Abdominal multiorgan procurement from slaughterhouse pigs: a bespoke model in organ donation after circulatory death for ex vivo organ perfusion compliant with the 3 Rs (Reduction, Replacement & Refinement)
Introduction
Solid-organ transplantation has become the gold standard treatment for patients with end-stage organ failure. However, the supply of usable organs for transplantation is unable to meet the rising demand, which has driven the development of new approaches to meet that demand. To date, the challenge of increasing the donor pool has primarily been addressed by using older and higher risk extended criteria donor (ECD) organs, but they often have poorer outcomes compared to organs procured from standard criteria donors (SCD). Technological advances to meet this challenge, including novel methods of preservation and organ perfusion have also been explored. These approaches may facilitate improved organ assessment and permit optimization of ECD organs, increasing the supply and ultimately improving outcomes for patients.
With the expansion in novel approaches and technologies in the field of organ perfusion and regeneration, preclinical studies are required to develop, assess and translate to clinical practice, as they offer well-controlled conditions that allow investigation of the underlying molecular mechanisms (1).
Porcine models have been very successful and helpful pre-clinical models in translational transplantation research, particularly in the development of perfusion technology. Pigs have similar abdominal visceral and vascular anatomy to humans, with similarly sized organs and therefore represent an excellent large animal model in transplantation (2). Furthermore, the genomic similarities with humans, particularly regarding the immune system and inflammation-related protein expression also increase the value of this specific model (3).
Given the clear benefits of the porcine model, there has been a dramatic increase in the development of many porcine species-specific laboratory resources with specific antibodies, assays and kits that have facilitated in-depth molecular analysis and allowed the exploration of multiple new avenues of enquiry.
Despite the scientific benefits of large animal models, significant ethical issues remain, and the acceptability of animal experimentation remains highly controversial and consequently heavily regulated. To meet the stringent ethical standards, many research groups are faced with prohibitively high costs associated with animal husbandry and large animal experiments (4). Despite an exciting and rapidly developing field, an excellent pre-clinical model and major technical advances all aimed at addressing a critical clinical issue, research opportunities are being stifled by ethical and economic barriers.
We propose that designing a reliable and efficient procurement process for porcine organs from the slaughterhouse will offer a workable solution for many research groups. Utilizing the slaughterhouse resource is not only ethically advantageous but in line with the principle of ‘Replacement’ within the 3Rs (Reduction, Replacement & Refinement), which form the ethical framework for scientific animal research (5). It also provides organs retrieved from an animal model that closely resembles the donation after circulatory death (DCD) clinical scenario, which is a major area of focus when expanding the donor pool in transplant research.
Although slaughterhouse porcine organs have been used in multiple ex vivo perfusion experiments (6-8) a standardized methodology for multiorgan retrieval has not yet been described. Here, we report a multiorgan procurement procedure that uses a standardized approach and provides viable organs that represent a DCD model and can be used in the development of ex vivo organ perfusion. We present the following article in accordance with the ARRIVE reporting checklist where applicable (available at https://dx.doi.org/10.21037/atm-21-2494).
Methods
Slaughterhouse organ procurement protocol
Set-up and preparation
On-site and in close vicinity of the location where animals will be slaughtered a large table or surface is placed to conduct the multi-organ procurement procedure effectively and efficiently. The table should be draped and an intravenous fluid delivery stand and giving-set (Baxter Medical, Deerfield, USA) set up in preparation for ex situ cold flush-out of organs. A large bowl of ice and gauze is required for liver back-table perfusion (Figure 1) and should be prepared before commencing the procedure. Two liters of organ preservation solution is heparinized with 1,000 IU/L of unfractionated heparin sodium (Wockhardt UK, Ltd.) and kept at 4 °C (39.2 °F).
