Chemically enhanced radiotherapy: visions for the future
Introduction
Radiotherapy (RT) is an important aspect of cancer care, with estimates suggesting that it is currently responsible for 40% of all cancer cures (1). Ever since RT has been used for treating cancer, there has been a constant quest to improve effectiveness while reducing toxicity. The earliest methods included experimentation with radiation time-dose-fractionation based on radiobiological modelling. Then, understandings about predictors of radiation response, such as anaemia and tumour hypoxia generated a quest for combating hypoxia by addition of experimental oxymimetic radiosensitizers (such as misonidazole & nimorazole) (2). That era also ushered the combination of chemotherapy with RT, earlier with agents such as hydroxyurea and methotrexate, and later with 5-FU analogs and platinum agents. The discovery of monoclonal antibodies and small molecule tyrosine kinase inhibitors against various growth factor receptors and angiogenic targets had encouraged the combination of RT with targeted therapy. The combination of hormonal therapy prior to RT in prostate cancer, the use of neoadjuvant chemotherapy prior to RT in head and neck squamous cancers have been other recent attempts intended at improving RT outcomes (3-5).
Despite the modern advances in RT such as the adoption of conformal and intensity modulated treatments, cancer patients in significant numbers continue to fall prey to the formidable malady. Thus it would be realistic to admit at present, that the current accomplishments are far from adequate, if not trivial. But there is hope for a better future. Newer understandings on cancer biology and newer perspectives in cancer therapies have opened up scope for dramatic improvements in outcomes for the future.
Recent understandings about the interactions between RT, chemotherapy and the host immune system has encouraged the development of strategies to utilize these to unlock new opportunities to improve RT outcomes. The advent of nanoscale medicine has allowed better tumour targeting of chemotherapeutic agents. The fact that RT and nanoscale medicines augment the efficacy of each other makes their concurrent use an excellent proposition worth exploration. The use of nanoscale particles with high atomic number elements as radiosensitizers is another exciting prospect worthy of investigation, as this approach can be expected to provide effects similar to heavy ion therapy, all while using a conventional photon beam. Newer perspectives in delivery of chemotherapy have explored the rather unconventional form of chemotherapy such as metronomic chemotherapy. These used with, or after RT may help enhance outcomes while sparing the patient from the toxicities of conventional chemotherapy. Also, the discovery of hyperradiosensitivity at low doses of radiation has encouraged the use of low dose RT as a chemopotentiator.
This editorial review touches upon the most promising prospects which may revolutionize radiotherapeutic treatment of cancer patients. Less emphasis has been placed on on-going trials involving newer conventional chemotherapeutics and existing molecular targeted therapies, and instead, focus has been placed upon novel ideas and technologies that are likely to be available in the foreseeable future.
Immunology, immunological interactions and prospects
While some components of the immune system, such as the cytotoxic T lymphocytes and NK cells strive hard to eliminate tumours, cancer cells enjoy the protective effect of certain immune suppressive cells and suppressive cytokines. Recently it has been understood that a variety of tumour protective immune factors exist, such as T-reg (CD4+CD25+ regulatory T-cells), MDSC (myeloid derived suppressive cells), certain cytokines (IL-10, TGF-beta) and regulatory tumor associated macrophages (TAMs) (6). It has been demonstrated that chemotherapy and RT alter tumours’ immune tolerance and that this could indeed be an important, albeit less recognized mechanism of action of these modalities.
Of late, it has been established that while maximally tolerated dose (MTD) chemotherapy depletes all immune cells, low dose chemotherapy selectively depletes T-reg cells and hence enhance antitumor immune response. For example, low dose cyclophosphamide reduces suppressive cells selectively without depleting cytotoxic T cells; but at higher doses, cyclophosphamide loses this specificity (7). Other agents at lower doses such as paclitaxel and 5-FU are known to cause MDSC apoptosis. The same is discussed later under the heading dedicated to metronomic chemotherapy.
Recent research has focussed upon the function of CTLA-4 and PD-1 receptors. These receptors present on cytotoxic T cells act in a suppressive manner so as to prevent autoimmunity. However, this inhibition also allows cancer cells to survive killing by these cytotoxic T-cells. Monoclonal antibodies against CTLA-4 (ipilimumab) and PD-1 (nivolumab) have demonstrated excellent results in melanoma and lung carcinoma. RT and anti-CTLA-4 and PD-1 inhibitors are likely to be synergistic. An interesting case report described a patient who had progressive disease despite initial immunotherapy with ipilimumab and a sudden dramatic systemic response after localized RT (8).
