ATF3: a novel biomarker for the diagnosis of acute kidney injury after cardiac surgery
Introduction
Acute kidney injury (AKI), caused by hypoperfusion, ischemia-reperfusion injury, neurohumoral activation, inflammation, oxidative stress, refers to a sudden decrease in renal function, which is the most common non-cardiac complication after cardiopulmonary bypass (CPB) surgery, with an incidence ranging from 30% to 59% (1-10). Studies in the past decades have found that despite the continuous development of CPB techniques, cardiac surgery techniques, and postoperative monitoring techniques, the incidence of AKI is still high (2,6). AKI after cardiac surgery not only affects the prognosis of patients, but also increases the mortality of patients after hospitalization and discharge (3,4,8). Most researchers have used the Acute Kidney Injury Network (AKIN) and/or the Risk, Injury, Failure, Loss, End Stage Kidney Disease (RIFLE) criteria to detect AKI. These criteria are based on serum creatinine (sCr) levels (increased by at least 1.5 times baseline) and urine output (<0.5 mL/kg/h for at least 6 hours) (10). However, AKI is still underdiagnosed, because of the lack of accurate biomarkers for distinguishing high-risk patients and for the early diagnosis of AKI.
In order to better estimate the extent of injury and to enable early diagnosis, differential diagnosis, and prognostic prediction of AKI, the international KDIGO committee requested the identification of markers for early AKI (11). ATF3 is a member of the ATF/CREB subfamily of the basic leucine zipper (BZIP) family and is expressed in low concentrations in most normal cells. It is not only a key regulatory factor in stress response, but also extensively participates in pathological and physiological processes such as maintenance of body homeostasis, wound healing, cell adhesion, and tumor formation. Previous studies have shown that ATF3 can be detected in urine within 2–24 h in the ischemia-reperfusion injury-induced AKI rat model (12). Panich et al. found that urinary ATF3 (uATF3) also showed similar performance in sepsis-induced AKI patients (13). Due to its early expression and the absence of ATF3 in the whole urine of healthy people, it is a promising biomarker for the early diagnosis of AKI (12,13).
However, ATF3 has not been studied in patients undergoing CPB cardiac surgery. In our study, for the first time, patients undergoing CPB surgery were used as the research object, and we retrospectively observed the expression of ATF3 in the blood and urine of patients during the perioperative period, and further analyzed whether ATF3 can be used as an early biomarker of AKI. We present the following article in accordance with the STARD reporting checklist (available at https://dx.doi.org/10.21037/atm-21-5231).
Methods
The purpose of this study was to investigate whether ATF3 can be used as a marker for the early diagnosis of AKI after CPB. These studies were registered in the Chinese Clinical Trial Registry (Identifier: ChiCTR2000030869). This study was approved by the Ethics Committee of Shanghai Tenth People’s Hospital (No. SHSY-IEC-KY-4.0/16-31/01), and conducted in accordance with the Helsinki Declaration (as revised in 2013). Informed consent was taken from all the patients.
All patients included in this study, aged from 18 to 85 years old, were scheduled for elective CPB (ECB) surgery. All of them volunteered to participate in the study and provided informed consent. All patients were mentally conscious and capable of independent behavior. The exclusion criteria were as follows: patients who were expected to be hospitalized for less than 3 days; patients with a history of chronic kidney disease preoperatively; patients with preoperative critical vital signs; patients with urinary tract infection within 3 days preoperatively; patients in critical condition postoperatively.
Clinical information collection
The preoperative, intraoperative, and postoperative information of patients was collected, as detailed in Table 1.