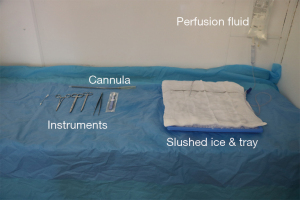
For kidney retrieval, a modified giving set is prepared to allow simultaneous direct cannulation of both renal arteries by connecting a rapid giving set to a Y-connector using sterile silicone tubing. The limbs of the Y-connector are extended using 20–30 cm lengths of silicone tubing, to which small arterial cannulae, such as Tibbs cannulae (DTR medical®) are secured. Plastic tubing clamps are incorporated to allow independent control of the two limbs.
Animals
Sus domesticus pigs (50–70 kg) are electrically stunned and while unconscious, exsanguinated by stab incision to the heart and great vessels in line with the standard abattoir process and in compliance the Welfare of Animals at the Time of Killing (England) Regulations 2015 (WATOK) and EU regulation 1099/2009. Whole blood is collected into a container with 20,000 IU of unfractionated heparin sodium (Wockhardt UK, Ltd.), or alternatively directly into blood collection bags and anticoagulated with citrate-phosphate-dextrose solution, for storage and preparation at the lab. The animals are briefly cleaned in a hot water bath at 60 °C (140 °F) for a pre-set time of 2 min, however, this may vary with other slaughterhouse establishments. The time from exsanguination to commencement of retrieval operation of the animal takes 5–10 min per animal and is similar to the ‘no-touch’ period following cardiac arrest in clinical human DCD retrieval procedures across Europe (9).
En bloc thoracoabdominal organ excision
The animals are then suspended from the posterior limbs, according to the local slaughterhouse procedure. A longitudinal midline incision is made to expose the abdomen and thorax and a circumferential incision around the anus and rectum allowing it to be pulled down in continuity with the gastro-intestinal tract. An en bloc removal of the abdominal and thoracic viscera is conducted to minimize damage to the organs and facilitate an ex situ separation of the required organs. The visceral block is explanted by detaching it from the longitudinal vertebral ligament the retroperitoneal space cutting these attachments flush against the vertebral column. The small and large bowel, duodenum, pancreas, stomach, esophagus, kidneys, spleen, heart, lungs, aorta and trachea are removed alongside the liver and diaphragm en bloc (Figure 2).
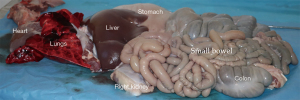
Liver procurement
The multi-organ block is transferred to the back-table where the liver is quickly inspected for damage or disqualifying tears and the liver parenchyma covered with slushed ice as the process of organ cannulation and perfusion commences. The distal (infra-renal) aorta can be quickly and easily identified and a 21-Fr Argyl straight aortic cannulaTM (Cardinal Health, Dublin Ohio, USA) is inserted for anatomical guidance (Figure 3). The organs are swiftly arranged in standard anatomical position but with the liver reflected caudally exposing the hepatoduodenal ligament, under-surface of the liver and gall bladder, where more slushed ice can be placed.
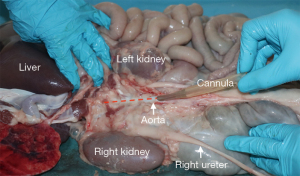
Infra-hepatic vena cava
The right kidney is identified and retracted laterally exposing the infra-hepatic inferior vena cava (IVC) as it enters the liver, it should be noted that in pigs, the hepatic segment of the IVC is intra-hepatic. From here the IVC is dissected inferiorly to the origin of the left renal vein, and is then divided, ensuring an adequate cuff of IVC beyond the most inferior part of the liver. The liver tissue extends on the posterior aspect of the vessel and is susceptible to damage if this is divided before the renal vein origin (Figure 4).
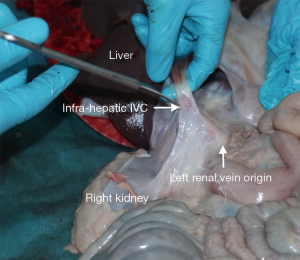
Portal cannulation
The portal vein (PV) is approached well away from the hepatic hilum where it lies deep to the pancreatic parenchyma at the junction of the superior mesenteric vein (SMV) and splenic vein (SV). The PV can be easily visualized once the pancreatic parenchyma has been spread exposing it within the pancreas. Once identified it should immediately be incised, cannulated and flushed with cold heparinized preservation fluid via a soft 18-Fr T-tube (Summit Medical, Cheltenham, UK) attached to the giving set (Figure 5A-5C).
