Study on the preparation and function of regulatory T cells from human peripheral blood
Introduction
The immune system has evolved to protect against pathogenic microbes and to provide regulation of physiological homeostasis. The immune tolerance and suppression had been long known, however, the main cell type responsible for this phenomenon was not identified until the 1990s. Sakaguchi S and colleagues discovered that a subset of CD4+ T cells constitutively expressing of the cytokine interleukin (IL)-2 receptor α-chain (CD25), known as regulatory T cells (Treg), were able to prevent autoimmunity upon transfer into thymectomized mice (1). Treg cells can be identified by the lineage marker forkhead box protein P3 (FOXP3), which is required for their development, function and stability. Mutations of FOXP3 gene lead to the development of immunodysregulation polyendocrinopathy enteropathy X-linked (IPEX) syndrome, due to the absence or dysfunction of Treg cells (2). As CD25 and FOXP3 are also expressed by activated CD4+ T cells, absence of the IL-7 receptor α-chain (CD127) is usually used as a complementary marker for Treg cells (3). Studies have shown that Treg cells can secrete immunosuppressive cytokines [such as transforming growth factor-β (TGF-β), IL-10, IL-35, etc.] and regulate the proliferation and activation of effector T cells (Teffs) and dendritic cells through these cytokines. Treg cells also express a variety of immunosuppressive molecules [such as cytotoxic T lymphocyte associated antigen 4 (CTLA-4), lymphocyte activation gene 3 (LAG-3), etc.] to directly inhibiting the activity of target cells. In addition, CD25, when highly expressed in Treg cells, can compete for IL-2 required by Teff cells, and Treg cells can also secrete granzymes to directly kill Teffs.
The balance of the human immune system is maintained by Treg cells and Teff cells, and once the balance between the two is broken, many diseases can emerge (4). An excessive number of Treg cells is closely associated with the emergence of tumors and infectious diseases, while an insufficient number of Treg cells can lead to autoimmune diseases. Studies have shown that the number of Treg cells was decreased or the function of Treg cells was compromised in a variety of autoimmune diseases, including multiple sclerosis (5), systemic lupus erythematosus (6), type I diabetes (7), rheumatoid arthritis (8), inflammatory bowel disease (9), and psoriasis (10), etc. This suggests that an intervention into the number and function of Treg cells may be an effective approach for treating a variety of autoimmune diseases.
Under normal conditions, the number of Tregs in the body is very small, which account for 5% to 10% of CD4+ T lymphocytes. Therefore, it is necessary to establish an effective expansion method to produce a sufficient number of functional Treg cells to meet the needs of clinical research. There were various protocols in the literature that elucidating the isolation and culture methods of human Treg cells. However, the expansion fold of Treg cells was low, and the expression of FOXP3 was not stable. In this study we used CD3/CD28 Dynabeads instead of monoclonal anti-CD3 antibodies and anti-CD28 antibodies to stimulate Treg cells activation and expansion which could be easily removed. And we added rapamycin in the culture medium to maintain FOXP3 expression of Treg cells. The Treg cells expanded by this optimized protocol exhibited high expansion fold, purity and immunosuppressive activity.
We present the following article in accordance with the MDAR reporting checklist (available at https://dx.doi.org/10.21037/atm-21-3812).
Methods
Main materials and reagents
The study was conducted in accordance with the 2013 Declaration of Helsinki. The informed consent was taken from all the volunteers. Peripheral blood samples were collected from 12 healthy volunteers. Among them, 8 were males and 4 were females, ranging in age from 23 to 75 years, with a median age of 60.5 years. Human lymphocyte separation solution was purchased from Tianjin Haoyang Biotechnology (Tianjin, China); a CD4+CD25+CD127dim/− cell sorting kit was purchased from Miltenyi Biotec (Germany); human AB serum was purchased from Thermo Fisher Scientific (USA); CD3/CD28 Dynabeads were purchased from Thermo Fisher Scientific (USA); IL-2 was purchased from Jiangsu Kingsley Pharmaceutical (China); rapamycin was purchased from Milteny Biotec (Germany); fixation/breaking membrane buffer, and fluorescent dyes were purchased from BioLegend (USA); and dimethyl sulfoxide (DMSO) was purchased from Santa Cruz Biotechnology, Inc (Dallas, TX, USA). The antibodies used in flow cytometry were purchased from BioLegend (USA) (Table S1). The study was conducted in accordance with the Declaration of Helsinki (as revised in 2013). The study was approved by Medical Ethics Committee of Sichuan University (No. K20210106) and informed consent was taken from all the volunteers.
