N-glycosylation of somatostatin receptor type 2 protects rats from acute pancreatitis
Introduction
Acute pancreatitis is an inflammatory disorder, and is the most common gastrointestinal disease leading to hospital admission (1). The mortality rate of acute pancreatitis ranges from 2.22% to 45.63%, depending on the severity of the disease (2), while activation of the pancreatic enzymes in acinar cells leading to autodigestion of pancreatic tissue triggers the disease (3). The growth hormone inhibitor somatostatin (SST) is an endogenous peptide and known to regulate diverse cellular processes such as neurotransmission, hormone secretion and cell proliferation. Somatostatin and its analogs are promising therapeutic agents for acute pancreatitis through inhibiting pancreatic enzyme secretion (4) At the same time, SST can also reduce visceral blood flow and promote the recovery of gastrointestinal function. A variety of mechanisms work together to reduce the activities of trypsin and pepsin in SAP patients, and then reduce the damage and inflammatory reaction of pancreas and gastric tissue in SAP patients. However, a clinical trial including 302 patients with moderate to severe acute pancreatitis showed somatostatin had no effects on the mortality rate, rate of newly developed complications, duration of the pain, rate of surgical interventions, and length of the hospital stay (5). Thus, it is necessary to explore the factors that affect the therapeutic response to somatostatin in acute pancreatitis.
Somatostatin and its analogs exert their functions by binding to G-protein-coupled somatostatin receptors (SSTRs) that comprise five subtypes (SSTR1–5), among which SSTR2 is predominantly responsible for endogenous somatostatin-mediated regulation of hormone secretion (6). Clinically available somatostatin analogs preferentially binds to SSTR2 (7-9), and its expression positively correlates with the outcome of somatostatin or somatostatin analog treatment in acromegaly (7) and growth hormone-secreting pituitary adenomas (10). Moreover, due to the abundant SSTR2 expression in pancreatic inflammatory and tumor cells, somatostatin-related compounds achieve promising therapeutic effects against pancreatic disease at least partially through SSTR2-induced activation of the inhibitor κB kinase (IKK)/NF-κB signaling cascade (11-13). Considering SSTR2 belongs to the superfamily of G-protein-coupled receptors, we speculated that its stability and membrane trafficking might affect the response to somatostatin therapy in patients with acute pancreatitis. However, the regulatory mechanism of the stability and membrane trafficking of SSTR2 remains unclear. Most membrane proteins, including SSTRs, undergo N-glycosylation (14), and SSTR2 displays typical seven-transmembrane segments and contains four putative N-glycosylation sites (N9, N22, N29, and N32). In Western blot experiments, the SSTR2 protein band migrates between 70 and 80 kDa, suggesting SSTR2 protein may be unglycosylated or N-glycosylated at one or more asparagine (Asn) residues (15). N-glycans are involved in the folding, stability, trafficking, and localization of the proteins, and consequently modulate their functions (16). N-glycosylation also affects ligand binding, and mutation or truncation of its sites in SSTR3 results in significantly reduced binding to somatostatin and impaired signal transduction (17). Thus, we hypothesized that N-glycosylation of SSTR2 might affect its stability, membrane localization, and downstream signal transduction in pancreatic inflammation.
To test our hypothesis, we confirmed that endogenous SSTR2 is only glycosylated at N9 residue in AR42J cells, and the absence of N9 glycosylation blocks SSTR2 trafficking and membrane localization, then we examined the role of N-glycosylation of SSTR2 in cerulein plus lipopolysaccharide (LPS)-stimulated pancreatic acinar cells as well as in a pancreatitis mouse model. Our data suggest that the presence of N-glycan is required for the stability, membrane trafficking, and downstream signal transduction of SSTR2, protecting rats from cerulein plus LPS-induced pancreatic inflammation. We present the following article in accordance with the ARRIVE reporting checklist (available at https://dx.doi.org/10.21037/atm-21-5249).
Methods
Animals
The animal study was approved by the Chinese National Committee for the Use of Experimental Animals for Medical Purposes, Jiangsu Branch [JN.No20190930c0480120(233)], and all procedures were carried out following the Guide for the Care and Use of Laboratory Animals (National Research Council, 1996, USA). A total of 20 male Sprague-Dawley rats, 6–8 week-age, weighing 180–200 g were purchased from the Beijing Vital River Experimental Animals Technology Co., Ltd. [Beijing, China, SCXK (Jing) 2016-0006], and maintained under specific pathogen-free conditions.
