Reciprocal induction of hepatitis C virus replication and stimulation of hepatic profibrogenic cytokine release and cellular viability by YKL-40
Introduction
Hepatitis C virus (HCV) infection is prone to chronicity, and more than 80% of acute HCV infection develops to chronic infection. The possible causes of chronic infection mainly include the following: high variability of HCV, pantropic virus to extrahepatic cells, as well as low levels of blood titer and immunogenicity, which result in continuous HCV infection (1). In recent years, direct-acting antivirals (DAAs) that target HCV proteins have been developed for HCV-related chronic hepatitis (2). However, because of its insidious onset, HCV infection is still one of the leading causes of chronic hepatitis, finally resulting in the development of hepatic fibrosis, cirrhosis, and hepatocellular carcinoma (HCC) (3-5).
HCV enhances the progression of hepatic fibrosis and cirrhosis through various molecular pathways. It regulates the extracellular matrix (ECM) and cellular adhesion genes during chronic infection, including remodeling enzymes [matrix metalloproteinases (MMPs)], cytokines, chemokines, the transforming growth factor (TGF)-β superfamily, vascular endothelial growth factors (VEGF), and genes involved in signal transduction, which finally induce progression of hepatic fibrosis (6). HCV induces the generation of reactive oxygen species (ROS) and subsequently activates the phosphorylation of p38 mitogen-activated protein kinase (MAPK), c-Jun N-terminal kinase (JNK), extracellular regulated protein kinases (ERK), and nuclear factor (NF)-κB, finally contributing to the induction of TGF-β (7). Hepatic stellate cells (HSCs) are widely considered as the most relevant source of hepatic myofibroblasts (8). However, HSCs are unable to infect Japanese fulminant hepatitis 1 (JFH1) supernatant (9). Thus, the question led to us to explore further the precise role of HSCs in HCV-related fibrosis.
YKL-40/chitinase 3-like protein 1 is a 40-kD secreted glycoprotein that is increased in several carcinoma and in non-neoplastic states, including chronic inflammation and tissue remodeling. The pro-inflammatory cytokines, including interleukin (IL)-1, tumor necrosis factor (TNF)-α, and IL-6, modulate YKL-40 expression in chondrocytes, macrophages, and glioblastoma multiforme cells. Additionally, YKL-40 is expressed during tissue development (e.g., in cartilage and in astrocytes) (10,11). Although the role of YKL-40 is not fully understood, serum YKL-40 levels have been confirmed to correlate with the severity of fibrosis and with its progression over time in patients with chronic hepatitis C (CHC) (12-14). The strong correlation between serum YKL-40 levels and hepatic mRNA levels in patients with CHC demonstrates that serum YKL-40 levels represent ongoing hepatic fibrogenesis. Additionally, serial measurement of serum YKL-40 levels can differentiate patients with slow from those with rapid progression of hepatic fibrosis (15-18). Serum levels of YKL-40 seem to predict antiviral treatment success in the natural setting of HCV-infection. Furthermore, previous studies indicated that serum levels of YKL-40 and hyaluronic acid (HA) as noninvasive markers of liver fibrosis in kidney transplant patients and haemodialysis patients with CHC (19,20). Serum YKL-40 measured at 3-8 months after liver transplantation, can accurately identify HCV-infected liver transplant recipients with rapid fibrosis progression (21,22). Additionally, YKL-40-gene polymorphism involves in acute cellular rejection and fibrosis progression after liver transplantation with HCV infection. A nucleotide substitution in YKL-40-gene (rs4950928; chromosome 1; G->C) induced higher YKL-40-levels in serum and more severe fibrosis in the presence of C-allele in HCV infection (23,24). A previous study reported that HCV modulates YKL-40 through the NOTCH signaling pathway (25). However, an infectious cell culture model of HCV was never used, and direct evidence of the effect of HCV on YKL-40 expression is needed. Therefore, we investigated the direct effect of HCV on YKL-40 expression in both hepatic parenchymal cells and HSCs and elucidated the precise mechanisms underlying YKL-40-mediated pathogenesis in the liver.
Here, we sought to determine whether HCV regulates YKL-40 expression and to elucidate the mechanisms by which YKL-40 mediates the liver fibrosis caused by HCV infection. In addition, we explored the expression of YKL-40 in hepatocytes and hepatic stellate cells, and the effect of these cells on YKL-40 production. We present the following article in accordance with the MDAR reporting checklist (available at https://dx.doi.org/10.21037/atm-21-4537).
