Genomics and phenomics of Hashimoto’s thyroiditis in children and adolescents: a prospective study from Southern India
Introduction
Ever since the entity of Hashimoto’s thyroiditis (HT) has been described by Hashimoto in 1912 (1), it has evolved as the most common cause of acquired hypothyroidism in children and adolescents (2-4). It is also known as chronic lymphocytic thyroiditis or auto-immune thyroiditis. HT is most prevalent in adults and elderly women between 45-65 years (3). HT in children rarely occurs before 4 years of age with peak age of incidence around adolescence (10-20 years) (5). The classical clinical presentation of HT is transient or recurrent hyperthyroid episodes, followed by euthyroid phase and finally ending up as sustained hypothyroidism with/without goiter. On the contrary, the clinical picture in children is usually non-classical and also under-reported. The diagnosis of HT is based on demonstration of elevated anti-TPO antibody titer; ultrasound criteria of diffuse hyperechogenicity and pathological features of lymphocytic infiltration, fibrosis and thyrocyte destruction (3). Though initially HT was considered as an immunological entity, with recent knowledge in molecular genetics the etiopathogenesis appears to be influenced by genetic and multiple environmental factors too (6).
The genetic analysis in HT shows two types of susceptibility genes—immune regulatory and thyroid specific genes (7). Thyroid specific genes act on inter- and intra-cellular mileu responsible for normal hormone synthesis. Immunomodulatory genes such as HLA-DR, CTLA-4, PTPN22 determine the thyroid auto-immunity (8). Thyroid specific genes such as Tpo, NIS, Duox2, thyroglobulin, TSH receptor have also been implicated in pathogenesis and thyroid dysfunction (3,9). In addition, the exact genotype—phenotypic correlations and risk categorization of hypothyroid phenotypes resulting from these known mutations are largely speculative. Especially, the genetic studies in pediatric HT are very sparse from Indian sub-continent. In this context, we conducted this study to analyse the existence and clinical implication of mutations in thyroid specific genes in children and adolescents with HT from a South Indian region.
This study is (I) to study the prevalence of dyshormonogenetic mutations in hypothyroid children with HT; (II) to derive phenotypes of hypothyroidism based on genetic mutations along with genotype-phenotypic correlation (GPC); (III) subset analysis of difference in genetic mutations based on sex, family history of hypothyroidism, puberty status, thyroid functional status; (IV) to study the impact of genetic events on natural history and management of cases.
Materials and methods
This is inter-disciplinary study conducted by collaboration between a tertiary care endocrinology hospital, biochemistry department of a teaching medical institute and genetics lab. The study was conducted over the period of 36 months from August 2012 to July 2015. Ethical clearance was obtained for this study from institutional ethical committee from the institute. Written informed consent was obtained from the attendants/guardians of all the children included in the study. We ensured that the study complies with international ethical norms according to Helsinki Declaration—Ethical Principles for Medical Research Involving Human Subjects (10). The inclusion criteria of hypothyroidism was based on demonstration of elevated serum thyroid stimulating hormone (TSH) level with (overt hypothyroidism) or without clinical features of hypothyroidism (subclinical hypothyroidism). The diagnosis of HT was based on demonstration of elevated anti-TPO antibody titer and ultrasonographic criteria of altered diffuse hyperechogenicity. In goitrous subjects, fine needle aspiration cytology (FNAC) findings of lymphocytic infiltrate and hurthle cells served as additional confirmatory evidence. Simplified WHO criteria was employed to grade goiter size (11). This study included 20 patients of hypothyroid children with HT. Only children between 5-18 years were included in the study. Exclusion criteria were malignant goiters, nodular goiters and congenital hypothyroidism cases. Detailed clinical history, history of growth milestones, consanguinity and family history of thyroid disease was documented in a structured proforma. After the diagnosis of hypothyroidism, patients were started on thyroxine replacement at the rate of 4 µg/kg/d with three monthly clinical and biochemical (with serum TSH) evaluation for titrating the dose of thyroxine to maintain euthyroidism. All the cases were followed for a minimum of 18 months. Serum was collected for genetic analysis of both known and unknown mutations. Detailed study protocol is given below.