Table 1
Variables | Total | Non-AKI group | AKI group | P |
---|---|---|---|---|
AKI, n | 83 | 41 | 42 | 1.000 |
Characteristics | ||||
Male, n (%) | 48 (57.83) | 28 (68.29) | 20 (47.62) | 0.057 |
Age (year) | 60.47±10.47 | 58.12±10.36 | 62.76±10.18 | 0.023 |
Height (cm) | 164.06±7.74 | 166.61±7.70 | 161.57±7.01 | 0.003 |
Body weight (kg) | 65.90±11.95 | 67.74±11.82 | 64.14±11.95 | 0.084 |
Hypertension, n (%) | 40 (48.78) | 8 (19.51) | 7 (16.67) | 0.736 |
Diabetes mellitus, n (%) | 15 (18.07) | 19 (46.34) | 21 (51.22) | 0.659 |
LVEF (%) | 58.55±8.39 | 58.63±8.38 | 58.48±8.50 | 0.708 |
Preoperative examination | ||||
WBC (×109/L) | 6.39±1.89 | 6.65±2.02 | 6.13±1.74 | 0.212 |
RBC (×1012/L) | 4.41±0.54 | 4.47±0.44 | 4.35±0.62 | 0.360 |
Hb (g/L) | 143.31±111.97 | 159.24±157.64 | 127.76±18.12 | 0.035 |
PLT (×109/L) | 210.06±120.51 | 232.20±160.52 | 188.45±54.19 | 0.104 |
ALT (U/L) | 28.01±18.87 | 31.84±21.34 | 24.19±15.35 | 0.041 |
AST (U/L) | 30.31±20.94 | 30.23±21.20 | 30.40±20.94 | 0.878 |
ALB (g/L) | 41.52±4.29 | 41.78±4.18 | 41.27±4.43 | 0.592 |
Conjugated bilirubin (μmol/L) | 5.66±3.56 | 5.61±3.66 | 5.71±3.50 | 0.760 |
Unconjugated bilirubin (μmol/L) | 8.18±6.87 | 8.14±6.73 | 8.24±7.10 | 0.914 |
Creatinine (μmol/L) | 75.74±17.79 | 76.43±18.07 | 75.07±17.71 | 0.731 |
Uric acid (μmol/L) | 354.26±99.90 | 348.12±106.34 | 360.71±93.60 | 0.576 |
Urea nitrogen (mmol/L) | 5.65±1.60 | 5.35±1.59 | 5.95±1.58 | 0.044 |
Surgical information, n (%) | ||||
Coronary artery bypass grafting | 34 (40.96) | 21 (51.22) | 13 (30.95) | 0.061 |
Coronary artery bypass grafting + valve surgery | 3 (3.61) | 0 (0.00) | 3 (7.14) | 0.248 |
Single valve replacement | 29 (34.94) | 14 (34.15) | 15 (35.71) | 0.938 |
Dual valve replacement | 10 (12.05) | 3 (7.32) | 7 (16.67) | 0.332 |
Major vascular surgery | 2 (2.41) | 0 (0.00) | 2 (4.76) | 0.494 |
Others (surgery for atrial septal defect, surgery for tumors, ablation for atrial fibrillation, etc.) | 5 (6.02) | 2 (4.88) | 3 (7.14) | 1.000 |
CPB | ||||
Total time (min) | 97.60±34.26 | 92.88±27.18 | 102.21±39.78 | 0.607 |
Cardiac arrest, n (%) | 48 (57.83) | 21 (51.22) | 27 (64.29) | 0.228 |
Time of clamping (min) | 37.18±35.96 | 30.66±32.68 | 43.55±38.22 | 0.119 |
Intraoperative RBC (U) | 1.06±1.80 | 0.78±1.39 | 1.33±2.10 | 0.184 |
Intraoperative plasma (mL) | 189.23±256.57 | 158.54±239.77 | 219.19±271.50 | 0.217 |
Intraoperative PLT, n (%) | 2 (2.41) | 0 (0.00) | 2 (4.76) | 0.494 |
ICU | ||||
ICU stay (h) | 74.78±65.07 | 71.78±57.32 | 77.71±72.42 | 0.196 |
Invasive ventilation (h) | 27.64±58.61 | 27.17±43.59 | 28.10±70.82 | 0.371 |
Complications, n (%) | ||||
Lung infection | 4 (4.82) | 2 (4.88) | 2 (4.76) | 1.000 |
Hypoxemia | 15 (18.07) | 7 (17.07) | 8 (19.05) | 0.815 |
Low cardiac output | 10 (12.05) | 8 (19.51) | 2 (4.76) | 0.084 |
Mediastinal hemorrhage | 4 (4.82) | 1 (2.44) | 3 (7.14) | 0.626 |
Atrial fibrillation | 18 (21.69) | 6 (14.63) | 12 (28.57) | 0.124 |
