USP41 promotes breast cancer via regulating RACK1
Introduction
Breast cancer (BC) is the most common cancer diagnosed among women and is the second leading cause of cancer death (1). In 2019, approximately 268,600 new cases of invasive BC and 48,100 cases of ductal carcinoma in situ (DCIS) were diagnosed among US women, and 41,760 women died from this disease (2). Similarly, in China, the incidence rate of BC has increased obviously both in urban and rural areas (3); however, the 5-year survival rate of BC in China is 73%, significantly lower than that in developed countries (4). With the development of precision medicine and targeted therapeutics, BC treatment has also begun to evolve toward a more individualized, precise, and targeted approach (5). As a highly heterogeneous disease, BC involves a complex etiology, including genetic alterations, reproductive factors, and environmental factor (6). Hence, it is of great significance to gain a better understanding of the mechanisms underlying BC and to develop novel and effective therapeutic strategies (7).
Ubiquitination can regulate a variety of complex cellular processes and may lead to the activation or deactivation of tumorigenic pathways in cancer (8,9). Deubiquitinating enzymes (DUBs), reversing the ubiquitination process by removal of ubiquitin, have emerged as promising drug targets for cancer therapy. The DUBs can be divided into 6 subclasses: ubiquitin C-terminal hydrolases (UCHs), ubiquitin-specific proteases (USPs or UBPs), ovarian tumor proteases (OTUs), Machado-Joseph disease proteases (MJDs), JAB1/MPN/Mov34 metalloenzymes (JAMMs), and monocyte chemotactic protein-induced deubiquitin family (MCPIPs). Among the 6 structurally different DUB families, the largest group comprises the ubiquitin-specific proteases (USPs) that belong to the cysteine protease family (10,11). Many studies have indicated that USPs regulate tumor formation by modulating the proliferation and death of cancer cells. In 2019, Zhang et al. showed that USP22 plays critical roles in the malignancy and progression of non-small cell lung cancer (12). In 2020, ubiquitin-specific protease 41 (USP14) was shown to promote prostate cancer progression through deubiquitinating the transcriptional factor ATF2 (13). Lai et al. found that low expression of USP4 was associated with poor survival among lung cancer patients (14). Yun et al. considered USP21 an attractive therapeutic target in metastatic colorectal cancer with high Fra-1 expression (15). In 2004, USP41 was first identified in human prostate, brain, lungs, aorta, and kidneys (16). In 2021, Ji et al. reported the role of USP41 in promoting lung cancer cell proliferation and migration (17). Until now, no data has been reported about the function of USP41 in cancer development, including BC (18). Moreover, the mechanism of USP41 in cancer progression remains unclear.
In this study, we reported for the first time that USP41 was upregulated in BC and interpreted its possible signaling for BC as oncogene. We found that USP41 upregulation enhanced the growth, proliferation, and invasion of BC cells. The potential mechanism of USP41 in BC was also explored, and it was shown that receptor for activated C kinase 1 (RACK1)-related signaling may participate in this progression. Therapy targeting USP41 and RACK1 may provide a novel and promising approach to BC treatment.
We present the following article in accordance with the MDAR reporting checklist (available at https://dx.doi.org/10.21037/atm-21-4921).
Methods
Clinical samples
We obtained BC specimens and paired adjacent normal breast tissue specimens from 10 patients with primary BC. All participants were female aged 45.7±12.50 and received no therapy before sample collection. The study was approved by an independent ethics committee of Fourth Military Medical University (KY20213157-1). The study was undertaken in accordance with the Good Clinical Practice guidelines and the Declaration of Helsinki (as revised in 2013). All participants were asked to provide written informed consent before enrollment. All tissues were collected immediately upon resection and transported in liquid nitrogen.
Cells, antibodies, and reagents
Human BC cell lines (MDA-MB-231, MCF-7) were obtained from American Type Culture Collection (ATCC; Rockville, MD, USA). The MDA-MB-231 cells were cultured in Dulbecco’s modified Eagle medium (DMEM) supplemented with 10% fetal bovine serum (FBS). The MCF-7 cells were grown in DMEM supplemented with 10% FBS. All the cells were cultured in a humidified atmosphere with 5% CO2 at 37 °C. RACK1 (Proteintech, USA, 1:1,000), USP41 (Invitrogen, USA, 1:1,000), β-actin (Servicebio, China, 1:1,000), goat anti-mouse IgG (1:5,000, Sigma-Aldrich, St. Louis, MO, USA) and GAPDH (Proteintech, USA, 1:1,000) were used for western blot.