Cell culture and treatment
Rat pancreatic acinar AR42J cells were obtained from the China Center for Type Culture Collection (Wuhan, China) and maintained at 37 °C in minimum essential medium (MEM) (Hyclone, Logan, UT, USA), which was supplemented with 10% fetal bovine serum (Hyclone), 100 U/mL penicillin and 100 µg/mL streptomycin, and the air contains 5% carbon dioxide. For cerulein plus LPS stimulation, AR42J cells were plated into 60-mm dishes and incubated for 24 h then stimulated with 10 nmol/L cerulein (Sigma-Aldrich, St. Louis, MO, USA) and 10 mg/L LPS (Sigma-Aldrich) for 0, 4, 8, 12, or 24 h (18) with phosphate-buffered saline (PBS) as a vehicle. Tunicamycin is a potent protein N-glycosylation inhibitor that has frequently been used to manipulate protein glycosylation in cells. To inhibit N-glycosylation, AR42J cells were cultured in medium containing 1 µg/mL tunicamycin (sigma-Aldrich) for 48 h, and for proteosome inhibition, cells were treated with 10 µM MG132 (Sigma-Aldrich) for 0, 2, or 4 h (19).
Vectors, site-directed mutagenesis, and transfection
A pcDNA3 plasmid overexpressing rat SSTR2 was purchased from the PPL company. To generate an asparagine-to-glutamine (N9Q) mutation in SSTR2 protein, a mutant SSTR2 plasmid was constructed by PCR-based mutagenesis using long-Taq-DNA polymerase (BIOSCI) and primers 5'-TGCTCTAGAATGGAGTTGACCTCTGAGCAGTTCAATCAAGGGAGC-3' (sense) and 5'-CCGGAATTCCAGATACTGGTTTGGAGGTT-3' (antisense). The plasmids were transiently transfected into AR42J cells using Lipofectamine 2000 (Invitrogen, Carlsbad, CA, USA) according to the manufacturer’s instructions.
Acute pancreatitis mouse model
Acute pancreatitis was induced in rats as previously described (3,20), and the experimental condition was as follows: the temperature was 22±2 °C; the humidity was 50%±5%; all mouse were fed with free drinking water and standard food; we maintained mouse circadian rhythm by alternating lighting. Briefly, rats were randomized to four groups: a pretreated with empty vectors (n=5) + PBS group, pretreated with empty vectors (n=5) + LPS group; vectors expressing wild-type SSTR2 (n=5) + LPS group, and vectors expressing N9Q-mutant SSTR2 (n=5) + LPS via caudal vein injection group. At 72 h after pretreatment, except for rats in the pretreated with empty vectors group, all others were subjected to 4 hourly intraperitoneal injections of cerulein (50 µg/kg) plus LPS (5 µg/mL) to induce acute pancreatitis. The control rats were administered the same volume of PBS at the same time points. Rats were sacrificed at several time intervals from 4 to 36 hours after the last intraperitoneal injection with cerulein and LPS.
Western blot analysis
Total proteins were obtained from AR42J cells using RIPA buffer, and to remove the N-glycan, 50 µg protein samples were incubated with 50 units of PNGase F (New England Biolabs, USA) at 37 °C for 4 h according to the manufacturer’s instruction. Peptide N-glycosidase F (PNGase F) is the most widely used enzyme for releasing N-glycans from glycoproteins in glycoanalytical workflows due to the broadest specificity and ability to liberate the most diverse range of N-glycan structures from glycoproteins. The protein samples were separated by SDS-polyacrylamide gel electrophoresis and transferred to a nitrocellulose membrane, and after blocking with 5% non-fat milk in Tris-buffered saline-Tween 20 (TBST) for 2 h at room temperature, the membrane was washed with TBST then incubated with primary antibody against SSTR2 (1:500), IκBα (1:500), p65 (1:500), p-p65 (1:1,000), or β-actin (1:1,000) at 4 °C overnight. All the above antibodies were purchased from Santa Cruz Biotechnology (Dallas, TX, USA). The membrane was then incubated with horseradish peroxidase conjugated secondary antibody (1:10,000; Santa Cruz Biotechnology) for 2 h at room temperature. The protein bands were detected using an enhanced chemiluminescence system (Thermo Fisher Scientific, Waltham, MA, USA), and their densities were quantified using Image J (NIH, MD, USA).