Methods
Cell lines and culture
Huh7.5.1 cells (human HCC) infected or uninfected with HCV-JFH1, and HSCs were maintained in Ultra Culture serum-free medium (Lonza, NY, USA) supplemented with 1% L-glutamine and 1% penicillin–streptomycin (Gibco, NY, USA). The JFH1-infected cells from days 3–30 post-infection were used in this study. These cells were cultured in a humidified incubator at 37 °C with 5% CO2.
Primers, antibodies, reagents, and small interfering RNAs (siRNAs)
All primers, antibodies, reagents and siRNAs used in this study are listed in Tables 1-4.
Table 1
Primers | Sense | Antisense |
---|---|---|
YKL-40 | TGATGTGACGCTCTACGGC | AATGGCGGTACTGACTTGATG |
HCV RNA | TCTGCGGAACCGGTGAGTA | TCAGGCAGTACCACAAGGC |
TGF-β1 | CAACAATTCCTGGCGATACCT | GCTAAGGCGAAAGCCCTCAAT |
VEGF-A | CGAAACCATGAACTTTCTGC | CCTCAGTGGGCACACACTCC |
MMP3 | CTGGACTCCGACACTCTGGA | CAGGAAAGGTTCTGAAGTGACC |
Table 2
Antibodies | Dilution | Agent |
---|---|---|
Rabbit anti-NS5A | 0.111111111 | ViroGen, Watertown, MA, USA |
Rabbit anti-YKL-40 | 0.736111111 | Sigma, St. Louis, MO, USA |
Rabbit anti-TNFR1 | 0.736111111 | Sigma, St. Louis, MO, USA |
Rabbit anti-NF-κB p65 | 0.736111111 | Cell Signaling, MA, USA |
Rabbit anti-p38MAPK | 0.736111111 | Cell Signaling, MA, USA |
Rabbit anti-JNK | 0.736111111 | Cell Signaling, MA, USA |
Rabbit anti-p44/42 ERK | 0.736111111 | Cell Signaling, MA, USA |
Rabbit anti-β-actin | 1:10000 | Sigma, St. Louis, MO, USA |
Table 3
Reagents (EMD Chemicals, Inc., NJ, USA) | Target pathways | Concentration (µM) |
---|---|---|
DMSO | Control | 10 |
LY294002 | PI3K | 50 |
AG490 | JAK2 | 50 |
SP600125 | JNK | 40 |
PD98059 | MEK1 | 40 |
SB203580 | P-38-MAP | 40 |
DPI | ROS | 5 |
PDTC | NF-κB activation | 20 |
Table 4
siRNAs (Dharmacon, CO, USA) | Catalogue No. | Concentration (nM) |
---|---|---|
TNFR1 | L-005197-00-0005 | 50 |
p38 MAPK1 | L-003555-00-0005 | 50 |
JNK | L-003514-00-0005 | 50 |
p44-ERK1 | L-003592-00-0005 | 50 |
NF-κB3 (p65) | L-003533-00-0005 | 50 |
Plasmid and siRNA transfection
The plasmids used in this study were transfected using FuGENE6 transfection reagent (Roche Applied Science, IN, USA) as described before (3,4). The indicated siRNAs and negative siRNA (Cell Signaling, MA, USA) were transfected using HiPerFect Transfection Reagent (QIAGEN, CA, USA) following the manufacturer’s protocol (3,4).
Quantitative PCR
RNA was isolated using TRIzol (Life Technologies) following the manufacturer’s protocol. Next, it was reverse transcribed by random priming with the High Capacity cDNA Reverse Transcription Kit (Applied Biosystems, CA, USA). Quantitative real-time PCR using the DyNAmo HS SYBR Green qPCR kit (Finnzyme, Espoo, Finland) was performed on a BioRad S1000 Thermal Cycler PCR System. Human glyceraldehyde-3-phosphate dehydrogenase (GAPDH) was used as a control for basal RNA levels. The primers are listed in Tables 1-4.
Western blotting analysis
Cells were lysed using RIPA buffer containing 1% NP-40, 0.1% SDS, 10 mM Tris-HCl (pH 7.4), 1 mM EDTA, 150 mM NaCl and a protease inhibitor cocktail and then sonicated. Proteins were subjected to 10% SDS-PAGE separation and transferred to PVDF membranes. After blocking with 5% bovine serum albumin in Tris-buffered saline with 0.1% Tween 20 (TBST), the PVDF membrane was rinsed and incubated with the primary antibody listed in Tables 1-4. The secondary antibody was HRP-conjugated ECL donkey anti-rabbit IgG (Amersham Biosciences, NJ, USA). The Amersham ECL Western Blotting Detection Kit was used to detect chemiluminescent signals.