The Cancer Genome Atlas (TCGA) database analyses
We used TCGA database (http://tcga-data.nci.nih.gov/tcga/) to investigate the expression of USP41 in BC. The BC patients were enrolled regardless of hormonal status and human epidermal growth factor receptor 2 (HER2) status. There were 1,104 cancer samples and 113 normal samples included. These participants had received chemotherapy, including adjuvant and neoadjuvant chemotherapy, but the specific regimen was unknown. The participants were segregated into “low” and “high” level of USP41 messenger RNA (mRNA) by median and we analyzed its relationship with survival.
Knockdown and overexpression assay
Knockdown assay was performed by transfecting the cells with siRNAs. siRNAs were obtained from HIPPOBIO (Huzhou, China). Lipofectamine® RNAiMAX Reagent (Invitrogen) was used to transfect the siRNAs in indicated cells, according to the manufacturers’ protocols. The sequences of siRNAs were as follow. siCtrl, 5'-UUCUCCGAACGUGUCACGU-3'; siUSP41#1, 5'-GGCUCAUCAGUGUCAGUACGU-3'; siUSP41#2, 5'-GCUCACCCUCCGACUUUCUUU-3'; siRACK1#1, 5'-CCAUGUUAUGGGAUCUCAA-3'; siRACK1#2, 5'-CCAACAGCAGCAACCCUAU-3'.
Overexpression assay was performed by transfecting the cells with pCDH vectors. Negative controls cells were transfected with empty pCDH vectors. Overexpressed cells were transfected with pCDH vectors containing the coding sequence of indicated genes.
Cell proliferation analysis
Cell Counting Kit-8 (CCK-8) assay was performed to measure proliferation of BC cells according to manufacturer’s instructions. Briefly, 6,000 cells/well were seeded in 96-well plates in medium containing 10% FBS and incubated under 37 °C, 5% CO2. After treatment, 10 µL CCK-8 reagent was added and incubated for 1 h, the absorbance under 450 nm was measured with a microplate reader. The same experiments were repeated after a defined incubation period.
Colony formation assay
The different cells were seeded in 6 cm dishes at a density of 300 cells/dish. Following incubation for 2 weeks in a humidified incubator at 37 °C in an atmosphere of 95% air and 5% CO2, the cells were washed with phosphate-buffered saline (PBS), and colonies were fixed with methanol for 10 min and stained with 0.5% crystal violet for 15 min. The number of colonies was counted under a microscope (CKX53; Olympus, Tokyo, Japan). All experiments were performed in triplicate dishes in 3 independent experiments.
Transwell assay
Transwell migration assay was performed using Transwell inserts. In serum-free medium, 8 ×104 cells were seeded into the upper chamber of the insert and the bottom of the chamber contained the DMEM with 10% FBS. After 36 h incubation, the cells were fixed with methanol and stained with Giemsa. Then cells on the top surface of the membrane were wiped off, and cells on the lower surface were examined under an inverted light microscope. The number of migrated or invaded cells was quantified by counting the number of cells from 10 random fields at ×100 magnification.
Apoptosis assays
Additionally, cellular apoptosis was determined by flow cytometry (FAC). Cell lines cultured in DMEM supplemented with 10% FCS were seeded in 96-well plates (2×104 cells/well). After treatment with USP41 overexpression or knockdown, cellular apoptosis was determined by FAC after a stain with Annexin v-FITC or propidium iodide (PI) or both for 15 min in the dark at room temperature following the instructions of the Annexin v-FITC apoptosis detection kit.
Cell cycle analysis
Cell cycle distribution was analyzed using flow cytometric analysis. Briefly, after treatment with USP41 overexpression or knockdown, cells were harvested and fixed with precooled 75% (v/v) ethanol for 24 h. Afterwards, ethanol was discarded by centrifugation. Fibroblasts were rehydrated with PBS at room temperature and resuspended in PI DNA staining buffer and incubated for 30 min at room temperature in the dark. Detection was performed on a flow cytometer (FACSAria; Becton, Dickinson and Co. Biosciences, Franklin Lakes, NJ, USA).