Prospects with nanomedicine
Nanoscale technology aims to enhance drug targeting, improve biodistribution, overcome resistance mechanisms and reduce toxicity of therapeutic molecules. Various nanomedicines utilizing forms of liposomes, polymers, micelles, dendrimers and others are observed to passively accumulate in tumours owing to their vasculature which is leakier due to wider fenestrations. This enhanced accumulation at tumour sites is called ‘enhanced permeability and retention’ (EPR) effect (9). Though obstacles to the EPR effect do exist, most significantly in the form of reticuloendothelial system (RES) capture, this can be reduced by the use of PEGylation which by producing a hydrated barrier causes hindrance to the attachment of phagocytes. The EPR effect along with PEGylation can increase tumour drug concentration by 10-100 times in comparison to that with the use of free drugs (10). There is immense potential for the use of nanoscale technology in cancer treatment (Table 1). First of all, the availability of agents such as liposomal doxorubicin and nano-albumin bound (nab-) paclitaxel has already enhanced efficacy and safety in comparison to the more traditional forms of doxorubicin and paclitaxel. Thus, the use nano-particle bound chemotherapy in place of conventional free forms of chemotherapy will render the delivery of concurrent chemoradiotherapy much more efficacious and tolerable (11).
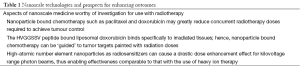
Full table
In a potential ‘eureka moment’ for oncology, it was observed that the use of poly-L glutamic acid bound paclitaxel as a radiosensitizer could reduce the TCD50 dose in a preclinical model from 53.9 Gray (Gy) to just 7.9 Gy (12). Another interesting observation has been that the tumour targeting of liposomal doxorubicin could be enhanced by the use of a peptide (HVGGSSV) which would bind selectively to irradiated tumours. Thus, this selective binding suggests that irradiation can be utilized to guide drug delivery to tumours (13).
The use of high atomic number (Z) nanoparticles as radiosensitizers is another extremely attractive approach on the horizon. The high Z atoms interact in a very different manner to ionizing radiation in comparison to low Z atoms. Since biological tissues are mostly made up of low Z atoms, the introduction of high Z nanoparticles into tumours can dramatically intensify response to RT. High Z atoms during photoelectric effect undergo inner shell ionization wherein one of the deeply bound electrons is ejected. This results in a highly unstable atomic system which stabilizes by the emission of low energy photons (fluorescence) and augur electrons. Several augur electron emissions can occur from single inner shell ionization and these augur electrons have a range of 10-100 nm and hence deposit energy very locally. Thus, a very high energy deposition which can be comparable to heavy ion therapy can be achieved with the use of a high Z nanoparticle radiosensitizer (14,15).
The most commonly studied high Z nanoparticle radiosensitizers in preclinical models include gold, iron oxide and hafnium oxide nanoparticles. An in-silico simulation showed that the use of gold nanoparticles as radiosensitizer could lead to higher dose enhancement with kilovoltage range ionizing radiation. For example, while the dose-enhancement with 2 megavolt photons was 53%, the dose enhancement with kilovoltage range photons was as high as 560% (16). While nanoparticles are known to enhance RT, the favour is returned by RT in that it enhances nanoparticle accumulation into tumours. In a mouse breast tumour model, it was observed that iron oxide nanoparticle accumulation was doubled after a single 15 Gy dose (17).
Metronomic chemotherapy to enhance RT
Metronomic chemotherapy is the chronic administration of chemotherapy at low doses which are minimally toxic, in a schedule of administration without prolonged drug-free breaks. Commonly used agents include low dose versions of conventional chemotherapy agents such as cyclophosphamide, methotrexate, capecitabine, etoposide, etc. In addition, a few non-cancer drugs such as celecoxib, metformin, valproate and such are being used (termed ‘drug-repositioning’, wherein drugs approved for non-oncological indications are re-positioned for oncological use (18).