Sample collection, DNA isolation and amplification
Three milliliters of early morning venous sample is collected from the included children in a sterile silicon coated glass tube. The 50, 25, 200 and 300 µL of serum samples were used for T3, T4, TSH and anti-TPO assay immediately. The 5 mL of blood was collected from forearm in EDTA vial for genetic analysis and stored at −20 °C. DNA was extracted from whole blood using genomic DNA isolation kit using salting out method according to manufacturer’s instructions. DNA quality was checked by UV absorption at 260 and 280 nm by agarose gel electrophoresis. DNA was quantified by spectrophotometric method with absorbance at 260 and 280 nm. The isolated DNA samples were stored at 4 °C for genetic analysis later.
Polymerase chain reaction (PCR) was used to amplify NIS gene, Tpo gene and Duox2 gene fragments of isolated DNA. Cycling conditions were 95 °C for 5 min (1 cycle); at 95 °C for 40 s, at 55 °C for 40 s, at 72 °C for 60 s (for 35 cycles) and final extension at 72 °C for 10 min using one pair of primers annealing at regions of interest. We used 8 sets of primers depending on number of screened SNPs—2 for Duox2; 2 for Tpo and 4 for NIS (sequence and size of primers are detailed in Table S1). Each set consisted of a forward and reverse reading frame. Quality of PCR products were checked with agarose gel electrophoresis. (Details of DNA sequencing and PCR protocols are described in Supplementary note 1, including Figures S1,S2 and Tables S1-S6).
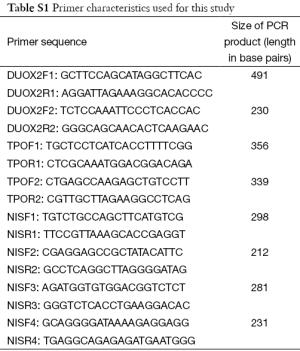
Full table
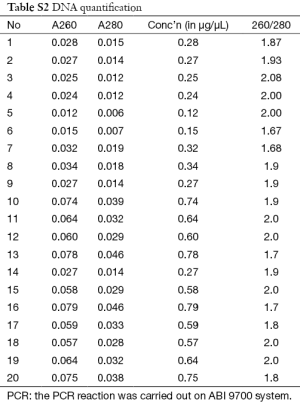
Full table
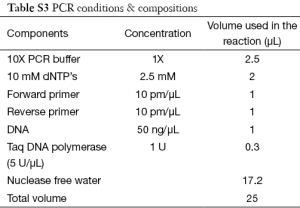
Full table
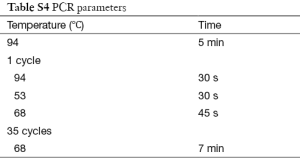
Full table
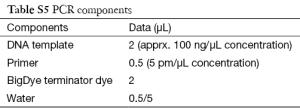
Full table
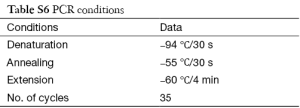
Full table
Mutational analysis of NIS, TPO, DUOX genes
Nucleotide sequences of all amplified PCR products were determined in both orientations by direct sequencing with an Applied Bio-systems 3730XL sequencer (Macrogen, Seoul, South Korea). The results were analyzed using Bio-Edit (v 7.1.3), Sequence scanner (v 1.0; Applied Biosystems Co.) and Nucleotide BLAST programs.
Two types of mutations were looked for—known (mutations already reported in dbSNP database of single nucleotide polymorphisms) and unknown (mutations never reported before). We screened for a total of 142 SNPs with the frequency distribution of 59 SNPs in NIS gene; 41 SNPs in Tpo and 42 SNPs in Duox2 genes [list of all the reference SNPs (refSNPs) are shown in Figure S1]. Known mutations were analysed with restriction fragment length polymorphism (RFLP) analysis. For unknown or novel mutations, we planned to select hotspots on sequencing and study them. The structural and functional analysis of the gene segments consisting mutations was performed.
Laboratory reference ranges of thyroid serum biomarkers (12)
- Serum total tri-iodothyronine (T3) =80-180 ng/dL;
- Serum total thyroxine (T4) =4.5-12.6 µg/dL;
- Serum thyroid stimulating hormone (TSH) =0.4-4.2 µIU/L;
- Serum anti-thyroid peroxidase antibody titer =0-34 IU/L.