Immunofluorescence assay
At 8 h after cerulein plus LPS treatment, we fixed AR42J cells with 4% paraformaldehyde in PBS for 1 h at room temperature. then they were blocked with 10% donkey serum for 2 h at room temperature. Then we incubated the cells with primary antibody against myc (1:500; Santa Cruz Biotechnology), calnexin (1:500; Santa Cruz Biotechnology), or p65 (1:500; Santa Cruz Biotechnology) in 1% bovine serum albumin overnight at 4 °C, followed by incubation with Alexa Fluor 568- or Alexa Fluor 488-conjugated antibody (Invitrogen) for 2 h at room temperature. The nucleus was stained using 5 µg/mL Hoechst, and images were captured using a laser scanning confocal microscope (LSM710; Zeiss, Germany).
Liquid chromatography-electrospray ionization-mass spectrometry (LC-ESI-MS) analysis
To identify the N-glycosylation site of SSTR2, myc-his-SSTR2 proteins were purified using a Ni column and eluted with imidazole solution. The purified SSTR2 proteins then underwent PNGase F-mediated removal of N-glycan, dialysis on a C18 column, and trypsin digestion. The digested sample was analyzed using an LC-ESI-MS system consisting of an Aquasil C-18 precolumn (30 mm × 0.32 mm, 5 mm, Thermo Scientific), a BioBasic C18 analytical column (150 mm × 0.18 mm, 5 mm, Thermo Scientific), a Waters CapLC, a Rheodyne 10-port valve, and a Waters Q-TOF Ultima with standard ESI-source, as previously described (21). The mobile phase A was composed of 65 mM ammonium formate (pH 3.0), and phase B was composed of 80% acetonitrile in solvent A. The capillary voltage and cone voltage were 3.2 kV and 35 V, respectively, and the source temperature and desolvation temperature were 100 and 120 °C, respectively. Peptides were trapped in the precolumn before being separated on the analytical column at a flow rate of 5 µL/min.
Measurements of biochemical parameters
Rats were sacrificed at 4 to 36 h after cerulein plus LPS administration. Blood samples were immediately collected from the vena cava, and serum samples were obtained by centrifuging the blood samples at 3,500 rpm for 10 min. The serum amylase and lipase activities were measured using an amylase activity assay kit and lipase activity assay kit (Sigma-Aldrich), respectively, according to the manufacturer’s protocols. The serum concentrations of TNF-α, IL-6, and myeloperoxidase (MPO) were measured using corresponding ELISA kits (Excell, Shanghai, China) following the manufacturer’s instructions. All samples were analyzed three times using a microplate reader (BioRad, Hercules, CA, USA).
Haematoxylin & eosin (H&E) staining
Fresh rat pancreas specimens were fixed in 4% paraformaldehyde in PBS, embedded in paraffin, and cut into 5-mm-thick sections. H&E staining was performed following standard procedures, and the histological grading of edema, leukocyte infiltration, and vacuolization was conducted using a scale ranging from 0 to 3 as previously described (22).
Statistical analysis
Statistical analysis was performed using Stata 7.0 (StataCorp, College Station, TX, USA). Data are processed with mean ± standard error. The differences among groups were determined by one-way analysis of variance, followed by Tukey’s post hoc multiple comparison test, and a P value <0.05 was considered statistically significant. All experiments were performed in triplicate.
Results
SSTR2 is glycosylated at N9 residue in AR42J cells
To examine the glycosylation state of SSTR2 in AR42J cells, we treated them with the N-glycosylation inhibitor tunicamycin. Western blot analysis revealed that the SSTR2 protein band shifted downward due to the decreased molecular weight in response to tunicamycin treatment (Figure 1A), and digestion of the cell lysates with endoglycosidase PNGase F caused a similar band shift (Figure 1B). These results suggest that endogenous SSTR2 in AR42J cells is N-glycosylated.
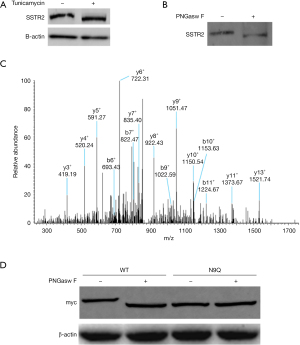
We then immunoprecipitated SSTR2 protein to identify the glycosylation sites, and the MS analysis showed that the N9 residue was a potential N-glycosylation site in SSTR2 (Figure 1C). Compared with the wild-type SSTR2 protein band, the N9Q-mutant SSTR2 protein band migrated downward but did not migrate further after PNGase F digestion (Figure 1D). These results suggest that endogenous SSTR2 is only glycosylated at N9 residue in AR42J cells.