Delirium | 1 (1.2) | 0 (0.00) | 1 (2.38) | 1.000 |
Septicemia | 1 (1.2) | 1 (2.44) | 0 (0.00) | 1.000 |
Death | 3 (3.61) | 2 (4.88) | 1 (2.38) | 1.000 |
CRRT | 2 (2.41) | 1 (2.38) | 1 (2.38) | 1.000 |
Data were expressed as or n (%). AKI, acute kidney injury; LVEF, left ventricular ejection fraction; WBC, white blood cell; RBC, red blood cell; Hb, hemoglobin; PLT, platelet; ALT, alanine transaminase; AST, aspartate transaminase; ALB, albumin; CPB, cardiopulmonary bypass; ICU, intensive care unit; CRRT, continuous renal replacement therapy.
Blood and urine samples (3 mL) were collected 1 day before surgery (T0) and 2 h (T1), 6 h (T2), 12 h (T3), 24 h (T4), and 48 h (T5) after surgery. After all samples were collected, the supernatant was centrifuged and stored in an Eppendorf tube and a refrigerator at −80 °C. We used an ELISA kit to detect sCr (Jiancheng, Nanjing) and ATF3 (96 Tests, CloudClone).
Grouping
Patients were divided into the AKI group and non-AKI group according to the KDIGO AKI definition. AKI was defined as a rapid decline in renal function within 48 h after surgery, represented by an absolute increase in sCr ≥26.5 µmol/L (0.3 mg/dL), or an increase ≥1.5 times the baseline, or urine volume less than 0.5 mL/kg/h for 6 h. Patients’ basal creatinine was defined as the sCr level at admission.
Statistical analysis
SPSS 19.0 software was used for statistical analysis. Normally distributed data were expressed as , and the t-test was used for comparisons between groups. Non-normally distributed data were represented by the median (upper digit to lower digit), and comparisons between groups were tested with the Wilcoxon test. Count data were analyzed using the chi-square test or Fisher’s exact probability. Receiver operating characteristic (ROC) curves and the area under the ROC curve (AUC) were used to evaluate the accuracy of ATF3 in the diagnosis of AKI. At the same time, linear regression analysis was used to analyze the influence of various preoperative factors on the baseline expression of ATF3 in blood and urine, and univariate linear regression analysis was performed.
Results
Basic data of patients
A total of 83 surgical patients were included in the study (Table 1). The mean age was 60.47±10.47 years old, and male patients accounted for 57.83% (48/83) of the total population. Preoperative hypertension was associated with 40 cases (48.78%). The preoperative left ventricular ejection fraction (LVEF) was 58.55±8.39 mL, and the preoperative creatinine was 75.74±17.79 µmol/L.
Among the subjects, 34 underwent coronary artery bypass grafting, 3 underwent coronary artery bypass plus valve surgery, 39 underwent valve surgery (single and double valve replacement), 2 underwent major vessel surgery, and 5 underwent other procedures (for atrial fibrillation ablation, atrial tumor, atrial septal defect repair, among others). All patients were successfully operated on and admitted to the cardiac care unit for perioperative treatment.