The biomarkers were assayed with SIEMENS ADVIA Centaur CP auto-analyser employing Electro-Chemiluminescent Immuno Assay (CLIA). TSH was measured with two site sandwich immunoassay and T3, T4, anti-TPO titers were measured by competitive immunoassay. To study the dietary iodine status, urinary iodine values were measured by modified Sandell and Kolthoff method using ammonium persulfate (13,14). About 95% of individuals had urinary iodine within the optimal range as per UNICEF and ICCIDD guidelines (11).
Definitions employed
Subclinical hypothyroidism
Condition characterized by minimal or no clinical symptoms of hypothyroidism, but associated with elevated serum TSH level and normal T3, T4 levels.
Overt hypothyroidism
Condition characterized by manifest clinical symptoms of hypothyroidism, associated with elevated serum TSH and low T3 and/or T4 levels.
Self-limiting hypothyroidism
Remission of hypothyroidism with normalisation of serum TSH level after withdrawal of thyroxine therapy at the end of follow-up period.
Sustained hypothyroidism
Maintenance of serum TSH level within normal range, only on continued thyroxine therapy at the end of follow-up period.
Statistical, specific data analysis and literature search
SPSS version 18.0 for windows (SPSS Inc., IL, USA) was employed. Descriptive analysis was done with student’s t-test for means and Chi-square test for categorical variables. For impact of genetic mutations of phenotypic attributes of hypothyroidism—univariate and multivariate analysis with logistic regression for qualitative data were employed. P value of <0.05 was considered as statistically significant.
For references, definitions, methodology—apart from text books and journals from library, we searched PubMed, Wikipedia, Google sites on internet with terms such as childhood hypothyroidism, dyshormonogenesis, Natural history of hypothyroidism, thyroid specific genes, HT, congenital hypothyroidism, puberty and thyroid, Duox2, Tpo, NIS genes, thyroglobulin, PCR, DNA sequencing, mutational analysis.
Results
Demographic, clinical and biochemical details of all the 20 children with hypothyroidism due to HT are displayed in Table 1. F:M ratio in our cohort was 7:3 with mean age of 11.05±4.03 years (5-18). Family history of hypothyroidism was present in 8/20 (40%) of patients. Meticulous evaluation showed 9/20 (45%) had at least one of the several possible associated auto-immune (AAI) manifestations. Vitiligo and Eosinophilia with atopy were the most frequent AAI manifestations. The 12/20 (60%) were pre-pubertal and rest post-pubertal. Mean serum TSH titers were 27.7±23.5 mIU/mL (range, 6.5-102.0 mIU/mL). All the cases had elevated anti-TPO antibody titers. Clinically, 7/20 (35%) and 4/20 (20%) children had goitrous HT and painful thyroiditis episodes respectively. Grade I and grade II diffuse firm goiters were found in 5 and 2 children respectively. The 3/4 painful thyroiditis required short term steroid therapy. Mean follow-up of the cohort was 23.5±6 months (range, 18-35 months).
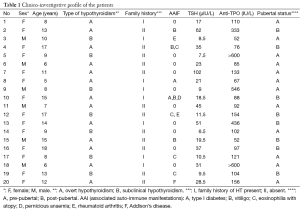
Full table
Table 2 shows comparison of clinic-investigative parameters between children and adolescents. We used an arbitrary cut-off age limit as 12 years to differentiate children from adolescents. Children had increased frequency of subclinical hypothyroidism and family history amongst first degree relatives compared to adolescents. On the contrary, AAI manifestations were less frequent in children. All these parameters reached statistical significance.
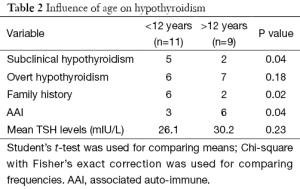
Full table
Table 3 displays the thyroid specific mutations found in this study. We detected 8 mutations in 7/20 (35%) children in the entire cohort (6 in NIS and 2 in Tpo genes. No mutations were observed in Duox2 gene. Table 4 describes the detailed structure and topography of all the detected mutations. Except for bi-allelic, synonymous mutation of Tpo gene in child No. 18, all other mutations were heterozygous in nature (Figure 1). Child No. 5 had dual NIS mutations—one substitutional synonymous and another missense in structure. The coding annotation of this missense mutation was R (CGG)→W (TGG) and L (CTC)→L (CTT) for the silent mutation in Child No. 2. Two synonymous mutations—one TPO and one NIS mutations were found in Child No. 13. All our mutations were localized in introns and we found none in exons. All the heterozygous mutations appear to be recessive in inheritance. Figure 2 shows a representative heterozygous NIS mutation corresponding to a known SNP.