In contrast to conventional MTD chemotherapy, the new approach of metronomic chemotherapy is very less toxic inherently by its design. Many subtle properties of chemotherapeutic agents could possibly have been masked by the MTD approach, but are now being unravelled in the metronomic approach (19). While MTD chemotherapy solely aims towards killing of malignant cells, the metronomic approach owes its efficacy to numerous other effects. Metronomic chemotherapy has anti-angiogenic effects and this has been demonstrated experimentally in that it was able to reduce angiogenic factors such as thrombospondin-1 (20). Another study in patients undergoing thoracic irradiation for lung cancer revealed that the addition of metronomic chemotherapy caused a marked reduction of VEGF (21). In addition to its anti-angiogenic effects, metronomic chemotherapy also has immunoregulatory functions, wherein the elimination of immunosuppressive cells (such as T-reg & MDSC) and the increased MHC-1 molecule expression, increased dendritic cell maturation are all known to enhance anti-tumour immune response (22).
Metronomic chemotherapy has already proven beneficial in often difficult situations involving patients who were heavily treated with conventional regimens (23,24). At present there is a paucity of clinical trials combining concurrent metronomic chemotherapy with RT. If not in every patient, this approach could help improve outcomes at least in patients who are unlikely to tolerate use of conventional concurrent chemotherapy (Table 2). Lastly, it is very much feasible that the use of metronomic chemotherapy adjuvant after a course of standard treatment may help reduce distant recurrences. A study utilizing metronomic tegafur-uracil observed a very significant reduction in distant failures among nasopharyngeal carcinoma patients who had persisting plasma EBV DNA levels after completion of definitive chemoradiotherapy (25).
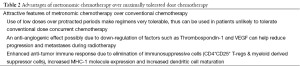
Full table
Prospects with antiangiogenic therapy
The use of RT can unintentionally enhance the process of angiogenesis by up-regulating factors such as VEGF in tumor cells, VEGFR in endothelial cells and αVβ3 integrin in tumor endothelial cells. Thus, the quest for combining antiangiogenic use with RT is very much rational (26). Recently, the results of the RTOG 0615 study regarding the addition of bevacizumab (monoclonal antibody targeting VEGF) with chemoradiation for nasopharyngeal carcinoma was made available. The phase II study demonstrated feasibility for adding bevacizumab to standard chemoradiation in treating nasopharyngeal carcinoma, and suggested that there may be a slight benefit in the form of delaying progression of subclinical distant disease (27). However the overall results with bevacizumab so far have been far from satisfactory, and this is despite the great zeal that existed for integrating bevacizumab into anti-cancer regimens a decade ago (28).
The toxicity and questionable efficacy of bevacizumab has now led to the quest for newer anti-angiogenic agents. The antiangiogenic effects of small molecule tyrosine kinase inhibitors such as sunitinib, sorafenib and pazopanib has roused interest, but their efficacy is yet to be established, especially in conjunction with RT.
The discovery of RGD (arginine-glycine-glutamine) as an αVβ3 integrin antagonist has opened another window of opportunity. Since RT in itself up-regulates αVβ3 integrin in tumor endothelial cells, despite tumour cell kill RT can unintentionally promote angiogenesis mediated progression/metastases in tumours such as glioma. The development of integrin antagonists such as the RGD peptidomimetic agent S247 and the cyclic RGD pentapeptide cilengitide may help enhance RT outcomes by antagonizing RT induced αVβ3 integrin upregulation (29). However, as with all anti-angiogenic agents, optimism must be guarded until when efficacy can be proven beyond doubt.
Prospects with newer conventional chemotherapy and targeted therapy agents
The expected increments in outcomes with the combinations of RT with conventionally delivered ‘newer’ chemotherapy agents (e.g., docetaxel, pemetrexed, gemcitabine) and molecular targeted therapies (e.g., cetuximab, nimotuzumab, erlotinib) are small, even if statistically significant. This is in contrast to the prospects to dramatic improvements potentially feasible with novel agents and newer methodology available at the horizon, for example with the use of gold nanoparticles as radiosensitizers, or with the use of immunological methods of enhancing RT. This editorial review does not consider these currently existing agents as ‘novel’ agents, and thus, little emphasis is placed upon these.
