Puncture and localization for percutaneous endoscopic lumbar discectomy with C-arm navigation: a randomized controlled cadaver trial
Introduction
Lumbar disc herniation (LDH) is a common disease with symptoms of low back pain and/or radiating pain in the lower limbs that can seriously affect a patient’s daily activities and ability to work (1,2). With the rapid development of spinal endoscopy technology and surgical instrumentation, percutaneous endoscopic lumbar discectomy (PELD) has been increasingly used to treat LDH due to its minimally invasive surgical qualities that result in less paravertebral soft tissue and nerve injuries and a faster recovery time (3,4). Safe and accurate puncture and localization are prerequisites for PELD (5,6). The conventional method for puncture and localization in PELD is guided by C-arm fluoroscopy, but it cannot provide an accurate puncture depth or angle for the puncture needle (7). During the puncture and localization procedure in PELD using C-arm fluoroscopy, the surgeon needs to determine the puncture point based on bony landmarks and clinical experience and must rely on spatial imagination to visualize the puncture needle until the target site is reached. This process often requires repeated fluoroscopy and punctures, which may increase the risk of paravertebral soft tissue and nerve injury. It may also lead to a higher risk of radiation exposure to both patients and medical staff and prolong the surgical intervention (8). The learning curve for puncture and localization in PELD is steep for junior surgeons (9). In the past decade, C-arm navigation have been increasingly used for pedicle screw placement in spine surgeries which could provide the real-time navigation feedback of depth and angle of pedicle screw placement and have shown significant benefits of higher accuracy, shorter surgery time, and less intraoperative radiation exposure than conventional localization methods (10,11). However, the puncture needle used in PELD cannot be stably connected to the computer-assisted navigation adapter. This defect has largely restricted the use of computer-assisted navigation techniques in the puncture and localization for PELD. In this study, a navigation cannula that could be connected to a computer-assisted navigation system was designed and developed using 3D printing technology based on the conventional puncture needle features of a spinal foraminoscope. Assisted by the 3D-printed navigation cannula, C-arm navigation was used for the puncture and localization of PELD in adult cadavers. The purpose of this study was to investigate whether C-arm navigation could effectively shorten the time spent on puncture and localization, reduce the risk of intraoperative radiation exposure, improve the success rate of the first puncture, and flatten the learning curve for junior surgeons. We present the following article in accordance with the CONSORT reporting checklist (available at https://dx.doi.org/10.21037/atm-21-5844).
Methods
Development of the 3D-printed navigation cannula
Based on the features of a Joimax puncture needle, a navigation cannula was designed using IMAGEWARE v.12.1 software (Siemens PLM Software, Plano City, Texas, USA) that could be connected to a computer-assisted navigation system (Figure 1A,1B). This navigation cannula was 200 mm in length and hollow inside, with an internal diameter of 1 mm. The cannula was divided into two parts: the distal part had a length of 170 mm, an external diameter of 2.5 mm, and a small tip used for the puncture; the proximal part was used to connect to the adapter of the computer-assisted navigation system, with a length of 30 mm, an external diameter of 8 mm and a handle at the proximal end (Figure 1A,1B). The design data of the navigation cannula were saved as a .hbd format and imported into a metal 3D printer (SLM-300; Hanbang Science and Technology Ltd., Guangdong, China). A navigation cannula was printed by the metal 3D printer using titanium alloy (Ti-6Al-4V) as the printing material (Figure 1C,1D). After heat treatment, surface finishing, and high-temperature sterilization, the 3D-printed navigation cannula was ready to be used in clinical surgery (Figure 1E,1F).