RNA extraction and reverse transcription quantitative polymerase chain reaction
Total RNA was isolated from the cells and tissues using TRIzol reagent, according to the manufacturer’s instructions (Invitrogen, Carlsbad, CA, USA). Complementary DNA (cDNA) was generated from 1 µg total RNA using SuperScript III (Invitrogen, USA) and polyN primers. qPCRwas performed using the StepOne and StepOne Plus real-time PCR system (Applied Biosystems, Foster City, CA, USA). The relative levels of gene expression were represented as 2 –ΔΔCt. The experiments were repeated in triplicate. The sequence of indicated primers was listed as follow: USP41 forward, 5'-TGGAGGGCAGTATGAGCTTTTT-3', and reverse, 5'-ATGACCGGAGTCTGCCATTC-3'; RACK1 forward, 5'-CCACCACGAGGCGATTTGT-3', and reverse, 5'-CCCAGGGTATTCCATAGCTTGAT-3'; β-actin forward, 5'-CATGTACGTTGCTATCCAGGC-3', and reverse, 5'-CTCCTTAATGTCACGCACGAT-3'.
Western blot
Cells were homogenized and lysed with radioimmunoprecipitation assay (RIPA) lysis buffer [100 mM NaCl, 50 mM Tris-HCl pH 7.5, 1% TritonX-100, 1 mM ethylenediamine tetraacetic acid (EDTA), 10 mM b-glycerophosphate, 2 mM sodium vanadate and protease inhibitor]. Protein concentration was assayed using the micro-bicinchoninic acid (BCA) protein assay (Pierce, Rockford, IL, USA). A quantity of 40l g of protein per lane was separated by 12% sodium dodecyl sulfate-polyacrylamide gel electrophoresis (SDS-PAGE) and electroblotted onto nitrocellulose (Amersham Pharmacia, Freiburg, Germany). Then, the membrane was blocked with 5% non-fat milk and incubated with antibodies against proteins. Incubation with the primary antibody was carried out overnight in a cold room. The membrane was then incubated with a secondary antibody conjugated to goat anti-mouse IgG (1:5,000, Sigma-Aldrich, St. Louis, MO, USA) and developed using enhanced chemiluminescence (ECL; Amersham Pharmacia, Piscataway, NJ, USA).
Co-immunoprecipitation and mass spectrometry
Co-immunoprecipitation and mass spectrometry (CoIP-MS) was used to explore the interacting proteins with USP41. The process consisted of 5 main steps: (I) incubation of cell lysates with antibodies, (II) binding of immune complexes to protein A/G agarose resin, (III) removal of non-interacting proteins, (IV) elution to obtain protein interacting complexes, (V) mass spectrometry identification of protein interacting complexes. The enriched co-immunoprecipitation products were analyzed by mass spectrometry. Peptides with scores b20 were removed, and higher scores meant a better degree of matching with the secondary atlas. Peptides were searched and compared qualitatively in UniProt (https://www.uniprot.org/). The UniquePep Count and the Cover Percent were also evaluated as auxiliary metrics for the final identification results.
Statistical analysis
The data were presented as means ± SD. Student’s t-test was used for statistical analysis, unless otherwise indicated. GraphPad Prism 8 (GraphPad Software Inc., La Jolla, CA, USA) and SPSS 23.0 Software (IBM Inc., Armonk, NY, USA) were used for all statistical analyses. Statistically significant differences were indicated when P<0.05.
Results
Overexpression of USP41 in BC cell lines and tissues
To investigate the role of USP41 in BC progression, we determined its expression in BC. Our TCGA database analysis revealed that in metastatic BC, USP41 expression was upregulated (Figure 1A). The overexpression of USP41 was negatively correlated with BC prognosis (Figure 1B). The protein levels of USP41 were higher in tumor tissues than their adjacent non-tumorous tissues in 10 pairs of clinical BC specimens (Figure 1C).