Utilizing low dose radiation as a chemopotentiator
Conventionally, RT is delivered with dose-fraction sizes of 1.8–3 Gy per fraction. Lower doses per fraction are generally avoided given the undue prolongation of overall treatment time, as well as the prediction of lesser cell kill at lower fraction sizes. It was, however, observed that cell killing at doses <1 Gy is greater than that predicted by the linear-quadratic model, hence the nomenclature ‘low dose hyperradiosensitivity’. It is postulated that this phenomenon is present below the threshold dose that would be required to initiate cellular radiation response mechanisms. In particular, a radiation inducible ATM gene dependent G2 phase checkpoint was found to have a threshold activation dose <0.4 Gy. Thus at such low doses, hyperradiosensitivity occurs due to failure of cell cycle arrest of these radiation-damaged G2-phase cells (30-32).
Despite the enhanced cell killing with low-dose fractionated radiotherapy (LDFRT), it would not be practical to utilize LDFRT alone as a treatment regimen, since the entire course of RT would be likely to be long enough to cause accelerated tumour cell repopulation to negate any cell killing. Hence, an innovative approach has been experimented, that involving the use of LDFRT to enhance chemotherapeutic outcomes.
Various studies have utilized LDFRT to potentiate various regimens of chemotherapy in difficult clinical situations. For example, LDFRT was used to potentiate chemotherapy with pemetrexed in recurrent lung carcinoma, and a dramatic enhancement in response rates was observed (33). LDFRT when used with gemcitabine in pancreatic carcinoma was associated with promising response rates (34). LDFRT was also observed to enhance response rates in head and neck carcinoma when used with neoadjuvant chemotherapy with paclitaxel and carboplatin (35). Among patients with breast carcinoma, when LDFRT was used with neoadjuvant chemotherapy, there was very good tolerability, and a good pathological complete response (pCR) rate. Among those patients who did not attain pCR, a fibrotic reaction was found to encase the residual tumour, which could potentially be inhibitory for residual cells to proliferate or metastasize (36).
Despite very good outcomes without any noticeable additional toxicity with the use of LDFRT as a chemopotentiator, it is rather unfortunate that very few clinical trials have focused upon its prospects. It is hoped that future research will help us refine the logic and the technicalities associated with the use of LDFRT as a chemopotentiator.
Summary and conclusions
While the prospects of promising modalities and methods to improve radiotherapeutic outcomes have been touched upon in this review, it must be said that there could be, and there will be many more innovations which could brighten up prospects of better outcomes for cancer patients (Table 3). The development very precise technologies of RT delivery, combined with the discovery of newer radiopharmaceuticals for positron emission tomography for functional imaging may enable extremely intelligent and biologically adaptive treatment delivery. The discovery of newer radioprotectors is largely welcome too, given that the existing radioprotector namely amifostine holds limited efficacy in limited sites. Also, advances in epigenetics will inevitably be attempted to augment RT outcomes.
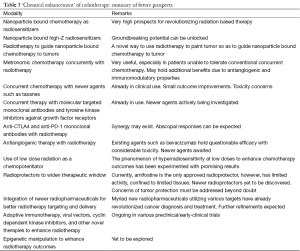
Full table
Going by existing trends, it is unfortunate that very little interest and funding is dedicated to studies using chemotherapy to enhance RT (37). It must also be remarked that despite extremely good prospects with technologies such as high-Z radiosensitizers, and despite very promising results with initial trials with low dose hyperradiosensitivity for chemopotentiation, there has been very few trials to continue the progress (this is in sharp contrast to the amount of funding and emphasis received by futile trials involving antiangiogenic therapies). It can only be hoped that in the future, trials dedicated to chemically enhance RT will receive their due share of funding.
While clinical research follows a strict rational approach for conceptual development, occasional ideas may be found through ‘out of the box, lateral thinking’, too. Too much of adherence to current standards may be harmful, as potential discoveries are lost due to our staunch obsession with the present, and neglect for the future. All said and done, progress, big or small can only be expected to be achieved through efforts, and with an open mind. While the world remains obsessed with the practise of ‘evidence based medicine’, it can only be hoped that new innovations do get their legitimate share of optimism and emphasis.
Acknowledgements
The authors thank Dr. Irappa Madabhavi, Medical Oncologist, for his valuable advice in preparing the manuscript.
Footnote
Conflicts of Interest: The authors have no conflicts of interest to declare.