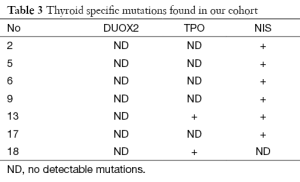
Full table
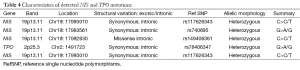
Full table
GPCs in Table 5 show that our mutations significantly expressed the presence of AAI manifestations and existence of family history in this study. Clinical phenotypes of painful thyroiditis, severity of hypothyroidism and absence of goiter were statistically significant in the presence of these mutations. But they could not reach significance on multivariate analysis.
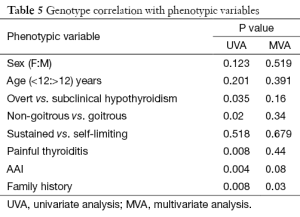
Full table
Discussion
HT or chronic autoimmune thyroiditis is the most common cause of acquired hypothyroidism in children and adolescents (2-4). HT is primarily a disease of adult and elderly women between 45-65 years. It has 10 to 20 times more female sex predilection compared to men (3). HT in children rarely occurs before 4 years of age with peak age of incidence around adolescence (10-12 years) (5). Though, we used an arbitrary cutoff of 12 years to differentiate between children and adolescents, the rationale was based on observations such as increased incidence of HT after 10-12 years; alteration of puberty onset with hypothyroidism; similarity of clinical spectrum in adolescents and young adults. Incidence varies from 0.1% to 3% depending on geographical area and higher dietary iodine content. Though the exact mechanism of higher rate of HT in Iodine-replete areas is elusive, it is hypothesized that excess Iodine stimulates auto-immune cascade leading to thyrocyte disruption by oxidative injury and/or defective Wolf-Chaikoff effect (15-17). Etiopathogenetically, HT has been held as an organ specific immunological disease, later evidence and surge of molecular studies suggests that both genetic and environmental factors influence its genesis (1,18,19). Increased familial risk in twins and first degree relatives have been observed in up to 30-40% in few studies. Many AAI manifestations such as vitiligo, Addison’s disease, insulin dependent diabetes, pernicious anemia, rheumatoid arthritis have been described either in isolation or as part of autoimmune polyglandular syndrome (APS). Accordingly, our cohort had AAI in 45% cases, which were significantly more frequent in adolescents than in children (20).
The classical clinical presentation of HT consists of three phases—initial hyperthyroid (subclinical or overt); euthyroid and final irreversible hypothyroid with/without goiter. The duration of these phases are neither discrete nor sequential in a given individual varying from weeks to months and sometimes with long euthyroid period ending up in hypothyroidism years later after the diagnosis (8). The natural history in children is usually more unpredictable compared to adults as most of the literature on clinical picture and natural history of HT is extrapolation of adult data (21,22). In addition, HT in children and adolescents appears to be reversible in significant proportion of cases and does not correlate with goiter or anti-TPO level unlike in adults. The diagnosis of HT is based on seropositivity of elevated anti-TPO antibody titer along with thyroid dysfunction with or without goiter. Radiologically, ultrasound criteria of diffuse hyperechogenicity (23) and pathologically, FNAC features of lymphocytic infiltration, fibrosis, hurthle cells, plasma cells and thyrocyte destruction are additional confirmatory criteria (24). We utilized clinical, biochemical, radiological criteria to diagnose HT with additional FNAC in goitrous cases. Testifying to the fact that hypothyroidism in child HT is often reversible, in our study 4 cases (20%) (1 in overtly hypothyroid and 3 in subclinically hypothyroid children) were euthyroid without thyroxine therapy by the end of follow-up duration of 18 months. Most of these were subclinical hypothyroidism and natural history is one of the self-limiting HT. Probably, longer follow-up could result in more self-limiting cases of HT. Studies shows that 40-50% of child HT cases with subclinical hypothyroidism are self-limiting/reversible requiring no further thyroxine replacement on long term follow-up (25-27).