Isolation and purification of human peripheral blood Tregs
Peripheral blood and phosphate-buffered saline (PBS) were mixed at a volume ratio of 1:2 and slowly added to a centrifuge tube prefilled with an equal volume of human lymphocyte separation solution. Conventional density gradient centrifugation was then used to separate the mononuclear cells and was followed by the immunomagnetic bead method to separate the Treg cells. According to the operating instructions for the CD4+CD25+CD127dim/− cell sorting kit, magnetic bead negative sorting was performed to remove CD4− T cells, while magnetic bead positive sorting was performed to isolate CD25+ cells.
In vitro expansion and culture of Tregs
The isolated and purified Treg cells were cultured in a basal medium, and human AB serum (5% by volume), IL-2 (1,000 U/mL), and rapamycin (100 nM) were added; on day 1 and day 14, CD3/CD28 Dynabeads was for stimulation. Culture medium was added every 2 to 3 days, and the culture period was 21 days.
Phenotype identification of Tregs
First, 1×106 cells were incubated with CD4−APC and CD25−PE antibodies for 30 minutes, washed with fixation/breaking buffer, incubated with FOXP3−FITC (forkhead box protein p3-fluorescein isothiocyanate) antibody for 60 minutes, and finally detected by flow cytometry. The detection of other molecules, including CD3, CD8, CD19, CD45RA, CD56, and HLA-DR (human leukocyte antigen-DR) on the surface of Tregs was conducted according to conventional methods.
Determination of the immunomodulatory function of Tregs
Freshly isolated PBMCs were used as target cells, and the target cells were labeled with CFSE (carboxyfluorescein succinimidyl ester) (final concentration of 5 µM). The Treg cells and PBMCs were then placed in a round-bottomed 96-well plate at ratios of 1:0, 1:1, 1:2, 1:4, 1:8, 1:16, and 0:1. After 3 to 4 days, the cells were collected for flow cytometric analysis. The proliferation level is expressed by the ratio of proliferation peaks. The cell proliferation inhibition rate was calculated as follows: rate = 100% × (proliferation peak ratio of the experimental group without Treg cell to the proliferation peaks in the coculture group with different target ratios)/proliferation peak ratio of the experimental group without Treg cells.
Statistical analysis
The data in this paper are expressed as mean ± standard deviation. GraphPad Prism 8 statistical software (GraphPad Software Inc., San Diego, CA, USA) was used for single-factor t-test analysis. A P value <0.05 indicates that the difference was statistically significant.
Results
Magnetic bead sorting results of human peripheral blood Treg cells
The results of flow cytometry showed that the proportion of CD25+FoxP3+ Treg cells in the peripheral blood of healthy people to peripheral CD4+ T cells was about (6.25±1.73)%. After sorting was performed with CD4+CD25+CD127dim/− immunomagnetic beads, Treg cells with a purity of (95.39±0.81)% could be obtained (Figure 1). Moreover, in the analysis of the magnetic bead sorting results of 12 volunteers, the Treg sorting rate of the ≤60 years group was (0.4863±0.1886)% and that of the >60 years group was (0.4250±0.0998)%. The difference was not statistically significant (P>0.05; Figure 2A).
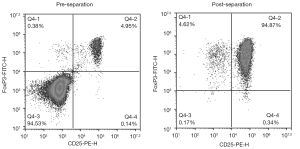
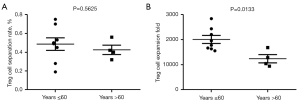
In vitro expansion of human peripheral blood Treg cells
Under the combined stimulation of CD3/CD28 Dynabeads, human AB serum, IL-2, and rapamycin, Treg cells cultured in vitro expanded markedly. At the end of the third week of culture, the cell count results indicated that the expansion factor of Treg cells in the >60 years group was 2,009±452.2, while the expansion factor of the Treg cells in the >60 years group was 1,238±330.0, which represented a statistically significant difference (Figure 2B).