References
- Radiotherapy for Cancer. Summary. Acta Oncol 1996;35 Suppl 6:9-23. [PubMed]
- Dische S, Saunders MI, Anderson P, et al. Clinical experience with nitroimidazoles as radiosensitizers. Int J Radiat Oncol Biol Phys 1982;8:335-8. [PubMed]
- Zhang L, Jiang N, Shi Y, et al. Induction chemotherapy with concurrent chemoradiotherapy versus concurrent chemoradiotherapy for locally advanced squamous cell carcinoma of head and neck: a meta-analysis. Sci Rep 2015;5:10798. [PubMed]
- Vokes EE, Haraf DJ, Panje WR, et al. Hydroxyurea with concomitant radiotherapy for locally advanced head and neck cancer. Semin Oncol 1992;19:53-8. [PubMed]
- Hu MH, Wang LW, Lu HJ, et al. Cisplatin-based chemotherapy versus cetuximab in concurrent chemoradiotherapy for locally advanced head and neck cancer treatment. Biomed Res Int 2014;2014:904341.
- Zheng Y, Dou Y, Duan L, et al. Using chemo-drugs or irradiation to break immune tolerance and facilitate immunotherapy in solid cancer. Cell Immunol 2015;294:54-9. [PubMed]
- Ghiringhelli F, Menard C, Puig PE, et al. Metronomic cyclophosphamide regimen selectively depletes CD4+CD25+ regulatory T cells and restores T and NK effector functions in end stage cancer patients. Cancer Immunol Immunother 2007;56:641-8. [PubMed]
- Postow MA, Callahan MK, Barker CA, et al. Immunologic correlates of the abscopal effect in a patient with melanoma. N Engl J Med 2012;366:925-31. [PubMed]
- Wong AD, Ye M, Ulmschneider MB, et al. Quantitative Analysis of the Enhanced Permeation and Retention (EPR) Effect. PLoS One 2015;10:e0123461. [PubMed]
- Davies Cde L, Lundstrøm LM, Frengen J, et al. Radiation improves the distribution and uptake of liposomal doxorubicin (caelyx) in human osteosarcoma xenografts. Cancer Res 2004;64:547-53. [PubMed]
- Lammers T, Subr V, Peschke P, et al. Image-guided and passively tumour-targeted polymeric nanomedicines for radiochemotherapy. Br J Cancer 2008;99:900-10. [PubMed]
- Milas L, Mason KA, Hunter N, et al. Poly(L-glutamic acid)-paclitaxel conjugate is a potent enhancer of tumor radiocurability. Int J Radiat Oncol Biol Phys 2003;55:707-12. [PubMed]
- Lowery A, Onishko H, Hallahan DE, et al. Tumor-targeted delivery of liposome-encapsulated doxorubicin by use of a peptide that selectively binds to irradiated tumors. J Control Release 2011;150:117-24. [PubMed]
- Coulter JA, Hyland WB, Nicol J, et al. Radiosensitising nanoparticles as novel cancer therapeutics--pipe dream or realistic prospect? Clin Oncol (R Coll Radiol) 2013;25:593-603. [PubMed]
- Maggiorella L, Barouch G, Devaux C, et al. Nanoscale radiotherapy with hafnium oxide nanoparticles. Future Oncol 2012;8:1167-81. [PubMed]
- Hainfeld JF, Dilmanian FA, Slatkin DN, et al. Radiotherapy enhancement with gold nanoparticles. J Pharm Pharmacol 2008;60:977-85. [PubMed]
- Giustini AJ, Petryk AA, Hoopes PJ. Ionizing radiation increases systemic nanoparticle tumor accumulation. Nanomedicine 2012;8:818-21. [PubMed]
- André N, Banavali S, Snihur Y, et al. Has the time come for metronomics in low-income and middle-income countries? Lancet Oncol 2013;14:e239-48. [PubMed]
- Miller KD, Sweeney CJ, Sledge GW Jr. Redefining the target: chemotherapeutics as antiangiogenics. J Clin Oncol 2001;19:1195-206. [PubMed]
- Montagna E, Cancello G, Dellapasqua S, et al. Metronomic therapy and breast cancer: a systematic review. Cancer Treat Rev 2014;40:942-50. [PubMed]
- Chen Y, Hyrien O, Okunieff P, et al. O-039 Impact of metronomic chemotherapy schedule on circulating VEGF and bFGF during concurrent thoracic radiotherapy. Lung Cancer 2005;49:S16.