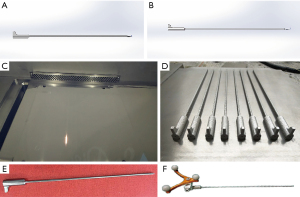
Subjects and materials
As the learning curve for puncture and localization in PELD is steep for junior surgeons, two junior spinal surgeons were involved in this study to investigate whether C-arm navigation could effectively flatten the learning curve for junior surgeons. Two junior spinal surgeons with one year of work experience with the same spine surgery team were randomly assigned to the two groups (group A using the C-arm navigation method for puncture and localization in PELD, and group B using the conventional localization method). Before the experiment, the surgeons had been trained in PELD for two months and had mastered the puncture and localization technique to the same proficiency level. A total of 16 adult cadavers were used for the experimental surgery. The cadavers, aged 28–65 years old, were treated with formaldehyde. There were ten male and six female cadavers with no lumbar injury, deformity, or surgical history. All cadavers were supplied from the human anatomical lab of Guangdong Medical University. All procedures performed in this study involving human participants were in accordance with the Declaration of Helsinki (as revised in 2013). The study was approved by regional ethical committee of Guigang City People’s Hospital, Guigang, China (No. GYYXLL-20180510). All donators’ next of kins gave their consent for all operations on the cadavers and publication of this paper. The equipment and materials used for the experiment were as follows: a Joimax transforaminal endoscopic surgical system (FS63421810; Joimax Inc., Germany), a C-arm X-ray machine (BV Vectra, Philips, Holland), a computer-assisted navigation system (Stealth Station S7 navigation system; Medtronic Sofamor Danek, Memphis, Tennessee, USA), and several 3D-printed navigation cannulas.
Study methods
In this study, a randomized, parallel-controlled cadaver trial was conducted to compare the effects of conventional localization using C-arm fluoroscopy and localization using C-arm navigation. And allocation ratio of this study was 1:1. The adult cadavers were randomly divided into groups A and B according to a random number table. Each group consisted of eight cadavers: five males and three females. The intervertebral foramen on both sides of the L3-L4, L4-L5, and L5-S1 levels in each cadaver were punctured using one of the two methods. For each group, there were 16 puncture sites at the L3-L4, L4-L5, and L5-S1 levels. The two junior surgeons used either the C-arm navigation method for puncture and localization or the conventional localization method on the eight cadavers in each group. The serial numbers of each level were marked according to the puncture sequence. In each lumbar level, 16 serial numbers [1–16] were marked. The technical parameters, such as fluoroscopy time, puncture time, and overall time taken for puncture and localization, as well as the number of fluoroscopies, puncture attempts, and success rate of the first puncture, were recorded for each method according to the lumbar levels and serial numbers.
Conventional puncture and localization procedure
The cadavers in group B were placed prone on a radiolucent operating table, using C-arm fluoroscopy for the puncture and localization in PELD. The midline of the spinous process was marked, and the puncture sites were identified by the transforaminal endoscopic spine system (TESSYS) technique (12,13). Under repeated C-arm fluoroscopy in the anteroposterior and lateral views, the puncture was performed using a Joimax puncture needle. The puncture angle and depth were adjusted step by step until the target site was reached. The puncture target of PELD was defined as follows: in the anteroposterior X-ray view, the tip of the puncture needle was placed at the apex of the superior articular process in the inferior vertebra; in the lateral X-ray view, the tip of the puncture needle was placed at the anteroinferior margin of the superior articular process in the inferior vertebra (the bottom of the intervertebral foramen).