Cellular viability assay
In brief, cells were seeded at a density of 104 cells/well (100 μL of DMEM with 10% fetal bovine serum) and incubated for 72 h in a 96-well white plates. The Cell Titer-Glo Viability Assay Kit (Promega) was used following the manufacturer’s protocol.
Statistical analysis
All data are expressed as the mean ± SD from at least four sample replicates. Statistical analysis was executed by two-tailed Student’s t-test with pooled variance. P<0.05 and P<0.01 were considered statistically significant.
Results
HCV induced YKL-40 production in JFH1 cells
We hypothesized that HSCs are the main source of secreted YKL-40 due to its initial activated role during hepatic fibrogenesis. First, we examined YKL-40 expression in Huh7.5.1 and HSCs in vitro. Surprisingly, we found that YKL-40 expression was undetectable during Huh7.5.1 and HSCs differentiation and remained undetectable until 4 months had passed (passage 40) (data not shown). We then detected YKL-40 expression in both Huh7.5.1 and HSCs incubated with the JFH1 supernatant. Interesting, YKL-40 production gradually increased with prolongation of HCV infection at both the mRNA (P<0.05; Figure 1A) and protein levels (Figure 1B) in the cell lysate by 5.49-fold at 25 days post-infection (25 dpi) and relatively unchanged until 60 dpi (data not shown). In contrast, YKL-40 expression was still below the limits of detection in HSCs and HepG2 cells (control) incubated with the JFH1 supernatant (data not shown), neither of these cell types are able to be persistently and efficiently infected by HCV. These findings suggest that HCV induces YKL-40 expression in hepatic parenchymal cells during their development.
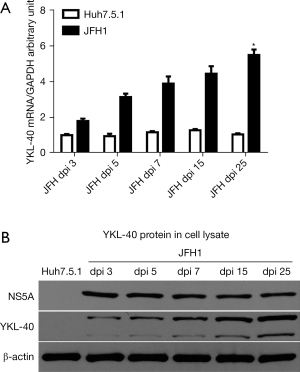
Effect of NF-κB on TNF-α-induced YKL-40 production in JFH1 cells
We next explored how HCV induces YKL-40 expression. Previous studies suggested that TNF-α induces YKL-40 expression in articular chondrocytes or airway epithelial cells (11,26), and it has been indicated that TNF-α is the most abundant cytokine secreted by HCV-infected cells (27,28). We, therefore, hypothesized that HCV induces YKL-40 expression in a TNF-α-dependent manner. Huh7.5.1 and JFH1 cells were stimulated with TNF-α (50 ng/mL) for 5, 14, 24, and 48 h before being harvested. We found that YKL-40 mRNA was detected early in Huh7.5.1 cells with a short 5 h exposure to TNF-α, indicating that a short stimulation of TNF-α is sufficient to induceYKL-40 mRNA. YKL-40 mRNA levels increased gradually in both the Huh7.5.1 and JFH1 cells with prolongation of the TNF-α incubation time (P<0.05; Figure 2A). Increased mRNA levels were followed by subsequent increases of the YKL-40 protein in the cell lysates (Figure 2B). However, YKL-40 mRNA levels seemed to reach a plateau with the extended TNF-α incubation of 72 h (data not shown), suggesting that TNF-α could be an initiative factor for HCV-induced YKL-40 production and that other special kinases control the cumulative activation of TNF-α. Interestingly, TNF-α was unable to induce YKL-40 production in HSCs (data not shown), demonstrating again that only hepatic parenchymal cells have the potential to produce the inflammatory cytokine YKL-40, which was consistent with our previous data.
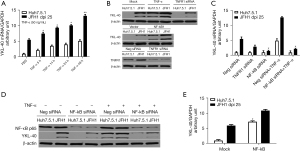
Furthermore, to identify whether HCV-induced YKL-40 generation was dependent on TNF-α, we used TNF-α receptor (TNF-R) siRNAs to knockdown the expression of TNF-R. We found that knockdown of TNF-R partially reduced YKL-40 mRNA (down to 50.2% of negative control siRNAs, P<0.05; Figure 2C) and protein levels (Figure 2B) in JFH1 cells, indicating that TNF-α partially accounted for the HCV induced-YKL-40 production. WB confirmed that TNF-R was knocked down by siRNAs (Figure 2B). The data demonstrated that TNF-α is sufficient but non-essential for HCV-induced YKL-40 production. Therefore, we speculated that there are other molecular processes controlling YKL-40 production during HCV infection.