Genetic studies have shown that immune modulating genes such as HLA-DR, CD40, CTLA-4, PTPN22 and thyroid specific genes—thyroglobulin and TSH receptor have been implicated in autoimmune thyroid disease (AITD). A Turkish study showed that CTLA4 gene polymorphisms increase the HT predilection in children and adolescents (22). Though TPO, thyroglobulin and TSH receptor have been recognized autoantigens in HT as shown by corresponding anti-TPO, anti-Tg, anti-TSHR antibodies in serum; sodium Iodide symporter (NIS) has also evolved as a novel autoantigen (3). NIS protein mediates active transport of iodide in to thyroid gland, a vital and rate limiting step in thyroid hormone biosynthesis and functional maturation of thyroid gland in newborn (28). NIS protein is regulated by SLCA5A gene, which is located on chromosome 19 at 19p13.11 with 16 exons (29). This was observed in in vitro and animal studies. Even serum of HT patients showed anti-NIS activity inhibiting Iodine uptake at thyrocyte membrane. Thyroid peroxidase (Tpo) gene located on chromosome 2p25 portion with 17 exons regulates, thyroid peroxidase is the major autoantigen in thyroid gland and anti-TPO antibodies have direct correlation with disease activity in HT (30). TPO is the key enzyme mediating the conversion of Iodide to Iodine, a mandatory step for coupling and organification in the production of thyroid hormones. Initially, CD4 T-lymphocyte related cytotoxicity has been implicated, but later evidence suggests that Tpo gene mutations determine the susceptibility of gland to HT. In addition, dual oxidase system (DUOX) characterized by Dual oxidase/dual oxidase maturation factor (DUOXA) NADPH oxidase complexes is the subcellular enzymatic machinery, which regulates the hydrogen peroxide function for TPO catalysed Iodination and coupling enroute effective thyroid hormone synthesis. This Duox2 gene is located on chromosome 15q15.3 with 34 exons (31). New evidence also suggests the role of Duox2 gene mutations in dyshormonogenesis. Though, no definite genetic mutations in Duox2 have been implicated in HT, in vitro studies show that Duox2 gene mutations increase the susceptibility of thyroid gland to Iodine triggered oxidative damage enroute HT (9).
All the mutations found in our study were in intronic regulatory regions of NIS and Tpo genes. These mutations were known SNPs in the gene regulatory regions. The nature of these mutations was synonymous, single allele heterozygous variants. We found no exonic or novel mutations. A mono-allelic defect with haploinsufficiency might cause milder defect in this mechanism leading to delayed onset or subclinical or transient self-limiting hypothyroidism. A more severe defect such as Bi-allelic, homozygous, exonic variants could lead to severe or early hypothyroidism as in congenital hypothyroidism. Though animal studies and in vitro studies endorse the above hypotheses, very few human studies especially from Indian subcontinent have published on the structure and expression of thyroid specific mutations in hypothyroid children. Though, other studies showed that frequent Tpo, and to a lesser extent Duox2 and NIS mutations predispose to HT (15,32,33), higher NIS mutations and absent Duox2 mutations in our study group may be due to geographic and ethnic variations or missed cryptic Duox2 mutations.
