%. Oral malignancies include cancers that occur in the mouth, lips, and pharynx, and oral squamous cell carcinoma (OSCC) accounts for over 90% of oral cancer. In 2018, OSCC comprised 43.8% of new HNSCC cases and 37.8% of deaths (2). Oral cancer is characterized by concealed onset, difficult diagnosis, rapid development, and is often accompanied by metastasis and destructive treatment (3). Therefore, it is necessary to find and develop new accurate diagnostic and effective treatment strategies for OSCC.
Serpin peptidase inhibitor clade H member 1 (SERPINH1), also known as heat shock protein 47 (HSP47), belongs to the Serpin superfamily and is functionally involved in the proper folding and secretion of collagen. The unique characteristics of SERPINH1 in regulating collagen production and its location on the cell membrane of many cancers have led to the utilization of SERPINH1 as a potential biomarker or therapeutic target for many diseases and cancers (4). The abnormal expression of SERPINH1 is closely related to a variety of cancers. Increased expression of SERPINH1 can promote cancer progression and is associated with poor survival, such as in hepatocellular carcinoma (5), lung cancer (6), renal cell carcinoma (7), and gastric cancer (8). Comprehensive screening of proteomics and transcriptome showed that SERPINH1 is strongly correlated with the poor prognosis of renal clear cell carcinoma, and it can regulate the expression of epithelial-mesenchymal transition (EMT) markers (9). Our current The Cancer Genome Atlas (TCGA) analysis showed that SERPINH1 is abnormally expressed in OSCC, but its specific regulatory mechanism is still unclear.
In this study, bioinformatics technology was used to analyze and screen the differentially expressed genes in oral squamous cell carcinoma compared with normal tongue mucosal tissue in TCGA database, and it was found for the first time that SERPINH1 may play a key role in the occurrence and development of OSCC. Through molecular biology experiments, at the cellular level, we found that silencing SERPINH1 could inhibit the growth and migration of OSCC cells, and SERPINH1 may be regulated by miR-29c. Therefore, exploring the molecular mechanism of SERPINH1 in OSCC will help us further understand the pathogenesis of OSCC. We present the following article in accordance with the MDAR reporting checklist (available at https://dx.doi.org/10.21037/atm-21-3720).
Methods
Bioinformatics
The GSE31056 microarray dataset containing gene expression data of 23 OSCC tumors and their normal marginal tissues was downloaded from the Gene Expression Omnibus (GEO) database (https://www.ncbi.nlm.nih.gov/geo/). The differentially expressed messenger RNAs (mRNAs) between normal group and tumor group in GSE31056 were obtained by the R language analysis tool GEO2R (10). At the same time, the public cancer transcriptome database UALCAN (http://ualcan.path.uab.edu/index.html) was used to examine the expression and patient survival information of SERPINH1, while TargetScan (http://www.targetscan.org/vert_72/) was used to identify the putative upstream micro RNA (miRNA) binding sites. The survival analysis and clinical stage of the target mRNA and corresponding miRNAs were analyzed by LinkedOmics database (http://www.linkedomics.org/login.php). The study was conducted in accordance with the Declaration of Helsinki (as revised in 2013).
Cell culture
Human OSCC cell lines TSCC1 (YS-X0472), CAL-27 (kl-CC101648), HSC-4 (HTX1888), SCC-4 (HS-Cs211105), SCC25 (EY-X1063) and human normal oral mucosal epithelial cells HL-047 (FS-X4623) were purchased from Beijing Boehner Culture Center (Beijing, China). All cell lines were cultured in Dulbecco’s modified Eagle medium (DMEM, Sigma-Aldrich, St. Louis, MO, USA) containing 10% fetal bovine serum (FBS, Gibco, Thermo Fisher Science, Inc., Waltham, MA, USA), 100 µg/mL streptomycin (Gibco, Thermo Fisher Science, Inc.) and 100 µg/mL penicillin (Gibco, Thermo Fisher Science, Inc.) at 37 °C in a humidified atmosphere of 5% CO2.