We then asked why YKL-40 secretion hits a plateau with prolonged stimulation by TNF-α. A previous study had reported that short exposure of chondrocytes to TNF-α (1–4 h) resulted in persistent activation of NF-κB (11,29). We hypothesized that activated NF-κB caused by TNF-α was involved in YKL-40 expression during HCV infection. First, we observed that knockdown of NF-κB using siRNA nearly completely abolished YKL-40 mRNA transcript (P<0.05; Figure 2C), and protein levels (Figure 2D) in JFH1 cells in the presence or absence of TNF-α, respectively. Moreover, ectopic expression of NF-κB not only further enhanced YKL-40 expression in JFH1 cells but also induced YKL-40 mRNA (P<0.05; Figure 2E) and protein production (Figure 2B) in Huh7.5.1 cells. These findings suggested that NF-κB controlled the TNF-α-induced YKL-40 production in JFH1 cells.
Taken together, our findings indicated that HCV infection promoted TNF-α secretion, which in turn triggered the phosphorylation of NF-κB, and subsequently induced YKL-40 production.
Involvement and mechanism of MAPKs in HCV-induced YKL-40 production
Based on previous observations, we next explored whether there were other molecular processes involved in the activation of NF-κB that contributed to YKL-40 production during HCV infection. A previous study reported that HCV induced NF-κB activation through a ROS-MAPK (p38 MAPK, JNK, and ERK) pathway (7). We investigated whether ROS-MAPKs-NF-κB was involved in HCV-induced YKL-40 production. We monitored YKL-40 production in Huh7.5.1 or JFH1 cells treated with different inhibitors, and found that neither the PI3K inhibitor LY294002 nor the JAK2 inhibitor AG490 influenced HCV-induced enhancement of YKL-40 production. However, the ROS inhibitor DPI, the p38 MAPK inhibitor SB203580, the ERK inhibitor PD98059 and the JNK inhibitor SP600125 partially reduced the enhancement of YKL-40 mRNA and protein levels in JFH1 cells. Furthermore, the NF-κB inhibitor PDTC nearly completely eliminated HCV-induced YKL-40 production in JFH1 cells (P<0.05; Figure 3A). These findings suggested that ROS, MAPKs (p38 MAPK, ERK, and JNK), and NF-κB are all involved in HCV-induced YKL-40 production.
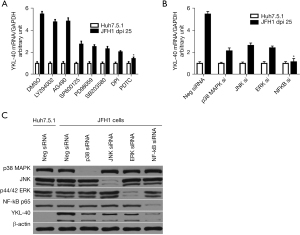
To further determine the specific effect of the previously indicated kinases on YKL-40 expression, we used siRNAs to knock them down. We found that the increase in YKL-40 mRNA levels was reduced by p38 MAPK siRNAs (down to 39.0%), ERK siRNAs (down to 44.6%), and JNK siRNAs (down to 48.3%) compared with the negative control siRNAs in JFH1 cells. NF-κB siRNAs nearly completely blocked HCV-induced YKL-40 enhancement (down to 20.1%), which confirmed our previous data (P<0.05; Figure 3B). Western blotting showed that the expressions of p38 MAPK, ERK, JNK and NF-κB protein were knocked down by their respective siRNAs (Figure 3C).
Combining these findings with those of the previous study (7), they suggest that HCV induces the generation of ROS, which subsequently activates MAPKs, including p38 MAPK, ERK, and JNK. These kinases then activate NF-κB path ways, ultimately leading to the enhancement of YKL-40. The results also suggest that HCV-induced YKL-40 production occurs in both a ROS-independent (TNF-α-NF-κB) and -dependent manner (ROS-MAPKs-NF-κB).
Positive feedback loop of YKL-40 protein triggering HCV replication
We have provided the first evidence that YKL-40 production is increased in JFH1 cells. However, because its biological functions are mostly unexplored, we next assessed the effect of secreted YKL-40 protein on HCV replication. Human purified YKL-40 protein with serial dilutions was added into JFH1 cells (3 dpi) in a serum-free medium and incubated for 48 h. We found that doses of YKL-40 protein up to 100 ng/mL increased HCV RNA levels by 2.89-fold compared with phosphate-buffered saline (PBS) in JFH1 cells (P<0.05; Figure 4A). These findings indicated that YKL-40 reciprocally enhances HCV replication, forming a positive feedback loop.