N9 glycosylation is required for SSTR2 protein trafficking and stability
Since N-glycosylation contributes to protein trafficking, localization, and stability (23), we examined the subcellular localization and stability of overexpressed wild-type and N9Q-mutant SSTR2 proteins in AR42J cells. Immunofluorescent staining showed a widespread distribution of the wild-type SSTR2 protein in the plasma membrane and colocalization of N9Q-mutant SSTR2 protein with the endoplasmic reticulum (ER) marker calnexin (Figure 2A). This finding suggests that the absence of N9 glycosylation blocks SSTR2 trafficking and membrane localization. Furthermore, Western blot analysis revealed that AR42J cells transfected with wild-type SSTR2 plasmid expressed significantly higher levels of fusion proteins than those transfected with N9Q-mutant SSTR2 plasmid. Treatment with proteasome inhibitor MG132 rapidly restored the expression of N9Q-mutant SSTR2 protein in AR42J cells (Figure 2B), suggesting these proteins are more sensitive to proteasome-mediated degradation than wild-type SSTR2. Taken together, these results suggest that N9-glycosylation is required for SSTR2 protein stability and membrane localization in pancreatic cells.
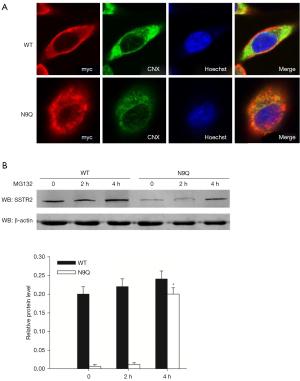
N9 glycosylation is required for SSTR2 to activate NF-κB signaling in inflammatory AR42J cells
In order to assess the contribution of N-glycosylation in SSTR2-mediated activation of NF-κB signaling, we provided AR42J cells with cerulein plus LPS to induce an inflammatory response. Western blot analysis demonstrated that cerulein plus LPS stimulation activated NFκB signaling in AR42J cells, as evidenced by a significant decline of NFκB suppressor IκBα (18) protein expression starting 8 h after treatment (Figure 3A). Compared with the control, overexpression of wild-type SSTR2 significantly attenuated protein expression of IκBα while enhancing protein expression of p-p65 in AR42J cells at 8 h after exposure to cerulein plus LPS, suggesting that overexpression of wild-type SSTR2 activates NF-κB signaling in AR42J cells upon cerulein plus LPS stimulation. On the other hand, overexpression of N9Q-mutant SSTR2 showed no effects on IκBα and p-p65 expression compared with the control (Figure 3B) and failed to facilitate nuclear transportation of p65 compared with wild-type SSTR2 (Figure 3C). These findings suggest N9 glycosylation is required for SSTR2 to activate NF-κB signaling in inflammatory AR42J cells.
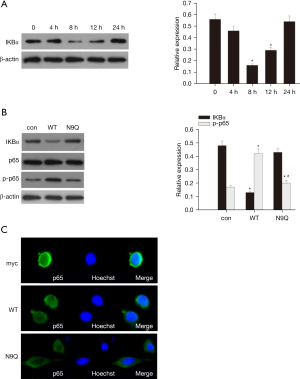
N-glycosylation of SSTR2 protects rats from acute pancreatitis
To explore the role of N-glycosylation of SSTR2 in acute pancreatitis in vivo, we pretreated the rats into 4 groups: control rats (n=5); empty vector (n=5); wild-type SSTR2-overexpressing vectors (n=5); and N9Q-mutant SSTR2-overexpressing vectors (n=5) before cerulein plus LPS administration As shown in Figure 4A-4D, compared with control rats, those treated with empty vector and cerulein plus LPS exhibited significantly increased serum levels of amylase, lipase, TNF-α, IL-6, and MPO, typical histopathological changes of acute pancreatitis, as well as considerably elevated H&E scores, suggesting cerulein plus LPS induces acute pancreatitis in the rats. Compared with empty vectors, pretreatment with wild-type SSTR2 partially, but significantly, reversed cerulein plus LPS-induced elevations in serum levels of biochemical parameters and histopathological changes in the pancreatic tissue. Pretreatment with mutant SSTR2-overexpressing vectors showed contrasting effects. These results suggest that N-glycosylation of SSTR2 ameliorates acute pancreatitis in vivo.