Phenotype and purity identification of Treg cells after expansion
After expansion, Treg cells formed aggregates of varying cell numbers (Figure S1). The samples on the 14th day and 21st day of the amplification were taken for flow cytometry. Representative flow cytometry gating strategy was shown in Figure S2. We analyzed the phenotype of Treg cells post-expansion by quantitative analysis of CD4+CD25+ cells and FOXP3+ cells. The results showed that the ratio of CD4+CD25+ cells on day 14 was (93.25±3.05)%, while the ratio of FOXP3+ cells was (94.19±4.21)%; on day 21, the CD4+CD25+ cells ratio was (92.86±4.36)%, and the ratio of FOXP3+ cells was (91.55±5.62)% (Figure 3).
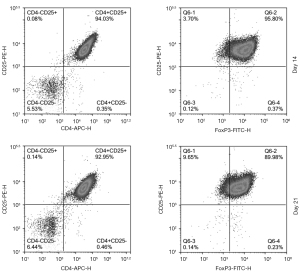
The purity of the sample on the 21st day was detected by flow cytometry. The results showed that the proportions of CD8+ T cells, CD19+ B cells, CD3−CD56+ NK cells, and CD3+ CD56+ NKT cells were very low (Figure 4). These results indicated that the purity of expanded Tregs was relatively high.
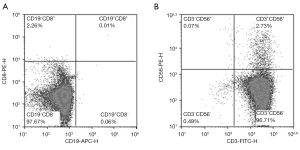
Detection of the immunosuppressive function of Treg cells after expansion
The expanded human peripheral blood Treg cells and PBMCs were subjected to in vitro lymphocyte proliferation experiments in order to analyze the ability of Treg cells to inhibit the proliferation of CD4+ T and CD8+ T cells in PBMCs. We used CFSE staining methods to assessing the proliferation rate of CD3/CD28 Dynabeads-stimulated PBMCs (Figure S3). The ratio of CD8+ T cell proliferation in the experimental group without Treg cells was 44.61% (Figure 5A); the ratio of the CD8+ T cell proliferation was significantly reduced when Treg cells and PBMCs were cocultured at a ratio of 1:1 (6.19%). According to calculations, the proliferation inhibition rates of Treg cells against CD8+ T and CD4+ T cells at a ratio of 1:1 were (84.23±3.43)% and (76.29±4.28)%, respectively, and the inhibition rate and effector to target (E:T) ratios showed an obvious pattern of positive correlation (Figure 5B).
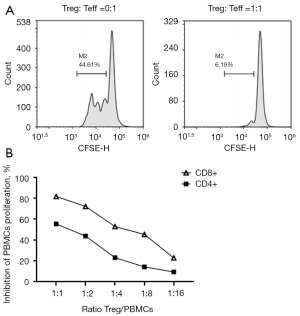
Discussion
Research into Treg cells has been ongoing for more than 20 years. In 1995, Sakaguchi et al. identified CD25 [IL-2 receptor α-chain (IL-2RA)] as a surface marker of Treg cells for the first time. Other researchers found that transplanting lymphocytes with CD4+CD25+ subgroups into nude mice would cause a variety of autoimmune diseases, while introducing CD4+CD25+ Treg cells could inhibit the occurrence of diseases (1). At present, it has been proven in animal models that Treg cells can treat a variety of autoimmune diseases and inhibit graft-versus-host response. However, the clinical use of Treg cells for cell therapy still faces difficulties and challenges (11,12). Existing Chinese studies on the expansion of human peripheral blood Treg cells in vitro show that Treg cells can proliferate 755.5±213.5 times after being cultured in vitro for 14 days (13). In this study, the optimized culture system of CD3/CD28 Dynabeads, human AB serum, IL-2, and rapamycin was found to significantly increase the expansion of Treg cells. We also found that the in vitro expansion fold of Treg cells in the ≤60 years group was significantly higher than that in the >60 years group, which suggests that aging may affect the proliferation ability of Treg cells (14).