Cellular lentivirus infection
The lentivirus infection of Mimic NC, miR-29c mimic, sh-NC, sh-SERPINH1, ov-NC, and ov-SERPINH1 were obtained from Shanghai Genechem Company (Shanghai, China) and the SCC25 cell lines were infected according to the manufacturer’s instructions.
RNA extraction and quantitative reverse transcription polymerase chain reaction (RT-qPCR)
Total RNAs were extracted using Trizol reagent (Invitrogen, Carlsbad, CA, USA) according to the manufacturer’s instructions. Reverse transcription (RT) and quantitative RT-qPCR were performed using the PrimeScript RT reagent kit (Takara Bio., Dalian, China) and SYBR Prime Script RT PCR kit (Takara Bio., Dalian, China), respectively. RNU6-1 (U6) and glyceraldehyde 3-phosphate dehydrogenase (GAPDH) were used as internal control for miR-29c and SERPINH1, respectively. The results were calculated using the 2−ΔΔCt method. The primer sequences used for RT-qPCR are listed in Table 1.
Table 1
Target gene | Primer (5'-3') |
---|---|
|
F: 5'-GCAGTAGCACCATTTGAAATC-3' |
R: 5'-GGTCCAGTTTTTTTTTTTTTTTAACC-3' | |
|
F: 5'-CTCGCTTCGGCAGCACA-3' |
R: 5'-AACGCTTCACGAATTTGCGT-3' | |
|
F: 5'-CAGAAGTTTCTCGGGACGGG-3' |
R: 5'-GCCTGCCTTTTTCATTCTGGG-3' | |
|
F: 5'-GGAGCGAGATCCCTCCAAAAT-3' |
R: 5'-GGCTGTTGTCATACTTCTCATGG-3' |
Western blotting analysis
Proteins for immunoblotting were resolved by using sodium dodecyl sulfate-polyacrylamide gel electrophoresis (SDS-PAGE) and then transferred onto nitrocellulose membrane (Bio-Rad, Hercules, CA, USA). The membrane was blocked with 5% skimmed milk and then incubated with SERPINH1 rabbit polyclonal antibody (ab109117, Abcam, Cambridge, UK; 1:2,000 dilution; RRID: AB_10888995) and GAPDH rabbit polyclonal antibody (#2118, Cell Signaling Technology, Boston, MA, USA; 1:5,000; RRID: AB_561053) at room temperature. The membrane was incubated with horseradish peroxidase-conjugated sheep anti-rabbit IgG secondary antibody (ab6721, Abcam; 1:2,000 dilution; RRID: AB_955447) at room temperature for 2 h, and then washed 3 times with phosphate-buffered saline with Tween 20 (PBST, PBS contained 0.1% Tween 20) for 20 min. Chemiluminescent signal was visualized using a ClarityTM Western ECL Substrate (#170-5061, Bio-Rad Laboratories, Inc.,) and detected by Tanon 5200 full automatic chemiluminescence image analysis system (Tanon Science and Technology Co., Ltd., Shanghai, China).
Cell viability and proliferation assay
Cell viability was measured by 3-(4,5-dimethylthiazol-2-yl)-2,5-diphenyl tetrazolium bromide (MTT) assay. At 48 h after transfection, cells were trypsinized and seeded onto the 96-well plate (5×103 cells per well). After 72 h of incubation, 10 µL of tetrazolium blue reagent (5 mg/mL) was added to each well and incubated for 4 h at 37 °C. After the supernatant was removed, 200 µL of dimethyl sulfoxide (DMSO) was added, and the resulting colored solution was quantified by measuring the absorbance at the 490 nm using a mini tablet reader (Bio-Rad Laboratories).