AKI occurred in 42 patients, accounting for 50.6% of the total population. Age and preoperative urea nitrogen levels in the AKI group were higher than those in the non-AKI group, while height, preoperative hemoglobin (Hb), and ALT were lower than those in the non-AKI group. Moreover, postoperative platelet (PLT) levels and urea nitrogen levels in patients with AKI were lower than those in patients without AKI. The statistical analysis indicated that surgery and CPB had little influence on the occurrence of AKI. There were no significant differences in ICU time, mechanical ventilation time, and complications between the two groups.
The expression of ATF3 in serum and urine
As shown in Table 2, there was no difference in blood ATF3 level between the two groups before surgery. The level of serum ATF3 (sATF3) in the AKI group was significantly higher than that in the non-AKI group 24 h after surgery (T4), and the difference was statistically significant (662.62±204.72 vs. 586.93±175.87; P=0.035). Unlike the insensitivity of sATF3 level in the two groups, there was a statistical difference in uATF3 level 6 h after surgery (T2). The uATF3 level in the AKI group was significantly higher than that in the non-AKI group (974.92±341.42 vs. 1,169.90±280.93; P=0.014). The difference remained until 12 h after surgery (T3) (993.23±291.16 vs. 1,190.05±309.58; P=0.002). uATF3 level decreased 24 h after surgery (T4) in the AKI group, but the difference was not significant compared with that in the non-AKI group.
Table 2
Time | T0 | T1 | T2 | T3 | T4 | T5 |
---|---|---|---|---|---|---|
sATF3 | ||||||
Non-AKI | 530.12 (155.97) | 628.12 (202.44) | 710.87 (197.43) | 753.97 (216.57) | 586.93 (175.87) | 503.29 (146.32) |
AKI | 489.18 (134.00) | 596.67 (159.23) | 759.86 (188.87) | 774.96 (203.22) | 662.62 (204.72) | 518.22 (130.62) |
t value | 0.94 | 0.60 | −1.47 | −0.92 | −2.15 | −0.54 |
P value | 0.352 | 0.548 | 0.146 | 0.361 | 0.035 | 0.594 |
uATF3 | ||||||
Non-AKI | 746.99 (255.36) | 868.98 (212.99) | 974.92 (341.42) | 993.23 (291.16) | 839.52 (308.14) | 728.70 (212.39) |
AKI | 733.30 (175.79) | 924.74 (217.65) | 1,169.90 (280.93) | 1,190.05 (309.58) | 924.15 (253.07) | 780.55 (277.70) |
t value | 0.29 | −0.95 | −2.53 | −3.14 | −1.52 | −1.07 |
P value | 0.769 | 0.346 | 0.014 | 0.002 | 0.132 | 0.290 |
Data were expressed as n (%). sATF3, serum ATF3; AKI, acute kidney injury; uATF3, urinary ATF3.
Verification of the accuracy of ATF3 in the diagnosis of AKI
Subsequently, we performed ROC analysis of ATF3 in blood and urine at each time point after surgery (Table 3 and Figure 1). The AUC of ATF3 at 24 h after surgery (T4) was 0.647 (95% CI: 0.523–0.771). The sensitivity and specificity were 0.43 and 0.80, respectively, when sATF3 level was higher than 643 pg/mL. The AUC of uATF3 was 0.691 (95% CI: 0.576–0.807) at 12 h postoperatively (T3). When uATF3 expression was higher than 1,216 pg/mL, the sensitivity and specificity of AKI were 0.43 and 0.85, respectively. The diagnosis time of AKI in urine was earlier than that in blood.