C-arm navigation puncture and localization procedure
The cadavers in group A were placed prone on a radiolucent operating table, using C-arm navigation for the puncture and localization in PELD. The puncture sites were identified by the TESSYS technique. The puncture and localization were assisted by C-arm navigation. Firstly, the C-arm X-ray machine was connected to the computer-assisted navigation system. The navigation reference frame was fixed to the contralateral posterior superior iliac spine (Figure 2A). The infrared transceiver of the navigation system was placed at one end of the cadaver, directly facing the navigation reference frame. With four infrared light-reflecting diodes on the reference frame, signal transmission could be conducted to the infrared transceiver of the navigation system. The navigation cannula was stably installed on the navigation adapter (Figure 2B). Secondly, anteroposterior and lateral X-ray images of the lumbar vertebra were taken. The images were automatically uploaded into the computer navigation system (Figure 2C). Then, the accuracy of the navigation cannula was verified by using its tip to touch the navigation reference frame (Figure 2D). When the accuracy of the 3D-printed navigation cannula was confirmed, it was used to puncture and localize according to the typical TESSYS technique. With C-arm navigation, the puncture depth and angle of the navigation cannula could be adjusted dynamically based on real-time navigation feedback until the target site was reached (Figure 2E-2G). Finally, the anteroposterior and lateral X-ray images of the lumbar vertebra were used to confirm the location of the navigation cannula in the intervertebral foramen (Figure 2H,2I).
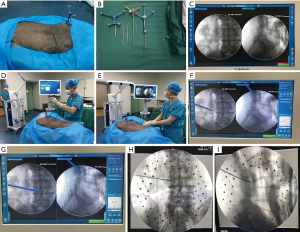
Observation indicators
Primary endpoints such as fluoroscopy time, puncture time, overall time taken for puncture and localization, as well as the number of fluoroscopies, puncture attempts, were recorded according to the serial numbers at the L3-L4, L4-L5, and L5-S1 levels. All these endpoints measures were obtained by the same secretary in our department after the cadaver was placed in the prone position and the median line along the spinous process and the iliac crest were marked. The learning curves of the overall time taken for the puncture and localization against the serial numbers at the above three lumbar levels were plotted for the two methods. All the primary endpoints and success rate of the first puncture were compared between the two methods, and the correlations between these indicators were analyzed.
Statistical method
SPSS 22.0 software (IBM, Chicago, USA) was used for all statistical analyses. Continuous and categorical variables between both groups were analyzed with the Student’s t-test and χ2 test, respectively. The Pearson correlation test was used to evaluate potential correlations between fluoroscopy time, puncture time, overall time taken for puncture and localization, number of fluoroscopies, and puncture attempts. A P value <0.05 was considered statistically significant.
Results
Sixteen adult cadavers were randomly divided into groups A and B according to a random number table. Each group consisted of eight cadavers. The intervertebral foramen on both sides of the L3-L4, L4-L5, and L5-S1 levels in each cadaver were punctured using one of the two methods. For each group, there were 16 puncture sites at the L3-L4, L4-L5, and L5-S1 levels respectively. The learning curves for the two methods were plotted based on the overall time taken for puncture and localization against the 16 serial numbers marked at the L3-L4, L4-L5, and L5-S1 levels, as shown in Figure 3. It can be seen from the figure that the learning curve for the C-arm navigation method was flatter. As the number of punctures increased, the overall time taken for puncture and localization decreased progressively. In contrast, although there was a decreasing trend in the overall time taken for puncture and localization using the conventional method, apparent fluctuations were observed.
A comparison of the two methods was made based on the technical parameters such as fluoroscopy time, puncture time, and overall time taken for puncture and localization, as well as the number of fluoroscopies, puncture attempts, and the success rate of the first puncture. The results are shown in Tables 1,2. The fluoroscopy, puncture, and total puncture-localization time for group A were 5.61 (±1.37), 2.29 (±1.22), and 9.78 (±2.66) minutes, respectively, and 15.72 (±3.59), 4.87 (±1.70), and 20.59 (±4.79) minutes for group B, respectively (P<0.05). Fluoroscopy was used on average 5.15 (±1.34) times in group A and 20.04 (±5.05) times in group B (P<0.05). There was an average of 1.08 (±0.28) puncture attempts in group A and 4.67 (±1.88) in group B (P<0.05). The success rate of the first puncture in group A was 91.7% and 10.4% in group B (P<0.05). All indicators in group A were significantly lower than those in group B (P<0.05).