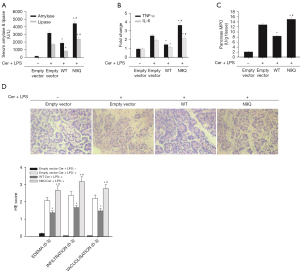
Discussion
Somatostatin and its analogs targeting SSTR2 are promising therapeutic agents for pancreatic inflammation and tumor treatment, at least partially via the NF-κB signaling pathway (13). N-glycosylation plays vital roles in protein maturation and secretion, subcellular trafficking, and signaling transduction of G protein-coupled receptors (24-26). Loss of N-glycans impairs the stability, membrane localization, ligand binding, and signal transduction of SSTRs and consequently affects the response to somatostatin therapy (17). In the present study, we found that endogenous SSTR2 protein was glycosylated at the N9 residue in rat pancreatic acinar AR42J cells. N9Q mutation in SSTR2 impaired the stability of SSTR2, retained the mutant protein in the ER, and blocked cerulein plus LPS-induced activation of NF-κB signaling. Importantly, overexpression of wild-type but not N9Q-mutant SSTR2 ameliorated cerulein plus LPS-induced pancreatic inflammation. Together, these findings suggest N9 glycosylation is required for the stability, membrane localization, and signal transduction of SSTR2 protein, protecting the pancreas from inflammatory stimuli.
Our results showed that AR42J cells transfected with wild-type SSTR2 plasmid expressed significantly higher levels of fusion proteins than cells transfected with mutant SSTR2 plasmid, suggesting that N9Q mutant fusion SSTR2 protein is considerably less stable than the wild-type protein in cells. The rapid restoration of mutant SSTR2 protein expression after MG132 treatment suggests that proteasomes are responsible for its degradation. Although protein misfolding resulting from amino acid mutation may cause rapid proteasomal degradation (27), our results suggest that loss of N-glycan mainly contributes to the degradation of mutant SSTR2 by proteasomes for the following reasons: (I) by treating AR42J cells with the N-glycosylation inhibitor tunicamycin, SSTR2 was indeed glycosylated, and trafficking of native SSTR2 isoforms beyond the ER was disrupted by inhibition of N-glycosylation. (II) Owing to the similarity of asparagine and glutamine in size and structure, glutamine has been frequently used for asparagine substitution mutation in the study of N-glycosylation in glycoproteins (28,29). The shift of the immunoblot bands of N9Q-mutant SSTR2 is likely due to the absence of large sugar moiety rather than the replacement of a single amino acid. (III) Glycoproteins with truncated N-glycans may fail to fold properly and retain in the cytoplasm where they are destroyed by proteasomal degradation (16).
NF-κB signaling plays an important role in immune responses, inflammation, and cellular stress, and SSTR2 has been shown to activate NF-κB signaling in pancreatic acinar AR42J cells and NIH3T3 cells (13,30). Here, we found that N9Q-mutant SSTR2 failed to activate NF-κB signaling in AR42J cells, suggesting that disruption of N-glycosylation of SSTR2 blocks the downstream signal transduction in pancreatic cells.
In conclusion, we demonstrated that N-glycosylation was essential for the stability, membrane localization, and signal transduction of SSTR2 in pancreatic cells. Loss of N-glycan disrupts the protective role of SSTR2 in acute pancreatitis. In the treatment of pancreatitis, we often use somatostatin, however, we are not completely clear about the therapeutic effect and the mechanism. But it is worth affirming that as the half-life of somatostatin is very short, it is not suitable for long-term treatment. On the other hand, somatostatin has a negative effect on the movement of gallbladder Oddi sphincter. Therefore, it has been suggested that doctors should not use secretory drugs to treat acute pancreatitis. Yet, the results from human studies of somatostatin used for therapeutic purposes are not conclusive. Our results provide new insights into the mechanism underlying the controversial therapeutic effects of somatostatin and its analogs in acute pancreatitis treatment.
Acknowledgments
The authors would like to thank all study participants who enrolled in this study.
Funding: This work was supported by the National Basic Research Program of China (973 Program, No. 2012CB822104), National Natural Science Foundation of China (31170766, 81470806), and Nantong City Social Development Projects funds (HS2012032).