Table 3
Variables | Cut-off | AUC (95% CI) | Sensitivity (95% CI) | Specificity (95% CI) |
---|---|---|---|---|
sATF3 | ||||
T1 | 549.00 | 0.522 (0.394–0.650) | 0.500 (0.349, 0.651) | 0.415 (0.264, 0.565) |
T2 | 680.64 | 0.623 (0.500–0.745) | 0.619 (0.472, 0.766) | 0.634 (0.487, 0.782) |
T3 | 840.24 | 0.571 (0.442–0.700) | 0.405 (0.256, 0.553) | 0.781 (0.654, 0.907) |
T4 | 643.00 | 0.647 (0.523–0.771) | 0.429 (0.279, 0.578) | 0.805 (0.684, 0.926) |
T5 | 513.00 | 0.567 (0.430–0.705) | 0.405 (0.256, 0.553) | 0.781 (0.654, 0.907) |
uATF3 | ||||
T1 | 843.00 | 0.548 (0.422–0.674) | 0.595 (0.447, 0.744) | 0.537 (0.384, 0.689) |
T2 | 1055.84 | 0.666 (0.545–0.787) | 0.619 (0.472, 0.766) | 0.707 (0.568, 0.847) |
T3 | 1216.00 | 0.691 (0.576–0.807) | 0.429 (0.279, 0.578) | 0.854 (0.746, 0.962) |
T4 | 770.02 | 0.616 (0.490–0.743) | 0.643 (0.498, 0.788) | 0.537 (0.384, 0.689) |
T5 | 865.48 | 0.574 (0.438–0.710) | 0.262 (0.129, 0.395) | 0.854 (0.746, 0.962) |
ROC, receiver operating characteristic; AKI, acute kidney injury; AUC, area under the ROC curve; sATF3, serum ATF3; uATF3, urinary ATF3.
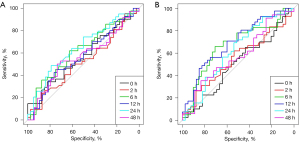
Analysis of factors influencing baseline ATF3 levels
Through linear regression analysis (Table 4), we found that preoperative ATF3 expression was not affected by age, gender, cardiac function, leucocyte level, and biochemical examination. The baseline urine ATF3 level was correlated with the preoperative creatinine and uric acid levels (P=0.012 and 0.036 for sATF3 and uATF3, respectively).
Table 4
Variables | sATF3 | uATF3 | |||
---|---|---|---|---|---|
t value | P value | t value | P value | ||
Age | 1.66 | 0.100 | 0.80 | 0.426 | |
T2DM | 0.63 | 0.528 | 1.84 | 0.069 | |
HBP | 1.12 | 0.266 | 0.21 | 0.831 | |
EF | −1.69 | 0.095 | −1.51 | 0.136 | |
Male | 1.51 | 0.136 | −0.48 | 0.631 | |
Preoperative WBC | 0.23 | 0.823 | −0.66 | 0.512 | |
Preoperative RBC | −0.06 | 0.955 | −1.39 | 0.167 | |
Preoperative Hb | −0.46 | 0.648 | 0.29 | 0.774 | |
Preoperative PLT | −0.14 | 0.889 | 0.66 | 0.514 | |
Preoperative ALT | −0.07 | 0.942 | 0.59 | 0.560 | |
Preoperative AST | 0.26 | 0.797 | 0.43 | 0.667 | |
Preoperative ALB | −0.51 | 0.610 | 0.07 | 0.947 | |
Preoperative conjugated bilirubin | 0.60 | 0.551 | −0.28 | 0.784 | |
Preoperative unconjugated bilirubin | −1.15 | 0.252 | −0.73 | 0.469 | |
Preoperative sCr | 1.15 | 0.254 | −2.59 | 0.012 | |
Preoperative uric acid | 1.14 | 0.259 | −2.14 | 0.036 | |
Preoperative BUN | −0.37 | 0.715 | −1.34 | 0.184 |
sATF3, serum ATF3; uATF3, urinary ATF3; T2DM, type 2 diabetes mellitus; HBP, high blood pressure; EF, ejection fraction; WBC, white blood cell; RBC, red blood cell; Hb, hemoglobin; PLT, platelet; ALT, alanine transaminase; AST, aspartate transaminase; ALB, albumin; sCr, serum creatinine; BUN, blood urea nitrogen.