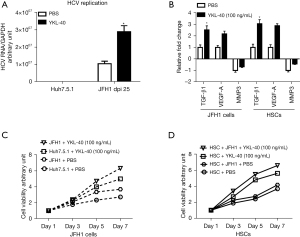
Effect of YKL-40 protein in both hepatic parenchymal cells and HSCs
We further explored the effect of the YKL-40 protein on hepatic profibrogenic cytokine release and cellular viability. Cells were incubated with the YKL-40 protein at a concentration of 100 ng/mL for 48 h. We found that purified YKL-40 protein increased TGF-β1and VEGF-A mRNA expression by 2.52-fold and 2.17-fold, respectively, compared with the PBS controls in JFH1 cells. Interestingly, similar stimulation effects of the YKL-40 protein on TGF-β1 and VEGF-A mRNA were also observed in HSCs (3.05- and 2.87-fold, respectively). To gather more evidence, we also investigated mRNA expression of matrix metalloproteinase 3 (MMP3), a profibrogenic cytokine, which was downregulated. We found that MMP3 mRNA levels were further decreased by purified YKL-40 protein compared with PBS controls in both JFH1 cells (0.67-fold) and HSCs (0.43-fold) (P<0.05; Figure 4B).
In addition, we monitored cellular viability of Huh7.5.1 and HSCs in the presence or absence of the JFH1 supernatant. These cells were treated with purified YKL-40 protein (100 ng/mL). We found that purified YKL-40 protein increased cellular viability in a time-dependent manner. Additionally, the enhancement of the stimulation by YKL-40 protein started earlier (day 3) and was more obvious compared with PBS treatment in HSCs in the presence of the JFH1supernatant (P<0.05; Figure 4C,4D).
In summary, our results demonstrated that only hepatic parenchymal cells infected with JFH1 produced the YKL-40 protein. The YKL-protein enhances HCV replication in a positive feedback loop and stimulates hepatic profibrogenic cytokine release and cellular viability of both hepatic parenchymal cells and HSCs. Our findings could provide a theoretical basis for the continual progression of liver fibrosis (Figure 5).
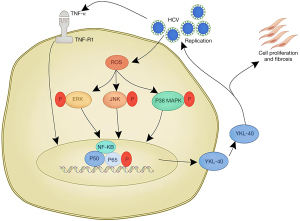
Discussion
In the present study, we have provided the first direct evidence from the JFH1 model that HCV enhances YKL-40 production. We have further proposed that accumulative activation of NF-κB controls the HCV-induced YKL-40 production through both a ROS-dependent MAPK signaling pathway and a ROS-independent TNF-α pathway. YKL-40 subsequently acts as a positive feedback mediator, reciprocally inducing HCV replication, and stimulating hepatic profibrogenic cytokine release and cellular viability. Our results emphasized the role of sustained induction of YKL-40 and activation of NF-κB in the liver fibrogenesis caused by HCV infection.
High serum levels of YKL-40 have been detected in chronic HCV-infected patients with liver fibrosis (16,25), but because direct evidence of the effect of YKL-40 dysfunction on HCV-mediated liver diseases has been lacking, we first explored the source of YKL-40 in the liver. We monitored YKL-40 expression in JFH1-infected cells with different infection times. The production of YKL-40 increased gradually with prolonged infection by JFH1-HCV, being mild at 5 dpi and 7 dpi, then resuming at 9 dpi. We speculated that this might be related to the growth characteristics of JFH1 cells, which gradually appeared apoptotic at 3 dpi and became markedly so from 5 to 8 dpi. They then recovered normal growth until 9 dpi, at which point a monoclonal strain had formed. Our data showed that the YKL-40 protein was produced by hepatic parenchymal cells infected with HCV and that the protein levels accumulated with prolonged HCV infection. The mechanisms by which the YKL-40 proteins accumulated during prolonged HCV infection were elucidated in a subsequent experiment. Meanwhile, no YKL-40 protein was produced in either hepatic parenchymal cells uninfected with JFH1 or in HSCs in the presence or absence of the JFH1 supernatant. We were surprised that YKL-40, as an inflammatory factor, was not produced by HSCs, which are widely considered the most relevant source of hepatic myofibroblasts. Therefore, we investigated the role of YKL-40 in HCV-related hepatic fibrogenesis in our next study.