Table 1
Technical parameters | Group A | Group B | t | P |
---|---|---|---|---|
Overall time of puncture and localization (minutes) | 9.78±2.66 | 20.59±4.79 | −13.676 | 0.000 |
Number of fluoroscopies | 5.15±1.34 | 20.04±5.05 | −19.746 | 0.000 |
Fluoroscopy time (minutes) | 5.61±1.37 | 15.72±3.59 | −18.225 | 0.000 |
Puncture attempts | 1.08±0.28 | 4.67±1.88 | −13.041 | 0.000 |
Puncture time (minutes) | 2.29±1.22 | 4.87±1.70 | −8.578 | 0.000 |
Table 2
Group | Total puncture sites | No. of targets reached on the first puncture | No. of targets reached after more than 1 puncture | Success rate of the first puncture | χ2 | P |
---|---|---|---|---|---|---|
Group A | 48 | 44 | 4 | 91.7% | 63.403 | 0.000 |
Group B | 48 | 5 | 43 | 10.4% |
The correlation analysis of the above indicators showed that there were significant correlations between the overall time of puncture-localization and puncture time (r=0.905; P<0.001), the overall time of puncture-localization and fluoroscopy time (r=0.978; P<0.001), the overall time of puncture-localization and puncture attempts (r=0.913; P<0.001), the overall time of puncture-localization and number of fluoroscopies (r=0.957; P<0.001), the fluoroscopy time and puncture attempts (r=0.903; P<0.001), the fluoroscopy time and number of fluoroscopies (r=0.972; P<0.001), and puncture attempts and number of fluoroscopies (r=0.924; P<0.001), as shown in Figure 4.
Discussion
Accurate and safe preoperative puncture and localization are crucial in PELD and may affect postoperative clinical outcomes (14). Conventional methods for puncture and localization in PELD largely depend on the surgeon’s technique, clinical experience, mastery of the local lumbar anatomy, tactile feedback from the body’s surface landmarks, and spatial understanding (5). Repeated fluoroscopy and punctures are often needed to reach the puncture targets, which may increase the risk of paravertebral soft tissue and nerve root injury, as well as patient and medical staff radiation exposure (6). Long-term exposure to large doses of X-ray radiation may be related to some diseases, such as leukemia, thyroid cancer, skin erythema, cataracts, and other malignancies (15-18). Moreover, localization by tactile feedback of bony landmarks is not sensitive and is unreproducible. Due to their limitations in spatial imagination and clinical experience, junior surgeons often have to perform repeated punctures and fluoroscopy to position the puncture needle percutaneously into the target site in the early stages of learning PELD, which may prolong their learning curve (5). Therefore, it is essential to find an alternative technique that can minimize the localization variance and learning curve of junior surgeons, improve the success rate of the first puncture, and reduce radiation exposure and surgery time.
In the past few years, new methods have been proposed for puncturing and localization to improve the location accuracy, reduce radiation exposure, and flatten the learning curve for PELD. Consequently, some achievements have been made in reducing the overall time taken for puncture and localization, reducing intraoperative X-ray radiation, increasing the accuracy of puncture and localization, and flattening the learning curve for PELD. Among them, medical imaging system-assisted puncture and localization for PELD have received particular attention. Wei et al. reported on 89 LDH patients who had received the O-arm method for puncture and localization in PELD. Their study showed that the surgeon could accurately determine the surgical anatomy and place the puncture needle into the optimal target site using multiplanar intraoperative images of the O-arm (19). However, the amount of radiation exposure or surgery time was not mentioned in their study. There are also some drawbacks to O-arm that raise concerns, such as the high radiation exposure, high cost, and poor-quality images of soft tissues. Choi et al. used a specially designed fluoroscope in PELD that integrated images of X-ray and MR (an XMR suite) with a table that could slide from the MR to the X-ray fluoroscopy suite and vice versa (20). Their results showed that a precise skin puncture point and intraoperative decompression could be provided by using XMR in PELD. Even so, the cost of the procedure was expensive, the equipment took up significant space in the operating room, and the patient had to remain in the operating theatre for a lengthy period. Liu et al. and Zhao et al. reported their experiences with ultrasound volume navigation technology for puncture and localization in PELD (21,22). The intraoperative real-time ultrasound images and preoperative lumbar CT or MR images were fused to guide the puncture and localization in PELD. Their results indicated that ultrasound volume navigation could improve the accuracy of puncture and localization and decrease surgery time, puncture times, and radiation exposure in PELD. However, there were some limitations to their studies, including the small sample size and the lengthy ultrasound training required for spine surgeons. In summary, although the new methods discussed above have demonstrated improvements in puncture and localization for PELD, they are often complicated and difficult to apply in clinical settings.