Cell migration assay
Cell migration ability was determined by wound healing assay and transwell assay. For the wound healing assay, cells were seeded in 6-well plates and transfected with Mimic NC, miR-29c mimic, sh-NC and sh-SERPINH1, ov-NC, or ov-SERPINH1. A linear scratch wound was created on cell monolayers using a sterile 200 µL pipette tip, and the scratched area was photographed at ×100 magnification using a Leica DMI3000B computer-assisted microscope (Leica, Buffalo Grove, IL, USA). After scratching, images were captured at 0, 24, 48, and 72 h, and the gap area was analyzed using Image-Pro Plus v6.0 image analysis software (Media Cybernetics, Rockville, MD, USA). Transwell assay was performed using transwell chambers (Corning Inc., Corning, NY, USA) with a polycarbonate membrane. Cells (1×105 cells) were seeded onto upper transwell insert in serum-free DMEM, and the lower chamber contained 10% FBS. After incubating for about 10 h, the cells in the upper chambers were wiped off, and the cells in the lower chamber were further stained with crystal violet for 1 min at 25 °C. The stained cells were observed under a light microscope (Nikon, Melville, NY, USA, 100×) and counted in 5 randomly selected fields.
Cell cycle analysis
Briefly, the analyzed cells were collected, trypsinized, washed with PBS, and fixed with cold ethanol. The cells were subsequently stained with propidium iodide (PI, Sigma-Aldrich) for 15 min, and the percentage and proportion of cells in G0/G1, S, and G2M phase were analyzed by flow cytometry (Beckman Coulter, Brea, CA, USA).
Dual-luciferase reporter assay
For dual-luciferase reporter assay, the 3 prime untranslated region (3'-UTR) of SERPINH1 containing wild-type or mutated putative miR-29c binding site was constructed. The SCC25 cells were cultured in 24-well plates and then co-transfected with the above luciferase reporter plasmids and miR-29c mimic (or mimic NC for control group) using Lipofectamine 2000 reagent (Invitrogen). After transfection for 48 h, the cell lysates were collected and luciferase activity was detected by dual luciferase reporter gene detection system (Promega, Madison, WI, USA).
Statistical analysis
All statistical data were analyzed using SPSS 20.0 software (IBM Corp., Chicago, IL, USA). All data were presented as mean ± standard deviation (SD). The difference between groups was analyzed by t-test. Pearson chi-square (χ2) test was used to analyze the relationship between expression of miR-29c and SERPINH1. All experiments in the study were independently repeated 3 times, which were technical replicates. A P value <0.05 or <0.01 was considered statistically significant.
Results
The high expression of SERPINH1 in OSCC is related to poor prognosis
As shown in Figure 1A,1B, the gene expression in each sample was symmetrically distributed with other samples. The median-centered values in all samples were indicative, thus the samples were normalized and cross-comparable. To paraphrase, subsequent bioinformatics analysis could be continued, and the data was valuable for further screening. Through the analysis of GEO2R in the GSE31056 dataset, we found that SERPINH1 was highly expressed in tumor tissues (Figure 1C). In addition, the analysis of the UALCAN database further showed that SERPINH1 was highly expressed in OSCC patients (Figure 1D), and patients with high SERPINH1 expression were significantly associated with poor survival (Figure 1E). Combined with the clinical data of the patients in the UALCAN database, there were significant differences between individual cancer (Figure 1F), tumor grade (Figure 1G) and nodal metastasis status (Figure 1H) of OSCC, and the expression level of SERPINH1 increased with the increase of tumor stages. Furthermore, the expression of SERPINH1 in the OSCC cell line was significantly higher than that in the human normal oral mucosal epithelial cell line HL-047 (Figure 1I,1J). Taken together, SERPINH1 may play an oncogenic role in OSCC, and its high expression is associated with poor prognosis.