We then explored the mechanisms by which HCV induced YKL-40 production. With transient stimulation by TNF-α, hepatic parenchymal cells secreted YKL-40 proteins and HCV-infected hepatic parenchymal cells (JFH1 cells) further enhanced YKL-40 production. This indicated that HCV indirectly accounted for the expression of YKL-40. The inflammatory factor TNF-α that was induced by HCV contributed to YKL-40 production. HSCs incubated with TNF-α still could not produce the YKL-40 protein, which was consistent with our previous data. Transient stimulation of TNF-α (5 h) was also sufficient to prolong YKL-40 production in either hepatic parenchymal cells or JFH1 cells. The cumulative expression reached its peak at 72 h. However, using siRNAs targeting TNF-R reduced YKL-40 production, which indicated that TNF-α is sufficient for but not essential to YKL-40 production. It also suggested that there could be certain other kinases controlling YKL-40 expression. A previous study had reported that transient stimulation by TNF-α resulted in sustained activation of NF-κB (30). We have experimentally demonstrated that activation of NF-κB is a core event in HCV-induced YKL-40 production, which was further indicated to occur in an NF-κB-dependent manner. Therefore, we propose that HCV infection induces TNF-α, which in turn activates NF-κB, and subsequently leads to the accumulation of YKL-40.
NF-κB is regulated by a variety of biological signaling pathways during HCV infection (4,25,31). A previous study reported that HCV induced oxidative stress and subsequently phosphorylated the MAPK signaling pathways of p38 MAPK, ERK and JNK, which cooperatively induced the activation of NF-κB (7). Thus, we explored whether there were other signaling pathways activating NF-κB that were involved in HCV-induced YKL-40 production. Using small molecule inhibitors and siRNAs, we confirmed that HCV induces ROS and activates MAPK signaling pathways, including p38 MAPK, ERK and JNK, which in turn activate NF-κB and subsequently stimulate YKL-40 secretion. Further experiments are needed to exclude the involvement of other signaling pathways (e.g., LPS-MYD88) in NF-κB-mediated YKL-40 production.
Further, we evaluated the biological function of the YKL-40 protein in HCV infection. Using purified YKL-40 protein, we found that it mildly stimulated HCV replication. We speculated that the mild increase was caused by the indirect inflammatory environment rather than direct interaction with HCV proteins. However, further experiments are needed to explore the precise mechanism. Hepatic profibrogenic cytokines are important markers for hepatic fibrosis. TGF-β, vascular growth factor, the ECM and remodeling enzymes are important factors involved in liver fibrosis. We examined the upregulated genes TGF-β1 and vascular endothelial growth factor A (VEGFA) as well as the downregulated gene MMP3. Purified YKL-40 protein stimulated hepatic profibrogenic cytokine release and cellular viability of both hepatic parenchymal cells and HSCs, and these effects were more significant in the presence of JFH1. These findings demonstrated that HCV induces YKL-40 production, which subsequently acts as a positive feedback to stimulate hepatic profibrogenic cytokine release and cellular viability in both hepatic parenchymal cells and HSCs. Our study emphasized the cooperative regulation of hepatic parenchymal cells and HSCs during the progression of liver fibrosis in HCV infection. The YKL-40 protein initiated HSCs fibrosis, even though HSCs were unable to be infected by HCV. Previous studies reported that the YKL-40 protein promotes tumor angiogenesis (32,33). Whether the YKL-40 protein has other biological functions in HCV infection remains to be explored.
Here, we report that HCV induced YKL-40 production in vitro and this increase was controlled by the activation of NF-kB in a ROS-dependent and -independent manner. Functionally, YKL-40 is an inflammatory factor for hepatic parenchymal cells that stimulates HCV replication and triggers hepatic profibrogenic cytokine release as well as cellular viability.
Conclusions
Here we have reported that HCV induces YKL-40 expression in both a ROS-dependent and -independent manner. On the one hand, HCV induces TNF-α secretion and subsequently activates NF-κB, which in turn inducesYKL-40 production. On the other hand, HCV induces oxidative stress, which consequently activates the MAPK signaling pathways of P38 MAPK, ERK and JNK, which in turn activate NF-κB. The activated NF-κB subsequently enhances YKL-40 production. As a positive feedback loop, the YKL-40 protein stimulates HCV replication while promoting cellular viability and the release of hepatic profibrogenic cytokines. This study also elucidated the cooperative regulation of hepatic parenchymal cells and HSCs in the progression of hepatic fibrosis.
Acknowledgments
We thank Dr. Raymond T. Chung for the gift of the Huh7.5.1 cell line and the infectious HCV virus JFH1 DNA construct.
Funding: This work was supported by the National Natural Science Foundation of China (81400624 to DC).
Footnote
Reporting Checklist: The authors have completed the MDAR reporting checklist. Available at https://dx.doi.org/10.21037/atm-21-4537
Data Sharing Statement: Available at https://dx.doi.org/10.21037/atm-21-4537
Conflicts of Interest: All authors have completed the ICMJE uniform disclosure form (available at https://dx.doi.org/10.21037/atm-21-4537). The authors have no conflicts of interest to declare.