Since the end of the 20th century, computer-assisted navigation systems have been used for spinal surgery, serving as precise, safe, and fast novel approaches for spinal surgery (23-25). Extensive studies have indicated that, compared with conventional methods, computer-assisted navigation technology can improve the accuracy of pedicle screw placement in cervical, thoracic, and lumbar spine surgeries, enhance the safety of spine surgeries, and reduce patient and medical staff intraoperative radiation exposure. Therefore, computer-assisted navigation techniques should be an available choice for puncture and localization in PELD. However, without a handle at the proximal end, the Joimax puncture needle used in PELD cannot provide a stable connection to the computer-assisted navigation adapter. This defect has largely restricted the application of the computer-assisted navigation technique in puncture and localization for PELD.
In the past decade, 3D printing technology has been widely used for manufacturing medical products, with its main advantages being precision and flexibility (26,27). Furthermore, 3D printing technology has the capacity for personalized customization in small quantities, which shortens the product research and development cycle and decreases the expense. Given these advantages of 3D printing technology, we designed and developed a navigation cannula for the present study that could be connected to a computer-assisted navigation system. We used 3D printing technology based on the features of the conventional puncture needle used in a spinal foraminoscope. Together with the 3D-printed navigation cannula, C-arm navigation was used for the puncture and localization in PELD. The puncture depth and angle of the navigation cannula could be adjusted dynamically based on real-time navigation feedback until the target site was reached. This technique flattened the learning curve for junior surgeons, improved the success rate of the first puncture, and significantly reduced the surgery, fluoroscopy, and puncture/localization times.
In this study, it was evident that the learning curve for the C-arm navigation method was flatter than for the conventional method. As the number of punctures increased, the overall time taken for the puncture and localization decreased progressively. Although there was an overall decrease in time taken with the conventional method, notable fluctuations were also observed. Moreover, the fluoroscopy, puncture, total puncture-localization time, number of fluoroscopies, and puncture attempts were all significantly lower using C-arm navigation compared with the conventional fluoroscopy-guided technique (P<0.05). The success rate of the first puncture in group A was 91.7% and 10.4% in group B (P<0.05). The reasons for the superiority of the C-arm navigation method may be as follows: The conventional puncture and localization procedure in PELD relies heavily on the surgeon's technique, clinical experience, mastery of the local lumbar anatomy, and spatial imagination ability. The repeated use of fluoroscopy and punctures were needed in the conventional method to adjust the puncture angle and depth until the puncture targets were reached, which undoubtedly increased intraoperative radiation exposure and risk of paravertebral soft tissue and nerve root injury, prolonged the operative time, and extended the duration of the learning curve. When using C-arm navigation, the puncture depth and angle of the navigation cannula could be adjusted dynamically by the surgeon based on real-time navigation feedback until the target site was reached. Therefore, this technique increased the success rate of the first puncture and significantly reduced the fluoroscopy and puncture time, and the number of fluoroscopies and puncture attempts. Furthermore, using C-arm navigation can overcome the learning difficulties caused by the limited clinical experience and spatial imagination ability of junior surgeons. In this study there were significant correlations between fluoroscopy time and puncture attempts (r=0.903; P<0.001), puncture attempts and number of fluoroscopies (r=0.924; P<0.001), and overall time taken for puncture-localization with puncture time, fluoroscopy time, puncture attempts, and number of fluoroscopies, respectively (r=0.905, 0.978, 0.913, 0.957; P<0.001). The results of the correlation analysis signified that PELD using C-arm navigation could minimize surgery time and the risk of radiation exposure for both patients and medical staff by reducing fluoroscopy and puncture time, and the number of fluoroscopies and puncture attempts.