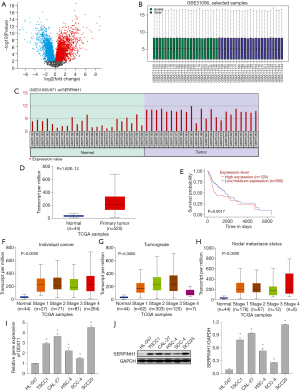
Down-regulation of SERPINH1 inhibited the proliferation and migration and promoted cell cycle arrest and apoptosis in OSCC cell line
The mRNA and protein levels of SERPINH1 in SCC25 cells transfected with sh-SERPINH1 were significantly decreased (Figure 2A,2B). The results of MTT assay showed that after inhibiting the expression of SERPINH1, the cell viability of SCC25 cells decreased significantly (Figure 2C). The results of wound-healing and transwell assays showed that the decreased expression of SERPINH1 reduced the migration ability of SCC25 cells (Figure 2D,2E). The results of 4',6-diamidino-2-phenylindole (DAPI) staining further showed that down-regulation of SERPINH1 expression reduced cell proliferation and promoted apoptosis (Figure 2F). The results of cell cycle analysis showed that the proportion of G0/G1 phase cells in the sh-SERPINH1 group was significantly lower than that in the control group, while the proportion of S phase cells was significantly higher than that in the sh-SERPINH1 group (Figure 2G). These results indicate that the reduced SERPINH1 expression inhibited the proliferation of OSCC tumor cells.
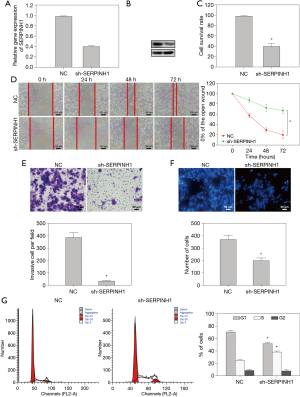
SERPINH1 as the downstream target gene of miR-29c
In order to understand the underlying regulatory mechanism of SERPINH1 in OSCC, we excavated and analyzed its upstream regulatory factors. TargetScan database was used to analyze the putative binding miRNAs on SERPINH1 and identified that miR-29c may act as the potential regulator of SERPINH1 (Figure 3A). LinkedOmics database analysis of miR-29c showed that there were differences among different clinicopathological grades (Figure 3B), different individual cancer stages (Figure 3C), and lymph node metastatic status stages (Figure 3D). In addition, LinkedOmics database survival analysis showed that patients with high miR-29c expression were associated with better survival outcome than patients with low miR-29c expression (Figure 3E). Pearson correlation analysis further showed that there was a negative correlation between miR-29c and SERPINH1 (Figure 3F). In addition, he expression of miR-29c in OSCC cell line was significantly lower than that in HL-047 cell line (Figure 3G), while the expression of SERPINH1 in these 2 cell lines was opposite. In order to validate the targeted regulation relationship between miR-29c and SERPINH1, we first examined the mRNA and protein expression of SERPINH1 in miR-29c mimic-transfected OSCC cells. The results showed that the expression of SERPINH1 was significantly down-regulated in miR-29c overexpressed cells (Figure 3H,3I). The results of dual luciferase reporter assay showed that miR-29c exerted a significant inhibitory effect on luciferase activity of the SERPINH1-WT group (Figure 3J). Taken together, these results indicate that SERPINH1 is the downstream target of miR-29c and is negatively regulated by miR-29c in OSCC.
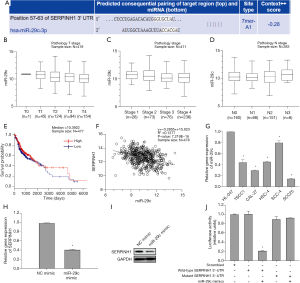
Overexpression of miR-29c inhibits the proliferation and migration and promotes cell cycle arrest and apoptosis in OSCC cells
Since miR-29c can regulate the expression of SERPINH1, we further studied the effect of miR-29c overexpression on cell function. The transfection efficiency of miR-29c is shown in Figure 4A-4C. The results of MTT assay (Figure 4D), wound healing assay (Figure 4E), transwell assay (Figure 4F), and DAPI staining (Figure 4G) showed that miR-29c overexpression significantly inhibited the proliferation and migration of OSCC cells and promoted apoptosis. Moreover, the overexpression of SERPINH1 significantly reduced the inhibitory effects of miR-29c on cell migration, invasion, and apoptosis. In addition, overexpression of miR-29c caused cell cycle arrest at S phase, which may be the cause of the inhibition of OSCC cell proliferation (Figure 4H). In summary, these results indicate that miR-29c overexpression can inhibit the cellular function of OSCC, and SERPINH1 overexpression can reverse the inhibitory effect of miR-29c.