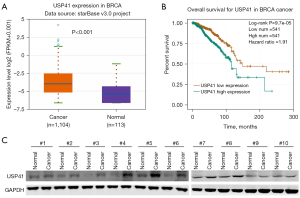
Establishment of stable USP41 expression in BC cell lines
To further explore the function of USP41, we used small interfering RNA (siRNA) to generate a stable cell line (MCF7 and MDA-MB-231 BC cells) in which USP41 was knocked down. We also established USP41 overexpression cell lines by transfecting MCF7 and MDA-MB-231 cells. Transfection efficiency was confirmed by western blot analysis and reverse transcription-quantitative polymerase chain reaction (RT-qPCR). Compared with the cells transfected with the control siRNA, the cells that had been transfected with the USP41 siRNA plasmid exhibited a significantly decreased expression of USP41 at both the protein and mRNA level (Figure 2A,2B). On the contrary, the MCF7 and MDA-MB-231 BC cells that had been transfected with the USP41 overexpression plasmid exhibited obviously increased expression of USP41 at both the protein and mRNA level (Figure 2C,2D).
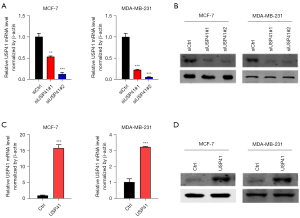
Effects of USP41 gene on BC growth
The CCK-8 and colony formation assays were used to exam the effects of USP41 expression on BC cell proliferation. As shown in Figure 3, knockdown of USP41 suppressed the proliferation of MCF-7 and MDA-MB-231 cells (Figure 3A,3C). In contrast, the overexpression of USP41 significantly enhanced the proliferation of MCF-7 cells and MDA-MB-231 cells (Figure 3B,3D). Knockdown of USP41 reduced the colony-forming ability of MCF 7 cells and MDA-MB-231 cells (Figure 3E), while overexpression of USP41 promoted the colony-forming ability of MCF-7 cells and MDA-MB-231 cells (Figure 3F).
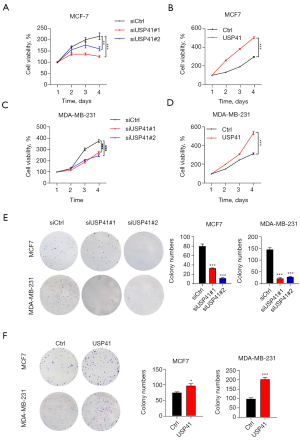
Effects of USP41 gene on BC migration
Transwell assay indicated that USP41 inhibition inhibited the migration compared to control cells in MCF-7 cell line (Figure 4A) and MDA-MB-231 cell line (Figure 4B). Meanwhile, the overexpression of USP41 enhanced cell invasion in MCF-7 cells (Figure 4C). Identically, USP41 overexpression promoted cell migration in MDA-MB-231 cell line (Figure 4D).
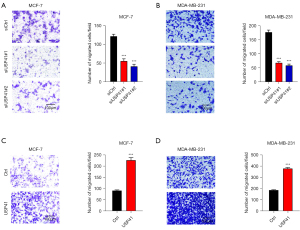
Effects of USP41 gene on BC cell cycle and apoptosis
We next confirmed the role of USP41 in BC cell cycle and apoptosis. As shown in Figure 5A,5B, knockdown of USP41 enhanced cell apoptosis in MCF-7 and MDA-MB-231 cells. Cell cycle arrests of MCF-7 and MDA-MB-231 were also studied. It was shown that USP41 knockdown could arrest cells in G0/G1 phase (Figure 5C,5D).
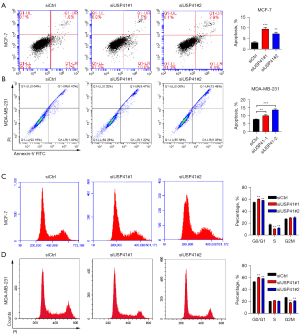
USP41 interacted with RACK1 in BC cells
Study of protein-protein interactions (PPIs) is an essential process to understand the biological functions of proteins and its underlying mechanism (19). Here, we performed CoIP-MS to discover novel proteins interacting with USP41. The CoIP-MS analyses identified a list of genes that interacted with USP41, including RACK1. The Co-IP results indicated that USP41 could interact with RACK1 (Figure 6A). Immunoblotting results showed that USP41 promoted the protein expression of RACK1 (Figure 6B). We also found the overexpression of RACK1 in BC tissues, compared with those in the adjacent normal tissue samples (Figure 6C). Next, we inhibited the expression of RACK1 by gene knockdown (Figure 6D). Colony-forming assay demonstrated that knockdown of RACK1 reduced the colony-forming ability of BC cells (Figure 6E,6F). Transwell assay indicated that knockdown of RACK1 inhibited the migration compared to control cells (Figure 6G).