One of the main objectives of this study was analysis of GPC, which shows that our mutations do have significant impact on the phenotypic variables clinically. Because we had multiple phenotypic variables, we used reverse Univariate and multivariate analysis with presence or absence of mutations as outcome to study the GPC. We attempted to study the impact of these mutations on various phenotypic traits such as demographic, biochemical, and clinical variables to risk categorize child hypothyroidism. Our study shows that these NIS and Tpo mutations expressed increased frequency of subclinical hypothyroidism with associated autoimmune manifestations and family history in hypothyroid children, reaching statistical significance. If this information can be proven and applied in future widely, it helps in preventive measures at clinical level. We found couple of case-control studies by Srikanta et al. and Balmiki et al., from Eastern part of India trying to risk categorize hypothyroidism based on Tpo genetic polymorphisms (32,34). Though, both the studies risk categorized the subjects based on biochemical phenotype of anti-TPO antibody titer and levels of serum thyroid hormones, but were performed on adult hypothyroidism with very short/no follow-up. Otherwise, to the best of our knowledge and literature search, we found no study from subcontinent with GPC in hypothyroid children and adolescents for comparing our results. Though several genetic studies outside subcontinent have been conducted on hypothyroidism and as many as 500 mutations in Duox2, Tpo and NIS mutations have been reported. While, very few studies have looked in to the influence of genotype on pattern, timing and natural history of hypothyroidism in HT, most of the literature on GPC exists for CH (35,36). Our study tried to find GPC for phenotypic variables such as sex, family history, severity of hypothyroidism, age of patient, AAI manifestations, and pubertal status. Though all our mutations were in introns, we know that some introns play essential role in gene regulatory functions such as non-sense mediated decay and mRNA export (37,38). Moreover, synonymous nature of mutations in this study might be non-silent as suggested by significant GPC. Studies in literature justify that synonymous mutations in non-coding regions can be phenotypically expressive by altering splicing pattern, mRNA, tRNA affinities leading to post-transcriptional and post-translational modification of downstream proteins (39). Though the exact mechanism is elusive, we speculate that above phenomenon might be driving these intronic mutations in to influencing hypothyroid phenotypes. Expressivity of mutations also depends on multiple environmental influences and unknown epigenetic mechanisms. Increased incidence of single allele mutations in our study could be due to undetected cryptic mutations in regulatory gene segments or the other allele.
In addition, the genotype-phenotype correlation with ambitious vision of predicting future clinical course of disease based on genetic mutations is an area of active research for thyroidologists. Though, we are still far away from gene based intervention, this study could act as an early milestone in the path of ultimate aim i.e., to predict/treat susceptibility to hypothyroidism and specific hypothyroid phenotypes based on specific genetic defect pre-emptively. Robust GPCs can facilitate development of protocol for screening families based on specific mutation. In spite of being a smaller study, our results show existence of significant GPC, which can hopefully be unravelled in future with more clarity. This molecular diagnosis allows genetic counseling and screening for asymptomatic carriers in family for specific mutation (40).
The apparent drawbacks of our study are small cohort, shorter follow-up and lack of control group. We conducted this pilot study within the limitations and also it was difficult to procure an ideal control group for children due to hospital based sample, inability to get family history of thyroid disease in controls. The obvious strengths of this study are clinical follow-up of 18 months, focused study on hypothyroidism in children, risk categorization of hypothyroidism based on detected mutations, GPC, exclusion of major confounding factors—Iodine excess or deficiency, congenital hypothyroidism, strong diagnostic criteria for HT, a study first of its kind from South India, and study of genotype-phenotype correlation.
Conclusions
- NIS gene followed by Tpo mutations appears to be most prevalent mutations in HT amongst South Indian children, ethnic and environmental factors may be responsible for this pattern;
- These mutations appear to determine phenotypic expression such as severity of hypothyroidism, goiter, auto-immune manifestations and family history;
- Larger studies are needed to specifically characterize thyroid-specific genomic expression and hypothyroid phenotypes in children and adolescents.
Supplementary Note 1: Details of DNA sequencing and PCR methodology
Quality check and quantification of DNA
The process includes Agarose gel electrophoresis and Spectrophotometric analysis of DNA.
- Agarose gel electrophoresis: electrophoresis was carried out to check the quality of DNA for degradation or any RNA contamination. This 1 µL of sample and electrophoresed on 1% agarose gel.
- Spectrophotometric analysis: The DNA samples were quantified by spectrophotometric method. This 1 µL of sample was diluted 1,000 times and absorbance was taken at 260 and 280 nm. The ratio of 260/280 gives the purity of the samples. The quantification was carried out on Beckmann coulter spectrophotometer DU-520.
Agarose gel electrophoresis of PCR products
The samples were electrophoresed on 1% agarose gel using buffer 1X TAE. For reference both 100 bp ladder (Fermentas) was loaded alongside the samples. For analysis 7 µL of PCR product was taken and 5 µL of 6x Gel loading dye was added and loaded to the agarose gel.
DNA sequencing protocol
Chemicals used
3M Sodium acetate (pH-5.2), absolute ethanol, 70% ethanol (70 mL absolute ethanol and 30 mL water), ABI 3730XL sequencer, ABI Bigdye Terminator kit v3.1.