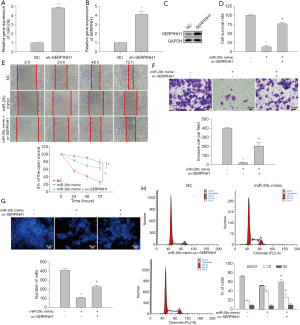
Discussion
In the present study, we found that the expression of SERPINH1 was significantly up-regulated in OSCC. Cell biology experiments showed that silencing SERPINH1 expression inhibited the proliferation and metastasis of OSCC cells. Mechanism studies further showed that miR-29c can regulate the expression of SERPINH1. In addition, bioinformatics analysis of the GSE31056 dataset revealed that the expression of SERPINH1 was significantly upregulated in OSCC tumor tissues. At the same time, this study also confirmed the increased mRNA and protein expression of SERPINH1 in OSCC cell lines.
Another bioinformatics analysis supported our finding that the expression of SERPINH1 was significantly increased in HNSCC and associated with poor overall survival (OS) rate, indicating that SERPINH1 is a potential clinical target and prognostic marker of HNSCC (11). Genome-wide analysis of alternative splicing revealed the specific expression of SERPINH1 in human hepatocellular carcinoma (12), and another study also showed that the expression of SERPINH1 is related to the development and prognosis of pancreatic ductal adenocarcinoma (13). Therefore, SERPINH1 may play an important role in OSCC tumorigenesis and development. In this study, the UALCAN database was used to examine the association between the expression of SERPINH1 and the survival and clinical staging of OSCC patients. We found that high SERPINH1 expression is significantly associated with poor survival. In addition, the expression of SERPINH1 differed significantly in different individual cancer stages, different clinicopathological grades, and different lymph node metastasis stages (4,9). Thus, we recommend using SERPINH1 as a prognostic marker for survival and early diagnosis of OSCC. In addition, we also verified the effect of SERPINH1 on cell function by silencing the expression of SERPINH1 in SCC25 cells, and found that silencing SERPINH1 not only significantly inhibited cell proliferation and migration, but also significantly increased the proportion of cells in S phase. Thus, our study further clarified the crucial function of SERPINH1 in OSCC.