Ethical Statement: The authors are accountable for all aspects of the work in ensuring that questions related to the accuracy or integrity of any part of the work are appropriately investigated and resolved.
Open Access Statement: This is an Open Access article distributed in accordance with the Creative Commons Attribution-NonCommercial-NoDerivs 4.0 International License (CC BY-NC-ND 4.0), which permits the non-commercial replication and distribution of the article with the strict proviso that no changes or edits are made and the original work is properly cited (including links to both the formal publication through the relevant DOI and the license). See: https://creativecommons.org/licenses/by-nc-nd/4.0/.
References
- AASLD-IDSA HCV Guidance Panel. Hepatitis C Guidance 2018 Update: AASLD-IDSA Recommendations for Testing, Managing, and Treating Hepatitis C Virus Infection. Clin Infect Dis 2018;67:1477-92. [Crossref] [PubMed]
- Bartels DJ, Sullivan JC, Zhang EZ, et al. Hepatitis C virus variants with decreased sensitivity to direct-acting antivirals (DAAs) were rarely observed in DAA-naive patients prior to treatment. J Virol 2013;87:1544-53. [Crossref] [PubMed]
- Cheng D, Zhao L, Zhang L, et al. p53 controls hepatitis C virus non-structural protein 5A-mediated downregulation of GADD45α expression via the NF-κB and PI3K-Akt pathways. J Gen Virol 2013;94:326-35. [Crossref] [PubMed]
- Serio I, Napoli L, Leoni S, Piscaglia F. Direct antiviral agents for HCV infection and hepatocellular carcinoma: facts and FADs. Transl Cancer Res 2019;8:S223-S32. [Crossref]
- Jee MH, Hong KY, Park JH, et al. New Mechanism of Hepatic Fibrogenesis: Hepatitis C Virus Infection Induces Transforming Growth Factor β1 Production through Glucose-Regulated Protein 94. J Virol 2015;90:3044-55. [Crossref] [PubMed]
- Estrabaud E, Vidaud M, Marcellin P, et al. Genomics and HCV infection: progression of fibrosis and treatment response. J Hepatol 2012;57:1110-25. [Crossref] [PubMed]
- Lin W, Tsai WL, Shao RX, et al. Hepatitis C virus regulates transforming growth factor beta1 production through the generation of reactive oxygen species in a nuclear factor kappaB-dependent manner. Gastroenterology 2010;138:2509-18, 2518.e1.
- De Minicis S, Candelaresi C, Marzioni M, et al. Role of endogenous opioids in modulating HSC activity in vitro and liver fibrosis in vivo. Gut 2008;57:352-64. [Crossref] [PubMed]
- Lin W, Wu G, Li S, et al. HIV and HCV cooperatively promote hepatic fibrogenesis via induction of reactive oxygen species and NFkappaB. J Biol Chem 2011;286:2665-74. [Crossref] [PubMed]
- Singh SK, Bhardwaj R, Wilczynska KM, et al. A complex of nuclear factor I-X3 and STAT3 regulates astrocyte and glioma migration through the secreted glycoprotein YKL-40. J Biol Chem 2011;286:39893-903. [Crossref] [PubMed]
- Recklies AD, Ling H, White C, et al. Inflammatory cytokines induce production of CHI3L1 by articular chondrocytes. J Biol Chem 2005;280:41213-21. [Crossref] [PubMed]
- Fontana RJ, Goodman ZD, Dienstag JL, et al. Relationship of serum fibrosis markers with liver fibrosis stage and collagen content in patients with advanced chronic hepatitis C. Hepatology 2008;47:789-98. [Crossref] [PubMed]
- Esmat G, Metwally M, Zalata KR, et al. Evaluation of serum biomarkers of fibrosis and injury in Egyptian patients with chronic hepatitis C. J Hepatol 2007;46:620-7. [Crossref] [PubMed]
- Rathcke CN, Vestergaard H. YKL-40, a new inflammatory marker with relation to insulin resistance and with a role in endothelial dysfunction and atherosclerosis. Inflamm Res 2006;55:221-7. [Crossref] [PubMed]
- Mehta P, Ploutz-Snyder R, Nandi J, et al. Diagnostic accuracy of serum hyaluronic acid, FIBROSpect II, and YKL-40 for discriminating fibrosis stages in chronic hepatitis C. Am J Gastroenterol 2008;103:928-36. [Crossref] [PubMed]