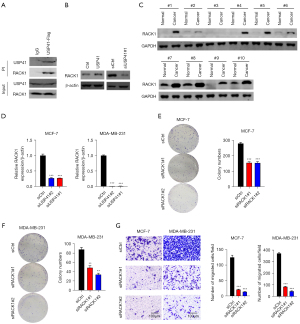
Knockdown of RACK1 reversed the oncogenic function of USP41 in BC cells.
At last, we knocked down RACK1 in USP41 overexpressed BC cells (Figure 7A). When USP41 overexpression significantly promoted the proliferation, colony formation, and cell invasion of MCF-7 and MDA-MB-231 cells, knockdown of RACK1 could obviously reduce the growth and invasion ability (Figure 7B-7D). Thus, USP41 promoted BC function through upregulating RACK1.
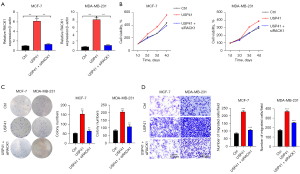
Discussion
The USPs are emerging as potential therapeutic targets in many diseases. In BC, several USPs were overexpressed, such as USP9X, USP15, USP32, USP9Y, USP10, USP25, USP4, and USP11 (20). In 2016, Li et al. showed that USP4 could inhibit BC cell growth through the upregulation of PDCD4 (21). Both USP14 and USP21 were also involved in the progression of BC (22,23). In 2018, USP2-69 was found over-expressed in breast invasive ductal carcinoma, and was closely related to proliferation promoting effects (24). Qiu et al. demonstrated that USP8 might predict better clinical characteristics and might be a protective factor for patients with BC (25). In 2020, USP8 was proved as a positive regulator of Notch signaling, offering a therapeutic target for BC (26). Pal et al. claimed that targeting USPs as BC treatment is especially promising (27). However, no results have been reported for USP41 in BC, even in tumors. In this study, USP41 was found overexpressed in BC and shown to be associated with the cancer progression for the first time.
A member of the tryptophan-aspartate-40 (WD-40) family of proteins, RACK1 is an evolutionarily conserved a 36 kDa scaffold protein with 7 WD-40 repeats, containing the subunit of G-proteins (28,29). The WD40 repeats of RACK1 provides the complex protein-protein bio-interactions among signaling molecules such as integrins, phosphodiesterase 4D5, and Src tyrosine kinase, as well as protein kinase C (PKC) (30). It seems that RACK1 is essential for cellular functions because its amino acid sequence in human is 100% consistent with that in rats, chickens, mice, and cows (31). As reported, RACK1 can interact with numerous signaling proteins and is regarded as a scaffolding, anchor, or adaptor protein, participating into multiple intracellular signal transduction, immune defense, cell growth, and migration pathways (32). In 2018, RACK1 was verified as an oncogene in colon cancer (33). In 2020, RACK1 overexpression was demonstrated to dampen the progression of cervical squamous cell carcinoma (34). Wu et al. showed that RACK1 stimulates tumor invasion and lymph node metastasis of cervical cancer, providing promising means for cervical cancer treatment (35). Dan et al. found that RACK1 promotes cancer progression by increasing the M2/M1 macrophage ratio in oral squamous cell carcinoma (36). For BC, RACK1 was firstly identified as a superior independent biomarker for diagnosis and prognosis in 2010. Comparing with conventional diagnostic index [Ki67, estrogen receptor (ER), progesterone receptor (PR) and HER-2] in BC, RACK1 possessed superiority in sensitivity and specificity as biomarker (37). In this study, we also found the overexpression of RACK1 in BC and its potential role in proliferation and migration. However, the mechanisms through which RACK1 regulates BC progression are still undetermined
The USPs-regulated signaling pathways implicated in BC are more likely to be transforming growth factor beta (TGF-β) signaling, which has a well-documented role in mediating epithelial-to-mesenchymal transition (EMT), tumor progression, and metastasis (20). In 2012, USP11 was found to augment TGF-β signaling by deubiquitylating ALK5 (38). Eichhorn et al. demonstrated that USP15 stabilized TGF-β receptor I and promoted oncogenesis through the activation of TGF-β signaling (39). In 2019, Zhang et al. showed that USP4 inhibition prevented the activity of the TGF-β/Smad signaling pathway (40). Galant et al. found that overexpression of several USPs amplifies fibrotic responses induced by TGF-β (41). In this study, USP41 was found to be associated with RACK1 and RACK1 has been identified as concomitant regulator of TGF-β1 (42). Zhou et al. indicated that RACK1 can inhibit collagen synthesis in KFs via inhibition of the TGF-β1/Smad signaling pathway (43). Silencing of RACK1was shown to attenuate renal fibrosis by inhibiting TGF-β signaling (44). Above all, USP41 may exert an oncogenic function via TGF-β signaling in combination with RACK1. However, the mechanisms through which RACK1 regulates BC are wide ranging and diverse. Further study is still needed to explore its role in USP41 mediated BC progression.