It is known that miRNAs plays an important role in biological processes, such as cell migration, differentiation, and apoptosis, as well as chemoresistance of tumor cells (14). The miRNAs can directly bind to the target gene mRNA to cause translational inhibition or post-transcriptional degradation of targeted mRNA (15). In order to further explore the upstream regulation mechanism of SERPINH1, we analyzed the TargetScan and LinkedOmics databases through bioinformatics, and found that miR-29c may be putative binding site located on the 3'-UTR of SERPINH1 mRNA. A series of experimental analyses showed that miR-29c can specifically down-regulate the expression of SERPINH1 in OSCC cells, suggesting that miR-29c may act as a tumor suppressor to regulate the tumorigenicity of OSCC (13). A study by Lopes et al. showed that compared to non-cancerous tissues of healthy volunteers, miR-29c is significantly expressed in oral cancer tissues and adjacent normal tissues (16). The miR-29c-KIAA1199 axis regulates gastric cancer migration by binding with WBP11 and PTP4A3 (17). In addition, miR-29c can inhibit the expression of FBXO31 by directly binding to the 3'UTR of FBXO31, resulting in downstream activation of p38 MAPK and subsequent 5-FU chemoresistance of esophageal squamous cell carcinoma (ESCC) (18). Up-regulation of long non-coding RNA TUG1 can inhibit miR-29c and subsequently promotes the proliferation, migration, and invasion of bladder cancer cells (19). In breast cancer cells, miR-29c acts as a tumor suppressor miRNA by targeting the TIMP3/STAT1/FoxO1 signaling pathway (20). In order to further verify whether miR-29c also plays a tumor suppressor in the tumorigenesis of OSCC, we overexpressed miR-29c in OSCC cell lines, and found that miR-29c overexpression significantly inhibited the proliferation and metastasis of OSCC cells. Moreover, when SERPINH1 was further overexpressed, the tumor suppressor effect of miR-29c was reversed, which is consistent with the role of miR-29c in other tumors. It is suggested that miR-29c may play an important role in tumor treatment. In the future, the miR-29c/SERPINH1 axis may be used as a therapeutic to improve the therapeutic effect and prognosis of OSCC.
Conclusions
An important role is played by SERPINH1 in the proliferation and migration of cancer cells. This study explored the mechanism by which miR-29c regulates the expression of SERPINH1 in the malignant process of OSCC. We found that SERPINH1 can not only be used as a marker for OSCC diagnosis, but also can be used as therapeutic target to improve survival of patients with OSCC. This may become a new direction for our future research. In conclusion, this study has provided a new theoretical basis for future molecular targeted therapy of OSCC.
Acknowledgments
Funding: This study was supported by the grants of Liaoning Province Key Scientific and Technological Project (20180550035).
Footnote
Reporting Checklist: The authors have completed the MDAR reporting checklist. Available at https://dx.doi.org/10.21037/atm-21-3720
Conflicts of Interest: All authors have completed the ICMJE uniform disclosure form (available at https://dx.doi.org/10.21037/atm-21-3720). All authors have completed the ICMJE uniform disclosure form. CW reports funding support from the Liaoning Province Key Scientific and Technological Project (20180550035). The authors have no other conflicts of interest to declare.
Ethical Statement: The authors are accountable for all aspects of the work in ensuring that questions related to the accuracy or integrity of any part of the work are appropriately investigated and resolved. The study was conducted in accordance with the Declaration of Helsinki (as revised in 2013).
Open Access Statement: This is an Open Access article distributed in accordance with the Creative Commons Attribution-NonCommercial-NoDerivs 4.0 International License (CC BY-NC-ND 4.0), which permits the non-commercial replication and distribution of the article with the strict proviso that no changes or edits are made and the original work is properly cited (including links to both the formal publication through the relevant DOI and the license). See: https://creativecommons.org/licenses/by-nc-nd/4.0/.