Footnote
Reporting Checklist: The authors have completed the ARRIVE reporting checklist. Available at https://dx.doi.org/10.21037/atm-21-5249
Data Sharing Statement: Available at https://dx.doi.org/10.21037/atm-21-5249
Conflicts of Interest: All authors have completed the ICMJE uniform disclosure form (available at https://dx.doi.org/10.21037/atm-21-5249). The authors have no conflicts of interest to declare.
Ethical Statement: The authors are accountable for all aspects of the work in ensuring that questions related to the accuracy or integrity of any part of the work are appropriately investigated and resolved. The animal study was approved by the Chinese National Committee for the Use of Experimental Animals for Medical Purposes, Jiangsu Branch [JN.No20190930c0480120(233)], and all procedures were carried out following the Guide for the Care and Use of Laboratory Animals (National Research Council, 1996, USA).
Open Access Statement: This is an Open Access article distributed in accordance with the Creative Commons Attribution-NonCommercial-NoDerivs 4.0 International License (CC BY-NC-ND 4.0), which permits the non-commercial replication and distribution of the article with the strict proviso that no changes or edits are made and the original work is properly cited (including links to both the formal publication through the relevant DOI and the license). See: https://creativecommons.org/licenses/by-nc-nd/4.0/.
References
- Lankisch PG, Apte M, Banks PA. Acute pancreatitis. Lancet 2015;386:85-96. [Crossref] [PubMed]
- Popa CC, Badiu DC, Rusu OC, et al. Mortality prognostic factors in acute pancreatitis. J Med Life 2016;9:413-8. [PubMed]
- Wan H, Yuan Y, Liu J, et al. Pioglitazone, a PPAR-γ activator, attenuates the severity of cerulein-induced acute pancreatitis by modulating early growth response-1 transcription factor. Transl Res 2012;160:153-61. [Crossref] [PubMed]
- Li J, Wang R, Tang C. Somatostatin and octreotide on the treatment of acute pancreatitis - basic and clinical studies for three decades. Curr Pharm Des 2011;17:1594-601. [Crossref] [PubMed]
- Uhl W, Büchler MW, Malfertheiner P, et al. A randomised, double blind, multicentre trial of octreotide in moderate to severe acute pancreatitis. Gut 1999;45:97-104. [Crossref] [PubMed]
- Ben-Shlomo A, Melmed S. Pituitary somatostatin receptor signaling. Trends Endocrinol Metab 2010;21:123-33. [Crossref] [PubMed]
- Gatto F, Feelders RA, van der Pas R, et al. Immunoreactivity score using an anti-sst2A receptor monoclonal antibody strongly predicts the biochemical response to adjuvant treatment with somatostatin analogs in acromegaly. J Clin Endocrinol Metab 2013;98:E66-71. [Crossref] [PubMed]
- Taboada GF, Luque RM, Neto LV, et al. Quantitative analysis of somatostatin receptor subtypes (1-5) gene expression levels in somatotropinomas and correlation to in vivo hormonal and tumor volume responses to treatment with octreotide LAR. Eur J Endocrinol 2008;158:295-303. [Crossref] [PubMed]
- Olias G, Viollet C, Kusserow H, et al. Regulation and function of somatostatin receptors. J Neurochem 2004;89:1057-91. [Crossref] [PubMed]
- Franck SE, Gatto F, van der Lely AJ, et al. Somatostatin Receptor Expression in GH-Secreting Pituitary Adenomas Treated with Long-Acting Somatostatin Analogues in Combination with Pegvisomant. Neuroendocrinology 2017;105:44-53. [Crossref] [PubMed]
- Qian ZR, Li T, Ter-Minassian M, et al. Association Between Somatostatin Receptor Expression and Clinical Outcomes in Neuroendocrine Tumors. Pancreas 2016;45:1386-93. [Crossref] [PubMed]
- Kitagawa M, Naruse S, Ishiguro H, et al. Pharmaceutical development for treating pancreatic diseases. Pancreas 1998;16:427-31. [Crossref] [PubMed]
- Liu AM, Wong YH. Activation of nuclear factor {kappa}B by somatostatin type 2 receptor in pancreatic acinar AR42J cells involves G{alpha}14 and multiple signaling components: a mechanism requiring protein kinase C, calmodulin-dependent kinase II, ERK, and c-Src. J Biol Chem 2005;280:34617-25. [Crossref] [PubMed]
- Banerjee DK. N-glycans in cell survival and death: cross-talk between glycosyltransferases. Biochim Biophys Acta 2012;1820:1338-46. [Crossref] [PubMed]
- Günther T, Tulipano G, Dournaud P, et al. International Union of Basic and Clinical Pharmacology. CV. Somatostatin Receptors: Structure, Function, Ligands, and New Nomenclature. Pharmacol Rev 2018;70:763-835. [Crossref] [PubMed]
- Parodi A, Cummings RD, Aebi M. Glycans in Glycoprotein Quality Control. In: Varki A, Cummings RD, Esko JD, et al., editors. Essentials of Glycobiology. Cold Spring Harbor (NY), 2015:503-11.