Discussion
AKI is a common clinical complication, especially after CPB. The incidence can be as high as 60%, and the mortality rate of severe patients can reach or exceed 50% (1-5). Early diagnosis of AKI is the most effective treatment method. Although clinical practice guidelines for KDIGO provide clear consensus criteria for the early detection of AKI, AKI remains underdiagnosed (10). The main reason is that the existing diagnostic criteria are based on the changes in sCr, which only appear when renal function is impaired by more than 50% (12). Moreover, creatinine can be affected by a variety of external factors (14). In order to better estimate the extent of injury and the early diagnosis of AKI, new biomarkers need to be developed for clinical application.
Studies have shown that exosomes are monolayer membranous vesicle bodies released from various cells under different stress conditions, containing a variety of genetic materials and protein signaling molecules. Exosomes can not only serve as novel markers for the diagnosis and prognosis of AKI, but also play an important role in the occurrence and development of renal disease (15-19). ATF3 is one of these exosomes. It is a member of the ATF/CREB subfamily of the BZIP family and is expressed at low concentrations in most normal cells. The expression level of ATF3 in most normal resting cells is relatively low, but when the cells are stimulated by traumatic pressure signals inside and outside the body, the level of ATF3 mRNA rises significantly, which determines the cell’s need to stimulate the cell. Looking back on the existing evidence, it can be seen that in the animal model, these pressure signals mainly include: ischemia, ischemia reperfusion, trauma, axonal microsurgery, chemical drug toxicity, etc. (20). Studies have founded that ATF3 was expressed early in urine in a rat AKI model (12,21). Panich et al. found that uATF3 level can be used for early diagnosis of the occurrence of AKI in sepsis patients (13). The mechanisms of ATF3 in kidney protection may be as follows: (I) ATF3 up-regulates the expression of proangiogenic factors through protein translation, thereby promoting the proliferation of renal tubular epithelial cells and reducing cell apoptosis, thus achieving a protective effect on the kidney (21); (II) ATF3 can also mediate the expression of downstream monocyte chemoattractant protein-1 (MCP-1), thus playing a role in renal protection (21-23); (III) ATF3 directly interacts with histone deacetylase 1 (HDAC1) to form ATF3-related HDAC1 deacetylated histones, which interferes with NF-κB binding and inhibits inflammatory gene transcription, thereby protecting renal function (24).
Due to its early expression in AKI and its absence in the whole urine of healthy people, it may be used as a biological marker for the early diagnosis of AKI (12,13).
Our study found that the levels of ATF3 in blood and urine were increased at 2 h after CPB surgery, which was consistent with previous studies. On this basis, we further prospectively observed the dynamic expression of ATF3 in the blood and urine of perioperative AKI patients. Blood ATF3 levels were significantly increased 24 h after surgery compared with non-AKI patients. There were no significant differences at 2, 6, 12, and 48 h post operation. Moreover, there was a statistically significant difference in uATF3 6 h after surgery, which was significantly higher in the AKI group than in the non-AKI group (P=0.014), and the difference remained until 12 h after surgery. There was no significant difference in uATF3 48 h after surgery, which may be related to the recovery of renal function.
Further studies found that uATF3 levels were better than blood ATF3 levels in AKI diagnosis after operation. The AUC of uATF3 was 0.691 (95% CI: 0.576–0.807) at 12 h after surgery. In addition, our study also found that the preoperative basic conditions of patients, such as weight, gender, cardiac function, and preoperative blood examination, had no effects on the expression of ATF3 in blood, suggesting that the expression of ATF3 in blood was extremely stable. In urine, only the preoperative renal function status had an effect, suggesting that the expression of ATF3 in urine was closely related to the renal function status.
In conclusion, our research shows that ATF3, especially uATF3, can be used as a biomarker for early diagnosis of AKI after CPB surgery. Because, ATF3 can be expressed early in the blood and urine of AKI patients after CPB surgery, and it will increase at 2 hours after surgery and reach a peak at 12 hours after surgery (Table 2). Secondly, the expression of ATF3 is stable in the basal state. For example, the expression of ATF3 in blood is not affected by the patient’s basic conditions and is not affected by cardiac function. The expression of ATF3 in urine is more sensitive than ATF3 in blood and has a closer relationship with renal function. Third, through ROC analysis, the AUC for the diagnosis of AKI by the level of urine ATF3 at 12 hours after surgery was 0.691 (Figure 1). Therefore, we believe that ATF3, especially urine ATF3, can become a potential early biomarker of AKI.