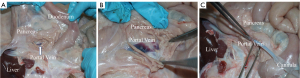
Hepatic artery
The porcine arterial inflow is variable and often branches very early, thus, preserving an aortic patch of the coeliac is advisable at this stage of the procurement process. This is helped by using the previously placed aortic cannula (Figure 3) as a guide. Superiorly, the thoracic aorta can be divided just above the diaphragm and inferiorly, the aorta is divided above the kidneys, leaving a cylinder of aorta around the cannula. The aortic tube can then be split on the posterior surface creating a superior mesenteric artery (SMA) and coeliac artery patch. Once the aortic patch has been fashioned, the artery is flushed with preservation fluid via a pediatric aortic cannula (Figure 6).
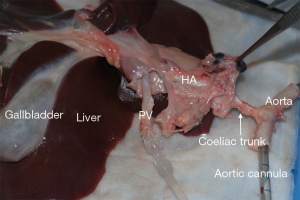
Bile duct and liver attachments
Once the arterial inflow, the PV and infra-hepatic IVC are divided, the remaining hepatoduodenal attachments are divided flush with the duodenum. The common bile duct (CBD) will be identified and divided as it enters the duodenum and is left long, to facilitate cannulation and bile collection during any experimental perfusions. This dissection is extended along the lesser curve of the stomach to the esophagus, dividing the diaphragmatic crus and the remaining diaphragmatic attachments are also carefully divided isolating the liver. The remaining vascular outflow, the supra-hepatic IVC is divided with a good length and circumferential cuff of the diaphragm. The separation of the liver is complete, and it can be transferred into the ice bowl for ongoing flushing.
Kidney procurement
Kidney retrieval begins with the same en bloc thoracoabdominal excision of the organs as described above. The modified giving set described under 2.1 is primed with preservation solution, and a pressure bag is used.
Arterial cannulation
The viscera are transferred to the back-table en bloc and placed with the dorsal aspect facing upwards. The infra-renal aorta is identified and the dorsal aspect divided longitudinally, starting caudally and ending when the origin of the SMA is seen (line of division is illustrated in Figure 3). The origins of the renal arteries are identified and cannulated using the modified giving set and Tibbs cannulae. The dorsal aspect of the renal artery is protected using damp gauze, and pincer-type clamps are applied to temporarily secure the cannulae in place whilst dissection is continued. Cold-flush of the organs is then started at a perfusion pressure no greater than 40 cmH2O (approximately 30 mmHg mean pressure) in order to achieve sufficient flush flow whilst avoiding excessive pressure. this is usually achievable within 1 min of the viscera being delivered to the back-table. Volume of cold flush is dependent of organ procured; 2 L for liver and 1 L for pancreas.
Dissection and retrieval of the left kidney
The ventral aspect of the aorta is divided, and the left renal vein exposed (allowing observation of the cold-flush effluent). The ureters are identified, cut inferiorly, and mobilized. Dissection is continued superiorly, mobilizing both kidneys from the peritoneal membrane laterally, anteriorly, and superiorly. On the left, the kidney must be mobilized from the pancreas. The left renal vein is divided at the junction with the IVC, and medial mobilisation of the left kidney is completed by cutting the aortic patch an appropriate distance from the origin of the renal artery, ensuring that it is taken with a cuff of peritoneum ventral to the hilar structures to protect the vasculature. The left kidney is then placed directly into a bowl of slush-ice for rapid topical cooling, with the arterial flush (secured by a pincer clamp) ongoing.
Dissection and retrieval of the right kidney
The IVC is then divided superiorly and inferiorly, and medial mobilization of the right kidney is completed by trimming the aortic patch. Again, a ventral cuff of peritoneum is taken to protect the hilar vasculature, and the right kidney is placed into slush-ice with cold flush ongoing.