- Hao YB, Yi SY, Ruan J, et al. New insights into metronomic chemotherapy-induced immunoregulation. Cancer Lett 2014;354:220-6. [PubMed]
- Barroso-Sousa R, da Fonseca LG, Souza KT, et al. Metronomic oral cyclophosphamide plus prednisone in docetaxel-pretreated patients with metastatic castration-resistant prostate cancer. Med Oncol 2015;32:443. [PubMed]
- Revannasiddaiah S, Madabhavi I, Bodh A, et al. Metronomic chemotherapy in anaplastic thyroid carcinoma: a potentially feasible alternative to therapeutic nihilism. Indian J Palliat Care 2015;21:245-9. [PubMed]
- Twu CW, Wang WY, Chen CC, et al. Metronomic adjuvant chemotherapy improves treatment outcome in nasopharyngeal carcinoma patients with postradiation persistently detectable plasma Epstein-Barr virus deoxyribonucleic acid. Int J Radiat Oncol Biol Phys 2014;89:21-9. [PubMed]
- Abdollahi A, Griggs DW, Zieher H, et al. Inhibition of alpha(v)beta3 integrin survival signaling enhances antiangiogenic and antitumor effects of radiotherapy. Clin Cancer Res 2005;11:6270-9. [PubMed]
- Lee NY, Zhang Q, Pfister DG, et al. Addition of bevacizumab to standard chemoradiation for locoregionally advanced nasopharyngeal carcinoma (RTOG 0615): a phase 2 multi-institutional trial. Lancet Oncol 2012;13:172-80. [PubMed]
- Citrin D, Ménard C, Camphausen K. Combining radiotherapy and angiogenesis inhibitors: clinical trial design. Int J Radiat Oncol Biol Phys 2006;64:15-25. [PubMed]
- Garcia-Barros M, Paris F, Cordon-Cardo C, et al. Tumor response to radiotherapy regulated by endothelial cell apoptosis. Science 2003;300:1155-9. [PubMed]
- Joiner MC, Marples B, Lambin P, et al. Low-dose hypersensitivity: current status and possible mechanisms. Int J Radiat Oncol Biol Phys 2001;49:379-89. [PubMed]
- Marples B, Wouters BG, Collis SJ, et al. Low-dose hyper-radiosensitivity: a consequence of ineffective cell cycle arrest of radiation-damaged G2-phase cells. Radiat Res 2004;161:247-55. [PubMed]
- Xu B, Kim ST, Lim DS, et al. Two molecularly distinct G(2)/M checkpoints are induced by ionizing irradiation. Mol Cell Biol 2002;22:1049-59. [PubMed]
- Mantini G, Valentini V, Meduri B, et al. Low-dose radiotherapy as a chemo-potentiator of a chemotherapy regimen with pemetrexed for recurrent non-small-cell lung cancer: a prospective phase II study. Radiother Oncol 2012;105:161-6. [PubMed]
- Regine WF, Hanna N, Garofalo MC, et al. Low-dose radiotherapy as a chemopotentiator of gemcitabine in tumors of the pancreas or small bowel: a phase I study exploring a new treatment paradigm. Int J Radiat Oncol Biol Phys 2007;68:172-7. [PubMed]
- Arnold SM, Regine WF, Ahmed MM, et al. Low-dose fractionated radiation as a chemopotentiator of neoadjuvant paclitaxel and carboplatin for locally advanced squamous cell carcinoma of the head and neck: results of a new treatment paradigm. Int J Radiat Oncol Biol Phys 2004;58:1411-7. [PubMed]
- Nardone L, Diletto B, De Santis MC, et al. Primary systemic treatment and concomitant low dose radiotherapy for breast cancer: final results of a prospective phase II study. Breast 2014;23:597-602. [PubMed]
- Ataman OU, Sambrook SJ, Wilks C, et al. The clinical development of molecularly targeted agents in combination with radiation therapy: a pharmaceutical perspective. Int J Radiat Oncol Biol Phys 2012;84:e447-54. [PubMed]