Although the results of this study are encouraging, there are still many shortcomings. First, only 83 patients were included in this study, which is fairly small and might lead to the generation of test errors. It is therefore necessary to further increase the sample size to confirm the clinical significance of ATF3. Second, our study showed that urine ATF3 was only negatively affected by sCr, which may be due to the fact that urine ATF3 was significantly increased when sCr expression was not increased under stimulation, so the false impression of a negative correlation appeared. In summary, the sample size and even the number of healthy persons need to be increased in our future work.
Conclusions
The expression of ATF3 in urine is related to the renal function status of patients. Due to its good stability and early expression, ATF3 can be used as a candidate marker for the early diagnosis of AKI after cardiac surgery in adults.
Acknowledgments
Funding: This work was financially supported by Scientific research project of Shanghai Health and Family Planning Commission (No. 201740243).
Footnote
Reporting Checklist: The authors have completed the STARD reporting checklist. Available at https://dx.doi.org/10.21037/atm-21-5231
Data Sharing Statement: Available at https://dx.doi.org/10.21037/atm-21-5231
Conflicts of Interest: All authors have completed the ICMJE uniform disclosure form (available at https://dx.doi.org/10.21037/atm-21-5231). The authors have no conflicts of interest to declare.
Ethical Statement: The authors are accountable for all aspects of the work in ensuring that questions related to the accuracy or integrity of any part of the work are appropriately investigated and resolved. This study was approved by the Ethics Committee of Shanghai Tenth People’s Hospital (No. SHSY-IEC-KY-4.0/16-31/01), and conducted in accordance with the Helsinki Declaration (as revised in 2013). Informed consent was taken from all the patients.
Open Access Statement: This is an Open Access article distributed in accordance with the Creative Commons Attribution-NonCommercial-NoDerivs 4.0 International License (CC BY-NC-ND 4.0), which permits the non-commercial replication and distribution of the article with the strict proviso that no changes or edits are made and the original work is properly cited (including links to both the formal publication through the relevant DOI and the license). See: https://creativecommons.org/licenses/by-nc-nd/4.0/.
References
- Reardon MJ, Van Mieghem NM, Popma JJ, et al. Surgical or transcatheter aortic-valve replacement in intermediate-risk patients. N Engl J Med 2017;376:1321-31. [Crossref] [PubMed]
- Leon MB, Smith CR, Mack MJ, et al. Transcatheter or surgical aortic-valve replacement in intermediate-risk patients. N Engl J Med 2016;374:1609-20. [Crossref] [PubMed]
- Du S, Tian J, Xiao Z, et al. Serum alpha 1-antitrypsin predicts severe acute kidney injury after cardiac surgery. J Thorac Dis. 2019;11:5053-62. [Crossref] [PubMed]
- Hirano D, Ito A, Yamada A, et al. Independent risk factors and 2-year outcomes of acute kidney injury after surgery for congenital heart disease. Am J Nephrol 2017;46:204-9. [Crossref] [PubMed]
- Liu W, Xi Z, Gu C, et al. Impact of major bleeding on the risk of acute kidney injury in patients undergoing off-pump coronary artery bypass grafting. J Thorac Dis 2018;10:3381-9. [Crossref] [PubMed]