The transcription factor FOXP3 has an important regulatory effect on the development and function of Treg cells. The mutation of this gene can cause Treg cell development, differentiation, and dysfunction (15). In the process of in vitro expansion, prolonged antigen stimulation may lead to a decrease in FOXP3 expression and affect the immunosuppressive activity of Treg cells (16). In this study, the Treg cell expansion cycle in vitro was 21 days, but the extension of the cycle did not affect the phenotype of Treg cells. The proportion of FOXP3+ was close to 90%, and there was no significant difference between the 14th day and the 21st day. This was likely a result of the addition of rapamycin in the amplification system. Studies have found that under the condition of continuous IL-2 stimulation, rapamycin activates the signal transducer and activator of transcription-5 (STAT5) pathway by inhibiting the mammalian target of rapamycin (mTOR) signaling pathway, thereby maintaining the proliferation of Treg cells and the expression of FOXP3 (17). The stable expression of FOXP3 can ensure the immunosuppressive activity of Treg cells. The in vitro lymphocyte proliferation inhibition test further confirmed that Treg cells expanded for 21 days can effectively inhibit the proliferation of CD4+ T and CD8+ T cells. We further found that Treg cells have a stronger inhibitory effect on the proliferation of CD8+ T cells, which may be due to the competition of Treg cells to bind more IL-2, resulting in a decrease in the IL-2 concentration in the system; meanwhile, CD8+ T cells are more sensitive at low concentrations of IL-2 (18).
In recent years, the safety and effectiveness of adoptive Treg cell therapy in the treatment of graft-versus-host disease, type I diabetes, Crohn’s disease, and other diseases have been confirmed. Brunstein et al. (19) found that the infusion of cord blood-derived Treg cells before hematopoietic stem cell transplantation can effectively reduce the risk of graft-versus-host reaction. The results of a phase 1 clinical study of adoptive infusion of Treg cells in the treatment of type I diabetes showed that Treg cells can survive in vivo for an extended period and improve the physical function of patients (20). A French multicenter clinical trial confirmed that the infusion of antigen-specific Treg cells is safe to treat refractory Crohn’s disease and can relieve symptoms in a dose-dependent manner (21). In this study, a technique for efficient expansion of human peripheral blood Treg cells in vitro was established. This approach has the characteristics of high expansion efficiency, stable cell phenotype, and strong cell activity. This research has laid the experimental foundation for the application of Treg cells in the clinical treatment of graft-versus-host diseases and autoimmune diseases.
Acknowledgments
Funding: This study was supported by the Science and Technology Department of Sichuan Province Fund (No. 2020YFS0210) and the Tibet Agriculture and Animal Husbandry College Flexible Talent Introduction Project (No. RXYJ1905).
Footnote
Reporting Checklist: The authors have completed the MDAR reporting checklist. Available at https://dx.doi.org/10.21037/atm-21-3812
Data Sharing Statement: Available at https://dx.doi.org/10.21037/atm-21-3812
Conflicts of Interest: All authors have completed the ICMJE uniform disclosure form (available at https://dx.doi.org/10.21037/atm-21-3812). All authors report funding from Science and Technology Department of Sichuan Province Fund (No. 2020YFS0210) and the Tibet Agriculture and Animal Husbandry College Flexible Talent Introduction Project (No. RXYJ1905). The authors have no other conflicts of interest to declare.
Ethical Statement: The authors are accountable for all aspects of the work in ensuring that questions related to the accuracy or integrity of any part of the work are appropriately investigated and resolved. The study was conducted in accordance with the Declaration of Helsinki (as revised in 2013). The study was approved by Medical Ethics Committee of Sichuan University (No. K20210106) and informed consent was taken from all the volunteers.
Open Access Statement: This is an Open Access article distributed in accordance with the Creative Commons Attribution-NonCommercial-NoDerivs 4.0 International License (CC BY-NC-ND 4.0), which permits the non-commercial replication and distribution of the article with the strict proviso that no changes or edits are made and the original work is properly cited (including links to both the formal publication through the relevant DOI and the license). See: https://creativecommons.org/licenses/by-nc-nd/4.0/.