When using C-arm navigation for puncture and localization in PELD, the following issues should be noted: (I) The surgeon should master the use of the computer-assisted navigation system and make sure that every step is correct. (II) The infrared transceiver of the navigation system should face the navigation reference frame at a close range. There should be no obstruction between the reference frame and the infrared transceiver to ensure the real-time transmission of the navigation information. (III) The navigation reference frame should be stably fixed to the contralateral posterior superior iliac spine. If it becomes loose during surgery, the accuracy of the puncture and localization under C-arm navigation will be impaired, or unnecessary injury to the dural sac and nerve root may occur. (IV) The X-ray images uploaded to the computer-assisted navigation system should be the standard anteroposterior and lateral lumbar spine X-ray images. (V) Before using C-arm navigation to assist puncture and localization in PELD, the navigation accuracy of the navigation cannula should be checked using the lumbar bony structures. (VI) While using the navigation cannula for puncture and localization in PELD, brute force puncture should be avoided, as it could cause deformation of the navigation cannula and affect the accuracy of the puncture and localization.
This study has some specific strengths as listed above but also has several limitations. Due to the relatively small number of cadavers, a larger number of cadavers should be used in future studies to verify the present results. In addition, the number of fluoroscopies used is not an accurate indicator of radiation exposure because the radiation dose of each image was not calculated. Moreover, the tissue texture of a cadaver treated with formaldehyde is different from the living human body, which may have caused the present results to differ from those obtained in real-life clinical puncture and localization. In addition, computer navigation system and puncture and localization using C-arm navigation are expensive, and many hospitals do not have computer navigation system.
Conclusions
Puncture and localization using C-arm navigation for PELD dramatically flattened the learning curve for junior surgeons and significantly improved the success rate of the first puncture. Moreover, PELD using C-arm navigation minimized surgery time and the risk of radiation exposure for both patients and medical staff by reducing fluoroscopy and puncture time, and the number of fluoroscopies and puncture attempts.
Acknowledgments
The authors would like to thank Kyle Malone, M.S. and Chun-Po Yen, M.D. for their editorial and statistical support of this manuscript.
Funding: This work was supported by the National Natural Science Foundation Project of China (81760401), the Science and Technology Project of Guangdong Province (2016B090917001), the Sanming Project of Medicine in Shenzhen (SZSM201612019), the Science and Technology Base and Talent Specialization of Guangxi (AD17129017, AD17195042), the Science and Technology Project of Guigang City (1803002, 1834002) and the Science and Technology Plan Project of Maoming City (2020KJZX011).
Footnote
Reporting Checklist: The authors have completed the CONSORT reporting checklist. Available at https://dx.doi.org/10.21037/atm-21-5844
Trial Protocol: Available at https://dx.doi.org/10.21037/atm-21-5844
Data Sharing Statement: Available at https://dx.doi.org/10.21037/atm-21-5844
Conflicts of Interest: All authors have completed the ICMJE uniform disclosure form (available at https://dx.doi.org/10.21037/atm-21-5844). The authors have no conflicts of interest to declare.
Ethical Statement: The authors are accountable for all aspects of the work in ensuring that questions related to the accuracy or integrity of any part of the work are appropriately investigated and resolved. All procedures performed in this study involving human participants were in accordance with the Declaration of Helsinki (as revised in 2013). The study was approved by regional ethical committee of Guigang City People’s Hospital, Guigang, China (No. GYYXLL-20180510). All donators’ next of kins gave their consent for all operations on the cadavers and publication of this paper.