In conclusion, USP41 overexpression promotes the proliferation and invasion of BC by combination with RACK1, which may serve as an emerging therapeutic potential target for BC treatment.
Acknowledgments
Funding: This work was supported by The National Natural Science Foundation of China (81572917); Key Project of Natural Science Foundation of Shaanxi Province (2021JZ-29); Key Research and Development Plan of Shaanxi Province (2018ZDXM-SF-066); Project of Xijing Hospital (XJZT18MJ30).
Footnote
Reporting Checklist: The authors have completed the MDAR reporting checklist. Available at https://dx.doi.org/10.21037/atm-21-4921
Data Sharing Statement: Available at https://dx.doi.org/10.21037/atm-21-4921
Conflicts of Interest: All authors have completed the ICMJE uniform disclosure form (available at https://dx.doi.org/10.21037/atm-21-4921). The authors have no conflicts of interest to declare.
Ethical Statement: The authors are accountable for all aspects of the work in ensuring that questions related to the accuracy or integrity of any part of the work are appropriately investigated and resolved. The study was approved by an independent ethics committee of Fourth Military Medical University (KY20213157-1). The study was undertaken in accordance with the Good Clinical Practice guidelines and the Declaration of Helsinki (as revised in 2013). All participants were asked to provide written informed consent before enrollment.
Open Access Statement: This is an Open Access article distributed in accordance with the Creative Commons Attribution-NonCommercial-NoDerivs 4.0 International License (CC BY-NC-ND 4.0), which permits the non-commercial replication and distribution of the article with the strict proviso that no changes or edits are made and the original work is properly cited (including links to both the formal publication through the relevant DOI and the license). See: https://creativecommons.org/licenses/by-nc-nd/4.0/.
References
- Siegel RL, Miller KD, Jemal A. Cancer statistics, 2019. CA Cancer J Clin 2019;69:7-34. [Crossref] [PubMed]
- DeSantis CE, Ma J, Gaudet MM, et al. Breast cancer statistics, 2019. CA Cancer J Clin 2019;69:438-51. [Crossref] [PubMed]
- Chen W, Zheng R, Zuo T, et al. National cancer incidence and mortality in China, 2012. Chin J Cancer Res 2016;28:1-11. [PubMed]
- Zeng H, Zheng R, Guo Y, et al. Cancer survival in China, 2003-2005: a population-based study. Int J Cancer 2015;136:1921-30. [Crossref] [PubMed]
- Greenwalt I, Zaza N, Das S, et al. Precision Medicine and Targeted Therapies in Breast Cancer. Surg Oncol Clin N Am 2020;29:51-62. [Crossref] [PubMed]
- Bombonati A, Sgroi DC. The molecular pathology of breast cancer progression. J Pathol 2011;223:307-17. [Crossref] [PubMed]
- Jiang J, Yang X, He X, et al. MicroRNA-449b-5p suppresses the growth and invasion of breast cancer cells via inhibiting CREPT-mediated Wnt/β-catenin signaling. Chem Biol Interact 2019;302:74-82. [Crossref] [PubMed]
- Clague MJ, Urbé S. Ubiquitin: same molecule, different degradation pathways. Cell 2010;143:682-5. [Crossref] [PubMed]
- Mansour MA. Ubiquitination: Friend and foe in cancer. Int J Biochem Cell Biol 2018;101:80-93. [Crossref] [PubMed]