References
- Bray F, Ferlay J, Soerjomataram I, et al. Global cancer statistics 2018: GLOBOCAN estimates of incidence and mortality worldwide for 36 cancers in 185 countries. CA Cancer J Clin 2018;68:394-424. [Crossref] [PubMed]
- Roi A, Roi CI, Negruțiu ML, et al. The Challenges of OSCC Diagnosis: Salivary Cytokines as Potential Biomarkers. J Clin Med 2020;9:2866. [Crossref] [PubMed]
- Yadav KD, Patil BA, Raheel SA, et al. Serum uric acid levels in patients with oral cancer, leukoplakia and submucous fibrosis: a cross-sectional study. Transl Cancer Res 2020;9:3084-91. [Crossref]
- Tian S, Peng P, Li J, et al. SERPINH1 regulates EMT and gastric cancer metastasis via the Wnt/β-catenin signaling pathway. Aging (Albany NY) 2020;12:3574-93. [Crossref] [PubMed]
- Wu G, Ju X, Wang Y, et al. Up-regulation of SNHG6 activates SERPINH1 expression by competitive binding to miR-139-5p to promote hepatocellular carcinoma progression. Cell Cycle 2019;18:1849-67. [Crossref] [PubMed]
- Kamikawaji K, Seki N, Watanabe M, et al. Regulation of LOXL2 and SERPINH1 by antitumor microRNA-29a in lung cancer with idiopathic pulmonary fibrosis. J Hum Genet 2016;61:985-93. [Crossref] [PubMed]
- Yamada Y, Sugawara S, Arai T, et al. Molecular pathogenesis of renal cell carcinoma: Impact of the anti-tumor miR-29 family on gene regulation. Int J Urol 2018;25:953-65. [Crossref] [PubMed]
- Kawagoe K, Wada M, Idichi T, et al. Regulation of aberrantly expressed SERPINH1 by antitumor miR-148a-5p inhibits cancer cell aggressiveness in gastric cancer. J Hum Genet 2020;65:647-56. [Crossref] [PubMed]
- Qi Y, Zhang Y, Peng Z, et al. SERPINH1 overexpression in clear cell renal cell carcinoma: association with poor clinical outcome and its potential as a novel prognostic marker. J Cell Mol Med 2018;22:1224-35. [PubMed]
- Barrett T, Wilhite SE, Ledoux P, et al. NCBI GEO: archive for functional genomics data sets--update. Nucleic Acids Res 2013;41:D991-5. [Crossref] [PubMed]
- Fan G, Tu Y, Wu N, et al. The expression profiles and prognostic values of HSPs family members in Head and neck cancer. Cancer Cell Int 2020;20:220. [Crossref] [PubMed]
- Chen H, Gao F, He M, et al. Long-Read RNA Sequencing Identifies Alternative Splice Variants in Hepatocellular Carcinoma and Tumor-Specific Isoforms. Hepatology 2019;70:1011-25. [Crossref] [PubMed]
- Zhang Y, Zhu L, Wang X. A Network-Based Approach for Identification of Subtype-Specific Master Regulators in Pancreatic Ductal Adenocarcinoma. Genes (Basel) 2020;11:155. [Crossref] [PubMed]
- Vishnubalaji R, Shaath H, Elango R, et al. Noncoding RNAs as potential mediators of resistance to cancer immunotherapy. Semin Cancer Biol 2020;65:65-79. [Crossref] [PubMed]
- Mei D, Tan WSD, Tay Y, et al. Therapeutic RNA Strategies for Chronic Obstructive Pulmonary Disease. Trends Pharmacol Sci 2020;41:475-86. [Crossref] [PubMed]
- Lopes CB, Magalhães LL, Teófilo CR, et al. Differential expression of hsa-miR-221, hsa-miR-21, hsa-miR-135b, and hsa-miR-29c suggests a field effect in oral cancer. BMC Cancer 2018;18:721. [Crossref] [PubMed]
- Wang L, Yu T, Li W, et al. The miR-29c-KIAA1199 axis regulates gastric cancer migration by binding with WBP11 and PTP4A3. Oncogene 2019;38:3134-50. [Crossref] [PubMed]
- Li B, Hong P, Zheng CC, et al. Identification of miR-29c and its Target FBXO31 as a Key Regulatory Mechanism in Esophageal Cancer Chemoresistance: Functional Validation and Clinical Significance. Theranostics 2019;9:1599-613. [Crossref] [PubMed]
- Guo P, Zhang G, Meng J, et al. Upregulation of Long Noncoding RNA TUG1 Promotes Bladder Cancer Cell Proliferation, Migration, and Invasion by Inhibiting miR-29c. Oncol Res 2018;26:1083-91. [Crossref] [PubMed]
- Li W, Yi J, Zheng X, et al. miR-29c plays a suppressive role in breast cancer by targeting the TIMP3/STAT1/FOXO1 pathway. Clin Epigenetics 2018;10:64. [Crossref] [PubMed]
(English Language Editor: J. Jones)