Procedure
The PCR product cleanup was carried out by enzymatic procedure using ExoSAP. The 3 µL of PCR product was taken and 2 µL of ExoSAP enzyme was added and incubated at 37 °C and further incubated at 80 °C. The product was directly taken up for Sequencing Reaction.
The PCR reaction is performed in two separate reactions one for forward and one for reverse reaction.
The unincorporated dye terminators were removed by performing post-processing step (ethanol precipitation). To 5 µL of PCR product 2 µL of 3M Sodium acetate (pH-5.2) and 80 µL of absolute ethanol was added and centrifuged at 4,500 rpm in Beckman 25R allegra machine for 20 min. Then supernatant was discarded and 120 µL of 70% ethanol was added. The plate was again centrifuged at 4,500 rpm in Beckman 25R allegra machine for 20 min. After discarding the supernatant the DNA pellet was air dried and resuspended in 10 µL of loading solution and loaded onto the machine.
Acknowledgements
We thank the children involved in this study, support staff, genetic analysis team, college administration and hospital staff for ensuring the high quality of this study and outcomes.
Footnote
Conflicts of Interest: The authors have no conflicts of interest to declare.
References
- Hashimoto H. Zur kenntniss der lymphomatosen veranderung der schilddruse (struma lymphomatosa). Arch Klin Chir 1912;97:219-48.
- Tunbridge WM, Evered DC, Hall R, et al. The spectrum of thyroid disease in a community: the Whickham survey. Clin Endocrinol (Oxf) 1977;7:481-93. [PubMed]
- Sar E, Karaoglu A, Yeşilkaya E. Hashimoto’s Thyroiditis in Children and Adolescents. In: Huang FP, editors. Autoimmune Disorders - Current Concepts and Advances from Bedside to Mechanistic Insights. Rijeka: InTech, 2011.
- Kochupillai N. Clinical endocrinology in India. Current Science 2000;79:1061-7.
- Setian NS. Hypothyroidism in children: diagnosis and treatment. J Pediatr (Rio J) 2007;83:S209-16. [PubMed]
- Weetman AP. Autoimmunity and endocrinology. Exp Clin Endocrinol Diabetes 1999;107 Suppl 3:S63-6. [PubMed]
- Brown RS. Autoimmune thyroiditis in childhood. J Clin Res Pediatr Endocrinol 2013;5 Suppl 1:45-9. [PubMed]
- Zaletel K, Gaberšček S. Hashimoto's Thyroiditis: From Genes to the Disease. Curr Genomics 2011;12:576-88. [PubMed]
- Ohye H, Sugawara M. Dual oxidase, hydrogen peroxide and thyroid diseases. Exp Biol Med (Maywood) 2010;235:424-33. [PubMed]
- World Medical Organization. Declaration of Helsinki. BMJ 1996;313:1448-9.
- World Health Organization, International Council for Control of Iodine Deficiency Disorders, UNICEF. Indicators for assessing iodine deficiency disorders and their control through salt iodization. Geneva: World Health Organization, 1994;1-55.
- Baloch Z, Carayon P, Conte-Devolx B, et al. Laboratory medicine practice guidelines. Laboratory support for the diagnosis and monitoring of thyroid disease. Thyroid 2003;13:3-126. [PubMed]
- Pino S, Fang SL, Braverman LE. Ammonium persulfate: a safe alternative oxidizing reagent for measuring urinary iodine. Clin Chem 1996;42:239-43. [PubMed]
- Sandell EB, Kolthoff IM. Micro determination of iodide by a catalytic method. Mikrochim Acta 1937;1:9-25.
- Teng W, Shan Z, Teng X, et al. Effect of iodine intake on thyroid diseases in China. N Engl J Med 2006;354:2783-93. [PubMed]
- Burek CL, Rose NR. Autoimmune thyroiditis and ROS. Autoimmun Rev 2008;7:530-7. [PubMed]
- Papanastasiou L, Vatalas IA, Koutras DA, et al. Thyroid autoimmunity in the current iodine environment. Thyroid 2007;17:729-39. [PubMed]
- Villanueva R, Greenberg DA, Davies TF, et al. Sibling recurrence risk in autoimmune thyroid disease. Thyroid 2003;13:761-4. [PubMed]
- Dittmar M, Libich C, Brenzel T, et al. Increased familial clustering of autoimmune thyroid diseases. Horm Metab Res 2011;43:200-4. [PubMed]
- Braverman LE, Cooper D. Werner & Ingbar's The Thyroid: a fundamental and clinical text, 10e. Philadelphia: Lippincott Williams & Wilkins, 2012.