- Johansen JS, Christoffersen P, Møller S, et al. Serum YKL-40 is increased in patients with hepatic fibrosis. J Hepatol 2000;32:911-20. [Crossref] [PubMed]
- Fontana RJ, Dienstag JL, Bonkovsky HL, et al. Serum fibrosis markers are associated with liver disease progression in non-responder patients with chronic hepatitis C. Gut 2010;59:1401-9. [Crossref] [PubMed]
- Saitou Y, Shiraki K, Yamanaka Y, et al. Noninvasive estimation of liver fibrosis and response to interferon therapy by a serum fibrogenesis marker, YKL-40, in patients with HCV-associated liver disease. World J Gastroenterol 2005;11:476-81. [Crossref] [PubMed]
- Schiavon LL, Carvalho-Filho RJ, Narciso-Schiavon JL, et al. YKL-40 and hyaluronic acid (HA) as noninvasive markers of liver fibrosis in kidney transplant patients with HCV chronic infection. Scand J Gastroenterol 2010;45:615-22. [Crossref] [PubMed]
- Schiavon LL, Narciso-Schiavon JL, Carvalho Filho RJ, et al. Serum levels of YKL-40 and hyaluronic acid as noninvasive markers of liver fibrosis in haemodialysis patients with chronic hepatitis C virus infection. J Viral Hepat 2008;15:666-74. [Crossref] [PubMed]
- Pungpapong S, Nunes DP, Krishna M, et al. Serum fibrosis markers can predict rapid fibrosis progression after liver transplantation for hepatitis C. Liver Transpl 2008;14:1294-302. [Crossref] [PubMed]
- Navasa M, Forns X. How accurately and how early can we predict rapid fibrosis progression in hepatitis C virus-infected patients after liver transplantation? Liver Transpl 2008;14:1237-9. [Crossref] [PubMed]
- Fontana RJ, Litman HJ, Dienstag JL, et al. YKL-40 genetic polymorphisms and the risk of liver disease progression in patients with advanced fibrosis due to chronic hepatitis C. Liver Int 2012;32:665-74. [Crossref] [PubMed]
- Eurich D, Neumann UP, Boas-Knoop S, et al. YKL-40-gene polymorphism affects acute cellular rejection and fibrosis progression after transplantation for hepatitis C virus-induced liver disease. J Gastroenterol Hepatol 2013;28:153-60. [Crossref] [PubMed]
- Sarma NJ, Tiriveedhi V, Subramanian V, et al. Hepatitis C virus mediated changes in miRNA-449a modulates inflammatory biomarker YKL40 through components of the NOTCH signaling pathway. PLoS One 2012;7:e50826 [Crossref] [PubMed]
- Park JA, Drazen JM, Tschumperlin DJ. The chitinase-like protein YKL-40 is secreted by airway epithelial cells at base line and in response to compressive mechanical stress. J Biol Chem 2010;285:29817-25. [Crossref] [PubMed]
- Presser LD, Haskett A, Waris G. Hepatitis C virus-induced furin and thrombospondin-1 activate TGF-β1: role of TGF-β1 in HCV replication. Virology 2011;412:284-96. [Crossref] [PubMed]
- Lee J, Tian Y, Chan ST, et al. TNF-α Induced by Hepatitis C Virus via TLR7 and TLR8 in Hepatocytes Supports Interferon Signaling via an Autocrine Mechanism. PLoS Pathog 2015;11:e1004937 [Crossref] [PubMed]
- Séguin CA, Bernier SM. TNFalpha suppresses link protein and type II collagen expression in chondrocytes: Role of MEK1/2 and NF-kappaB signaling pathways. J Cell Physiol 2003;197:356-69. [Crossref] [PubMed]
- Hirano F, Komura K, Fukawa E, et al. Tumor necrosis factor alpha (TNF-alpha)-induced RANTES chemokine expression via activation of NF-kappaB and p38 MAP kinase: roles of TNF-alpha in alcoholic liver diseases. J Hepatol 2003;38:483-9. [Crossref] [PubMed]
- Polyak SJ, Morishima C, Shuhart MC, et al. Inhibition of T-cell inflammatory cytokines, hepatocyte NF-kappaB signaling, and HCV infection by standardized Silymarin. Gastroenterology 2007;132:1925-36. [Crossref] [PubMed]
- Shao R. YKL-40 acts as an angiogenic factor to promote tumor angiogenesis. Front Physiol 2013;4:122. [Crossref] [PubMed]
- Faibish M, Francescone R, Bentley B, et al. A YKL-40-neutralizing antibody blocks tumor angiogenesis and progression: a potential therapeutic agent in cancers. Mol Cancer Ther 2011;10:742-51. [Crossref] [PubMed]
(English Language Editor: K. Brown)