- Pereira RV. Ubiquitin-specific proteases are differentially expressed throughout the Schistosoma mansoni life cycle. Parasit Vectors 2015;8:349. [Crossref] [PubMed]
- Mevissen TET, Komander D. Mechanisms of Deubiquitinase Specificity and Regulation. Annu Rev Biochem 2017;86:159-92. [Crossref] [PubMed]
- Zhang K, Yang L, Wang J, et al. Ubiquitin-specific protease 22 is critical to in vivo angiogenesis, growth and metastasis of non-small cell lung cancer. Cell Commun Signal 2019;17:167. [Crossref] [PubMed]
- Geng L, Chen X, Zhang M, et al. Ubiquitin-specific protease 14 promotes prostate cancer progression through deubiquitinating the transcriptional factor ATF2. Biochem Biophys Res Commun 2020;524:16-21. [Crossref] [PubMed]
- Lai CY, Yeh DW, Lu CH, et al. Epigenetic Silencing of Ubiquitin Specific Protease 4 by Snail1 Contributes to Macrophage-Dependent Inflammation and Therapeutic Resistance in Lung Cancer. Cancers (Basel) 2020;12:148. [Crossref] [PubMed]
- Yun SI, Hong HK, Yeo SY, et al. Ubiquitin-Specific Protease 21 Promotes Colorectal Cancer Metastasis by Acting as a Fra-1 Deubiquitinase. Cancers (Basel) 2020;12:207. [Crossref] [PubMed]
- Quesada V, Díaz-Perales A, Gutiérrez-Fernández A, et al. Cloning and enzymatic analysis of 22 novel human ubiquitin-specific proteases. Biochem Biophys Res Commun 2004;314:54-62. [Crossref] [PubMed]
- Ji J, Yang S, Zu L, et al. Deubiquitinating enzyme USP41 promotes lung cancer cell proliferation and migration. Thorac Cancer 2021;12:1041-7. [Crossref] [PubMed]
- Young MJ, Hsu KC, Lin TE, et al. The role of ubiquitin-specific peptidases in cancer progression. J Biomed Sci 2019;26:42. [Crossref] [PubMed]
- Chen R, Xiao M, Gao H, et al. Identification of a novel mitochondrial interacting protein of C1QBP using subcellular fractionation coupled with CoIP-MS. Anal Bioanal Chem 2016;408:1557-64. [Crossref] [PubMed]
- Pal A, Donato NJ. Ubiquitin-specific proteases as therapeutic targets for the treatment of breast cancer. Breast Cancer Res 2014;16:461. [Crossref] [PubMed]
- Li Y, Jiang D, Zhang Q, et al. Ubiquitin-specific protease 4 inhibits breast cancer cell growth through the upregulation of PDCD4. Int J Mol Med 2016;38:803-11. [Crossref] [PubMed]
- Zhu L, Yang S, He S, et al. Downregulation of ubiquitin-specific protease 14 (USP14) inhibits breast cancer cell proliferation and metastasis, but promotes apoptosis. J Mol Histol 2016;47:69-80. [Crossref] [PubMed]
- Peng L, Hu Y, Chen D, et al. Ubiquitin specific protease 21 upregulation in breast cancer promotes cell tumorigenic capability and is associated with the NOD-like receptor signaling pathway. Oncol Lett 2016;12:4531-7. [Crossref] [PubMed]
- Liu JY, Zou LP, Wu HJ, et al. Effects of ubiquitin-specific proteases 2-69 on proliferation of breast cancer cells. Zhonghua Bing Li Xue Za Zhi 2018;47:455-60. [PubMed]
- Qiu H, Kong J, Cheng Y, et al. The expression of ubiquitin-specific peptidase 8 and its prognostic role in patients with breast cancer. J Cell Biochem 2018;119:10051-8. [Crossref] [PubMed]
- Shin S, Kim K, Kim HR, et al. Deubiquitylation and stabilization of Notch1 intracellular domain by ubiquitin-specific protease 8 enhance tumorigenesis in breast cancer. Cell Death Differ 2020;27:1341-54. [Crossref] [PubMed]