- Gopalakrishnan S, Marwaha RK. Juvenile autoimmune thyroiditis. J Pediatr Endocrinol Metab 2007;20:961-70. [PubMed]
- Wasniewska M, Salerno M, Cassio A, et al. Prospective evaluation of the natural course of idiopathic subclinical hypothyroidism in childhood and adolescence. Eur J Endocrinol 2009;160:417-21. [PubMed]
- Yeşilkaya E, Koç A, Bideci A, et al. CTLA4 gene polymorphisms in children and adolescents with autoimmune thyroid diseases. Genet Test 2008;12:461-4. [PubMed]
- Swain M, Swain T, Mohanty BK. Autoimmune thyroid disorders-An update. Indian J Clin Biochem 2005;20:9-17. [PubMed]
- Gopalakrishnan S, Chugh PK, Chhillar M, et al. Goitrous autoimmune thyroiditis in a pediatric population: a longitudinal study. Pediatrics 2008;122:e670-4. [PubMed]
- Rallison ML, Dobyns BM, Keating FR, et al. Occurrence and natural history of chronic lymphocytic thyroiditis in childhood. J Pediatr 1975;86:675-82. [PubMed]
- Ikemoto K. A long-term follow-up study of patients with goitrous Hashimoto's disease--prognosis and its predictive factors. Nihon Naibunpi Gakkai Zasshi 1990;66:619-35. [PubMed]
- Matsuda A, Kosugi S. A homozygous missense mutation of the sodium/iodide symporter gene causing iodide transport defect. J Clin Endocrinol Metab 1997;82:3966-71. [PubMed]
- Dai G, Levy O, Carrasco N. Cloning and characterization of the thyroid iodide transporter. Nature 1996;379:458-60. [PubMed]
- Kimura S, Hong YS, Kotani T, et al. Structure of the human thyroid peroxidase gene: comparison and relationship to the human myeloperoxidase gene. Biochemistry 1989;28:4481-9. [PubMed]
- De Deken X, Wang D, Dumont JE, et al. Characterization of ThOX proteins as components of the thyroid H(2)O(2)-generating system. Exp Cell Res 2002;273:187-96. [PubMed]
- Guria S, Bankura B, Balmiki N, et al. Functional analysis of thyroid peroxidase gene mutations detected in patients with thyroid dyshormonogenesis. Int J Endocrinol 2014;2014:390121.
- Popławska-Kita A, Telejko B, Siewko K, et al. Decreased Expression of Thyroglobulin and Sodium Iodide Symporter Genes in Hashimoto's Thyroiditis. Int J Endocrinol 2014;2014:690704.
- Balmiki N, Bankura B, Guria S, et al. Genetic analysis of thyroid peroxidase (TPO) gene in patients whose hypothyroidism was found in adulthood in West Bengal, India. Endocr J 2014;61:289-96. [PubMed]
- Spitzweg C, Morris JC. Genetics and phenomics of hypothyroidism and goiter due to NIS mutations. Mol Cell Endocrinol 2010;322:56-63. [PubMed]
- Fugazzola L, Muzza M, Weber G, et al. DUOXS defects: Genotype-phenotype correlations. Ann Endocrinol (Paris) 2011;72:82-6. [PubMed]
- Bicknell AA, Cenik C, Chua HN, et al. Introns in UTRs: why we should stop ignoring them. Bioessays 2012;34:1025-34. [PubMed]
- Cenik C, Chua HN, Zhang H, et al. Genome analysis reveals interplay between 5'UTR introns and nuclear mRNA export for secretory and mitochondrial genes. PLoS Genet 2011;7:e1001366. [PubMed]
- Rearick D, Prakash A, McSweeny A, et al. Critical association of ncRNA with introns. Nucleic Acids Res 2011;39:2357-66. [PubMed]
- Grasberger H, Refetoff S. Genetic causes of congenital hypothyroidism due to dyshormonogenesis. Curr Opin Pediatr 2011;23:421-8. [PubMed]