Open Access Statement: This is an Open Access article distributed in accordance with the Creative Commons Attribution-NonCommercial-NoDerivs 4.0 International License (CC BY-NC-ND 4.0), which permits the non-commercial replication and distribution of the article with the strict proviso that no changes or edits are made and the original work is properly cited (including links to both the formal publication through the relevant DOI and the license). See: https://creativecommons.org/licenses/by-nc-nd/4.0/.
References
- Chen X, Chamoli U, Vargas Castillo J, et al. Complication rates of different discectomy techniques for symptomatic lumbar disc herniation: a systematic review and meta-analysis. Eur Spine J 2020;29:1752-70. [Crossref] [PubMed]
- Jang SH, Chang MC. Follow-up of at least five years after lumbar transforaminal epidural steroid injection for radicular pain due to lumbar disc herniation. Ann Palliat Med 2020;9:116-8. [Crossref] [PubMed]
- Gadjradj PS, Harhangi BS, Amelink J, et al. Percutaneous Transforaminal Endoscopic Discectomy Versus Open Microdiscectomy for Lumbar Disc Herniation: A Systematic Review and Meta-analysis. Spine (Phila Pa 1976) 2021;46:538-49. [Crossref] [PubMed]
- Jarebi M, Awaf A, Lefranc M, et al. A matched comparison of outcomes between percutaneous endoscopic lumbar discectomy and open lumbar microdiscectomy for the treatment of lumbar disc herniation: a 2-year retrospective cohort study. Spine J 2021;21:114-21. [Crossref] [PubMed]
- Fan G, Gu X, Liu Y, et al. Lower Learning Difficulty and Fluoroscopy Reduction of Transforaminal Percutaneous Endoscopic Lumbar Discectomy with an Accurate Preoperative Location Method. Pain Physician 2016;19:E1123-34. [PubMed]
- Wu RH, Deng DH, Huang XQ, et al. Radiation Exposure Reduction in Ultrasound-Guided Transforaminal Percutaneous Endoscopic Lumbar Discectomy for Lumbar Disc Herniation: A Randomized Controlled Trial. World Neurosurg 2019; [Epub ahead of print]. [Crossref] [PubMed]
- Li Z, Zhang C, Chen W, et al. Percutaneous Endoscopic Transforaminal Discectomy versus Conventional Open Lumbar Discectomy for Upper Lumbar Disc Herniation: A Comparative Cohort Study. Biomed Res Int 2020;2020:1852070. [Crossref] [PubMed]
- Qin R, Liu B, Hao J, et al. Percutaneous Endoscopic Lumbar Discectomy Versus Posterior Open Lumbar Microdiscectomy for the Treatment of Symptomatic Lumbar Disc Herniation: A Systemic Review and Meta-Analysis. World Neurosurg 2018;120:352-62. [Crossref] [PubMed]
- Ao S, Wu J, Tang Y, et al. Percutaneous Endoscopic Lumbar Discectomy Assisted by O-Arm-Based Navigation Improves the Learning Curve. Biomed Res Int 2019;2019:6509409. [Crossref] [PubMed]
- Kelley BV, Hsiue PP, Upfill-Brown AM, et al. Utilization trends and outcomes of computer-assisted navigation in spine fusion in the United States. Spine J 2021;21:1246-55. [Crossref] [PubMed]
- Wallace N, Schaffer NE, Freedman BA, et al. Computer-assisted navigation in complex cervical spine surgery: tips and tricks. J Spine Surg 2020;6:136-44. [Crossref] [PubMed]
- Xiong C, Li T, Kang H, et al. Early outcomes of 270-degree spinal canal decompression by using TESSYS-ISEE technique in patients with lumbar spinal stenosis combined with disk herniation. Eur Spine J 2019;28:78-86. [Crossref] [PubMed]