- Nehring RB, Richter D, Meyerhof W. Glycosylation affects agonist binding and signal transduction of the rat somatostatin receptor subtype 3. J Physiol Paris 2000;94:185-92. [Crossref] [PubMed]
- Liu Y, Yang L, Chen KL, et al. Knockdown of GRP78 promotes apoptosis in pancreatic acinar cells and attenuates the severity of cerulein and LPS induced pancreatic inflammation. PLoS One 2014;9:e92389 [Crossref] [PubMed]
- Jin F, Xiao D, Zhao T, et al. Proteasome inhibitor MG132 suppresses pancreatic ductal adenocarcinoma-cell migration by increasing ESE3 expression. Oncol Lett 2020;19:858-68. [PubMed]
- Yuan J, Liu Y, Tan T, et al. Protein kinase d regulates cell death pathways in experimental pancreatitis. Front Physiol 2012;3:60. [Crossref] [PubMed]
- Stadlmann J, Pabst M, Kolarich D, et al. Analysis of immunoglobulin glycosylation by LC-ESI-MS of glycopeptides and oligosaccharides. Proteomics 2008;8:2858-71. [Crossref] [PubMed]
- Jaworek J, Bonio J, Leja-Szpa A, et al. Sensory nerves in central and peripheral control of pancreatic integrity by leptin and melatonin. J Physiol Pharmacol 2002;53:51-74. [PubMed]
- Lopez-Fernandez L, Roncero MI, Prieto A, et al. Comparative proteomic analyses reveal that Gnt2-mediated N-glycosylation affects cell wall glycans and protein content in Fusarium oxysporum. J Proteomics 2015;128:189-202. [Crossref] [PubMed]
- Wang T, Nakagawa S, Miyake T, et al. Identification and functional characterisation of N-linked glycosylation of the orphan G protein-coupled receptor Gpr176. Sci Rep 2020;10:4429. [Crossref] [PubMed]
- Gonzalez de Valdivia E, Sandén C, Kahn R, et al. Human G protein-coupled receptor 30 is N-glycosylated and N-terminal domain asparagine 44 is required for receptor structure and activity. Biosci Rep 2019;39:BSR20182436 [Crossref] [PubMed]
- Li X, Zhou M, Huang W, et al. N-glycosylation of the β2 adrenergic receptor regulates receptor function by modulating dimerization. FEBS J 2017;284:2004-18. [Crossref] [PubMed]
- Hernández-Ramírez LC, Martucci F, Morgan RM, et al. Rapid Proteasomal Degradation of Mutant Proteins Is the Primary Mechanism Leading to Tumorigenesis in Patients With Missense AIP Mutations. J Clin Endocrinol Metab 2016;101:3144-54. [Crossref] [PubMed]
- Jia H, Guo Y, Song X, et al. Elimination of N-glycosylation by site mutation further prolongs the half-life of IFN-α/Fc fusion proteins expressed in Pichia pastoris. Microb Cell Fact 2016;15:209. [Crossref] [PubMed]
- Vallbracht M, Rehwaldt S, Klupp BG, et al. Functional Role of N-Linked Glycosylation in Pseudorabies Virus Glycoprotein gH. J Virol 2018;92:e00084-18. [Crossref] [PubMed]
- Guillermet-Guibert J, Saint-Laurent N, Davenne L, et al. Novel synergistic mechanism for sst2 somatostatin and TNFalpha receptors to induce apoptosis: crosstalk between NF-kappaB and JNK pathways. Cell Death Differ 2007;14:197-208. [Crossref] [PubMed]
(English Language Editor: B. Draper)