- Li S, Krawczeski CD, Zappitelli M, et al. Incidence, risk factors, and outcomes of acute kidney injury after pediatric cardiac surgery: a prospective multicenter study. Crit Care Med 2011;39:1493-9. [Crossref] [PubMed]
- Kwiatkowski DM, Price E, Axelrod DM, et al. Incidence, risk factors, and outcomes of acute kidney injury in adults undergoing surgery for congenital heart disease. Cardiol Young 2017;27:1068-75. [Crossref] [PubMed]
- Liang XL, Liu SX, Chen YH, et al. Combination of urinary kidney injury molecule-1 and interleukin-18 as early biomarker for the diagnosis and progressive assessment of acute kidney injury following cardiopulmonary bypass surgery: a prospective nested case-control study. Biomarkers 2010;15:332-9. [Crossref] [PubMed]
- Zarbock A, Kellum JA, Schmidt C, et al. Effect of early vs delayed initiation of renal replacement therapy on mortality in critically ill patients with acute kidney injury: the ELAIN randomized clinical trial. JAMA 2016;315:2190-9. [Crossref] [PubMed]
- Wang Y, Bellomo R. Cardiac surgery-associated acute kidney injury: risk factors, pathophysiology and treatment. Nat Rev Nephrol 2017;13:697-711. [Crossref] [PubMed]
- James M, Bouchard J, Ho J, et al. Canadian Society of Nephrology commentary on the 2012 KDIGO clinical practice guideline for acute kidney injury. Am J Kidney Dis 2013;61:673-85. [Crossref] [PubMed]
- Zhou H, Cheruvanky A, Hu X, et al. Urinary exosomal transcription factors, a new class of biomarkers for renal disease. Kidney Int 2008;74:613-21. [Crossref] [PubMed]
- Panich T, Chancharoenthana W, Somparn P, et al. Urinary exosomal activating transcriptional factor 3 as the early diagnostic biomarker for sepsis-induced acute kidney injury. BMC Nephrol 2017;18:10. [Crossref] [PubMed]
- Moran SM, Myers BD. Course of acute renal failure studied by a model of creatinine kinetics. Kidney Int 1985;27:928-37. [Crossref] [PubMed]
- Johnstone RM, Bianchini A, Teng K. Reticulocyte maturation and exosome release: transferrin receptor containing exosomes shows multiple plasma membrane functions. Blood 1989;74:1844-51. [Crossref] [PubMed]
- Stoorvogel W, Kleijmeer MJ, Geuze HJ, et al. The biogenesis and functions of exosomes. Traffic 2002;3:321-30. [Crossref] [PubMed]
- Dreyer F, Baur A. Biogenesis and functions of exosomes and extracellular vesicles. In: Federico M. editor. Lentiviral vectors and exosomes as gene and protein delivery tools. New York: Springer, 2016:201-16.
- Staals RH, Pruijn GJ. The human exosome and disease. Adv Exp Med Biol 2010;702:132-42. [Crossref] [PubMed]
- Bang C, Thum T. Exosomes: new players in cell-cell communication. Int J Biochem Cell Biol 2012;44:2060-4. [Crossref] [PubMed]
- Hai T, Wolfgang CD, Marsee DK, et al. ATF3 and stress responses. Gene Expr 1999;7:321-35. [PubMed]
- Chen HH, Lai PF, Lan YF, et al. Exosomal ATF3 RNA attenuates pro-inflammatory gene MCP-1 transcription in renal ischemia-reperfusion. J Cell Physiol 2014;229:1202-11. [Crossref] [PubMed]
- Thakar CV, Arrigain S, Worley S, et al. A clinical score to predict acute renal failure after cardiac surgery. J Am Soc Nephrol 2005;16:162-8. [Crossref] [PubMed]
- Mehta RH, Grab JD, O'Brien SM, et al. Bedside tool for predicting the risk of postoperative dialysis in patients undergoing cardiac surgery. Circulation 2006;114:2208-16; quiz 2208. [Crossref] [PubMed]
- Li HF, Cheng CF, Liao WJ, et al. ATF3-mediated epigenetic regulation protects against acute kidney injury. J Am Soc Nephrol 2010;21:1003-13. [Crossref] [PubMed]
(English Language Editor: C. Betlazar-Maseh)