- Pal A, Donato NJ. Ubiquitin-specific proteases as therapeutic targets for the treatment of breast cancer. Breast Cancer Res 2014;16:461. [Crossref] [PubMed]
- Kershner L, Welshhans K. RACK1 regulates neural development. Neural Regen Res 2017;12:1036-9. [Crossref] [PubMed]
- Adams DR, Ron D, Kiely PA. RACK1, A multifaceted scaffolding protein: Structure and function. Cell Commun Signal 2011;9:22. [Crossref] [PubMed]
- Ren Q, Zhou J, Zhao XF, et al. Molecular cloning and characterization of a receptor for activated protein kinase C1 (RACK1) from Chinese white shrimp; Fenneropenaeus chinensis. Dev Comp Immunol 2011;35:629-34. [Crossref] [PubMed]
- Nagashio R, Sato Y, Matsumoto T, et al. Expression of RACK1 is a novel biomarker in pulmonary adenocarcinomas. Lung Cancer 2010;69:54-9. [Crossref] [PubMed]
- Hu L, Lu F, Wang Y, et al. RACK1, a novel hPER1-interacting protein. J Mol Neurosci 2006;29:55-63. [Crossref] [PubMed]
- Xiao T, Zhu W, Huang W, et al. RACK1 promotes tumorigenicity of colon cancer by inducing cell autophagy. Cell Death Dis 2018;9:1148. [Crossref] [PubMed]
- Wang J, Chen S. RACK1 promotes miR-302b/c/d-3p expression and inhibits CCNO expression to induce cell apoptosis in cervical squamous cell carcinoma. Cancer Cell Int 2020;20:385. [Crossref] [PubMed]
- Wu H, Song S, Yan A, et al. RACK1 promotes the invasive activities and lymph node metastasis of cervical cancer via galectin-1. Cancer Lett 2020;469:287-300. [Crossref] [PubMed]
- Dan H, Liu S, Liu J, et al. RACK1 promotes cancer progression by increasing the M2/M1 macrophage ratio via the NF-κB pathway in oral squamous cell carcinoma. Mol Oncol 2020;14:795-807. [Crossref] [PubMed]
- Cao XX, Xu JD, Liu XL, et al. RACK1: A superior independent predictor for poor clinical outcome in breast cancer. Int J Cancer 2010;127:1172-9. [Crossref] [PubMed]
- Al-Salihi MA, Herhaus L, Macartney T, et al. USP11 augments TGFβ signalling by deubiquitylating ALK5. Open Biol 2012;2:120063 [Crossref] [PubMed]
- Eichhorn PJ, Rodón L, Gonzàlez-Juncà A, et al. USP15 stabilizes TGF-β receptor I and promotes oncogenesis through the activation of TGF-β signaling in glioblastoma. Nat Med 2012;18:429-35. [Crossref] [PubMed]
- Zhang J, Na S, Pan S, et al. Inhibition of USP4 attenuates pathological scarring by downregulation of the TGF-β/Smad signaling pathway. Mol Med Rep 2019;20:1429-35. [Crossref] [PubMed]
- Galant C, Marchandise J, Stoenoiu MS, et al. Overexpression of ubiquitin-specific peptidase 15 in systemic sclerosis fibroblasts increases response to transforming growth factor β. Rheumatology (Oxford) 2019;58:708-18. [Crossref] [PubMed]
- Pu Y, Liu YQ, Zhou Y, et al. Dual role of RACK1 in airway epithelial mesenchymal transition and apoptosis. J Cell Mol Med 2020;24:3656-68. [Crossref] [PubMed]
- Zhou P, Shi L, Li Q, et al. Overexpression of RACK1 inhibits collagen synthesis in keloid fibroblasts via inhibition of transforming growth factor-β1/Smad signaling pathway. Int J Clin Exp Med 2015;8:15262-8. [PubMed]
- Feng J, Xie L, Kong R, et al. RACK1 silencing attenuates renal fibrosis by inhibiting TGF-β signaling. Int J Mol Med 2017;40:1965-70. [Crossref] [PubMed]
(English Language Editor: J. Jones)