References
- Sakaguchi S, Sakaguchi N, Asano M, et al. Immunologic self-tolerance maintained by activated T cells expressing IL-2 receptor alpha-chains (CD25). Breakdown of a single mechanism of self-tolerance causes various autoimmune diseases. J Immunol 1995;155:1151-64. [PubMed]
- Zheng Y, Rudensky AY. Foxp3 in control of the regulatory T cell lineage. Nat Immunol 2007;8:457-62. [Crossref] [PubMed]
- Liu WH, Putnam AL, Zhou XY, et al. CD127 expression inversely correlates with FoxP3 and suppressive function of human CD4+ T reg cells. J Exp Med 2006;203:1701-11. [Crossref] [PubMed]
- Sakaguchi S, Mikami N, Wing JB, et al. Regulatory T Cells and Human Disease. Annu Rev Immunol 2020;38:541-66. [Crossref] [PubMed]
- Venken K, Hellings N, Liblau R, et al. Disturbed regulatory T cell homeostasis in multiple sclerosis. Trends Mol Med 2010;16:58-68. [Crossref] [PubMed]
- Ohl K, Tenbrock K. Regulatory T cells in systemic lupus erythematosus. Eur J Immunol 2015;45:344-55. [Crossref] [PubMed]
- Long SA, Cerosaletti K, Bollyky PL, et al. Defects in IL-2R signaling contribute to diminished maintenance of FOXP3 expression in CD4(+)CD25(+) regulatory T-cells of type 1 diabetic subjects. Diabetes 2010;59:407-15. [Crossref] [PubMed]
- Ehrenstein MR, Evans JG, Singh A, et al. Compromised function of regulatory T cells in rheumatoid arthritis and reversal by anti-TNFalpha therapy. J Exp Med 2004;200:277-85. [Crossref] [PubMed]
- Maul J, Loddenkemper C, Mundt P, et al. Peripheral and intestinal regulatory CD4+ CD25(high) T cells in inflammatory bowel disease. Gastroenterology 2005;128:1868-78. [Crossref] [PubMed]
- Bovenschen HJ, van de Kerkhof PC, van Erp PE, et al. Foxp3+ regulatory T cells of psoriasis patients easily differentiate into IL-17A-producing cells and are found in lesional skin. J Invest Dermatol 2011;131:1853-60. [Crossref] [PubMed]
- Trzonkowski P, Bacchetta R, Battaglia M, et al. Hurdles in therapy with regulatory T cells. Sci Transl Med 2015;7:304ps18 [Crossref] [PubMed]
- Raffin C, Vo LT, Bluestone JA. Treg cell-based therapies: challenges and perspectives. Nat Rev Immunol 2020;20:158-72. [Crossref] [PubMed]
- Wang X, Wu Q, Wang Y, et al. Establishment of in vitro efficient expansion of human peripheral blood regulatory T cells. Journal of Nanjing Medical University 2018;38:1034-8.
- Guo Z, Wang G, Wu B, et al. DCAF1 regulates Treg senescence via the ROS axis during immunological aging. J Clin Invest 2020;130:5893-908. [Crossref] [PubMed]
- Lu L, Barbi J, Pan F. The regulation of immune tolerance by FOXP3. Nat Rev Immunol 2017;17:703-17. [Crossref] [PubMed]
- Hoffmann P, Boeld TJ, Eder R, et al. Loss of FOXP3 expression in natural human CD4+CD25+ regulatory T cells upon repetitive in vitro stimulation. Eur J Immunol 2009;39:1088-97. [Crossref] [PubMed]
- Fraser H, Safinia N, Grageda N, et al. A Rapamycin-Based GMP-Compatible Process for the Isolation and Expansion of Regulatory T Cells for Clinical Trials. Mol Ther Methods Clin Dev 2018;8:198-209. [Crossref] [PubMed]
- Au-Yeung BB, Smith GA, Mueller JL, et al. IL-2 Modulates the TCR Signaling Threshold for CD8 but Not CD4 T Cell Proliferation on a Single-Cell Level. J Immunol 2017;198:2445-56. [Crossref] [PubMed]
- Brunstein CG, Miller JS, McKenna DH, et al. Umbilical cord blood-derived T regulatory cells to prevent GVHD: kinetics, toxicity profile, and clinical effect. Blood 2016;127:1044-51. [Crossref] [PubMed]
- Bluestone JA, Buckner JH, Fitch M, et al. Type 1 diabetes immunotherapy using polyclonal regulatory T cells. Sci Transl Med 2015;7:315ra189 [Crossref] [PubMed]
- Desreumaux P, Foussat A, Allez M, et al. Safety and efficacy of antigen-specific regulatory T-cell therapy for patients with refractory Crohn's disease. Gastroenterology 2012;143:1207-1217.e2. [Crossref] [PubMed]
(English Language Editor: J. Gray)