- Kapetanakis S, Gkasdaris G, Angoules AG, et al. Transforaminal Percutaneous Endoscopic Discectomy using Transforaminal Endoscopic Spine System technique: Pitfalls that a beginner should avoid. World J Orthop 2017;8:874-80. [Crossref] [PubMed]
- Zhang M, Yan L, Li S, et al. Ultrasound-guided transforaminal percutaneous endoscopic lumbar discectomy: a new guidance method that reduces radiation doses. Eur Spine J 2019;28:2543-50. [Crossref] [PubMed]
- Scott MC, Galivanche AR, Mets EJ, et al. Patients' and Physicians' Knowledge of Radiation Exposure Related to Spine Surgery. Spine (Phila Pa 1976) 2020;45:E1507-15. [Crossref] [PubMed]
- Harrison Farber S, Nayar G, Desai R, et al. Radiation exposure to the surgeon during minimally invasive spine procedures is directly estimated by patient dose. Eur Spine J 2018;27:1911-7. [Crossref] [PubMed]
- Safari Variani A, Saboori S, Shahsavari S, et al. Effect of Occupational Exposure to Radar Radiation on Cancer Risk: A Systematic Review and Meta-Analysis. Asian Pac J Cancer Prev 2019;20:3211-9. [Crossref] [PubMed]
- Iglesias ML, Schmidt A, Ghuzlan AA, et al. Radiation exposure and thyroid cancer: a review. Arch Endocrinol Metab 2017;61:180-7. [Crossref] [PubMed]
- Wei S, Tao W, Zhu H, et al. Three-dimensional intraoperative imaging with O-arm to establish a working trajectory in percutaneous endoscopic lumbar discectomy. Wideochir Inne Tech Maloinwazyjne 2016;10:555-60. [PubMed]
- Choi G, Modi HN, Prada N, et al. Clinical results of XMR-assisted percutaneous transforaminal endoscopic lumbar discectomy. J Orthop Surg Res 2013;8:14. [Crossref] [PubMed]
- Liu YB, Wang Y, Chen ZQ, et al. Volume Navigation with Fusion of Real-Time Ultrasound and CT Images to Guide Posterolateral Transforaminal Puncture in Percutaneous Endoscopic Lumbar Discectomy. Pain Physician 2018;21:E265-78. [PubMed]
- Zhao Y, Bo X, Wang C, et al. Guided Punctures with Ultrasound Volume Navigation in Percutaneous Transforaminal Endoscopic Discectomy: A Technical Note. World Neurosurg 2018;119:77-84. [Crossref] [PubMed]
- Gardeck AM, Pu X, Yang Q, et al. The effect of simulation training on resident proficiency in thoracolumbar pedicle screw placement using computer-assisted navigation. J Neurosurg Spine 2020; [Epub ahead of print]. [PubMed]
- Era M, Maeda J, Tomonaga T. Effect of a computer-assisted navigation system on the lag screw placement accuracy of less experienced surgeons. J Orthop Sci 2021;26:823-6. [Crossref] [PubMed]
- Wood MJ, McMillen J. The surgical learning curve and accuracy of minimally invasive lumbar pedicle screw placement using CT based computer-assisted navigation plus continuous electromyography monitoring - a retrospective review of 627 screws in 150 patients. Int J Spine Surg 2014;8:27. [Crossref] [PubMed]
- Maroulakos M, Kamperos G, Tayebi L, et al. Applications of 3D printing on craniofacial bone repair: A systematic review. J Dent 2019;80:1-14. [Crossref] [PubMed]
- Ricles LM, Coburn JC, Di Prima M, et al. Regulating 3D-printed medical products. Sci Transl Med 2018;10:eaan6521. [Crossref] [PubMed]
(English Language Editor: D. Fitzgerald)