Completion of cold-flush and initial bench-work
Once in slush-ice, the pincer-clamps are removed and the renal arteries dissected at the level of the aortic patch sufficient to encircle with a vascular sloop (MedasilTM), which is secured using an occlusive tube (CovidienTM Argyle vascular tourniquet kit) as a snugger. This fixes the Tibbs cannulae in place, prevents any leak whilst cold-flushing is completed, and allows initial bench-work to be performed. Bench work comprises of isolation of the vascular structures and the removal of adherent peritoneum and perinephric fat. Cold-flushing is complete once 500–750 mL preservation solution has run through each kidney and the venous effluent is clear.
Pancreas procurement
Again, en bloc thoracoabdominal excision of the viscera is used.
Aortic cannulation
The organs are transferred to the back-table where the infra-renal aorta is rapidly identified and cannulated with a 21-Fr Argyl straight aortic cannulaTM (Cardinal Health, Dublin Ohio, USA) connected and filled with the preferred preservation solution and clamped distally. The thoracic aorta is then identified and clamped with any lumbar branches closed with small vessel clamps to avoid unnecessary loss of perfusion fluid. The infra-renal aortic cannula is secured in place with a 0-2/0 VicrylTM (Ethicon, Livingston, UK) tie.
Mesenteric root
The colon is sharply dissected free from the duodenum and pancreas taking care to avoid entering the pancreas capsule, continuing until the root of the mesentery is identified (Figure 7A). The mesenteric root can then be divided (ideally using a vascular stapling device, although an under-running suture can also be used) as far away from the pancreas as possible (Figure 7B), as some branches for the duodenal segment of the porcine pancreas often depart from the initial 2–3 cm of the SMA. With the mesenteric root divided and cold perfusion via the aortic cannula ongoing, the abdominal multi-organ block can be placed on a large shallow bowl and covered with slushed ice and ice applied directly to organs to facilitate cooling. Stapling or suturing the mesenteric root is essential to minimize wastage of perfusion fluid through the bowel and improve the efficiency of the cold flush.
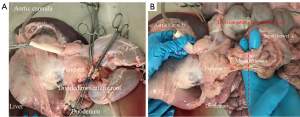
Bowel resection
While the perfusion is running, the small and large bowel can be removed in continuity with the rectum by dividing the distal duodenum. When performing this maneuver attention is paid to the ureters that run lateral to the large bowel but can be attached posteriorly by a peritoneal layer. Once identified, the ureters can be divided 6–7 cm from the renal hilum.
The proximal duodenum is divided or stapled distal to the pylorus taking care to avoid the structures of the hepatic hilum. The stomach can be dissected free cutting the remaining hepatogastric ligament along the lesser curve and the gastrosplenic ligament including the short gastric arteries that run along the greater curve. The PV is encountered during this part of the procedure and should be cut close to the liver, providing good length for the pancreas.
The spleen, which is loosely attached to the pancreas, should be safely removed from the hepatopancreatic block without damaging the pancreas. The splenic artery and vein can be identified 2–3 cm from the splenic hilum, away from the area where potential pancreatic vessels may branch out and they can be tied separately and divided. The splenic artery and vein can also be cannulated and used for dual pancreatic perfusion to ensure an effective flush of the organ.
Separation of the kidneys and pancreas
The pancreas and the duodenum should be dissected with a minimal mobilization of the gland and without opening the capsule. The duodenum is already divided proximally (distal to the pylorus) and distally at the ligament of Treitz (as previously described) (Figure 8). The splenic artery and vein are ligated and/or cannulated at the time of the removal of the spleen (as previously described). The aorta is then cut cranial to the renal arteries, preserving both the mesenteric artery and the celiac trunk to the aortic stump, and therefore preserving the arterial supply to the whole pancreas (Figure 9).
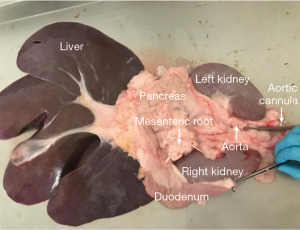
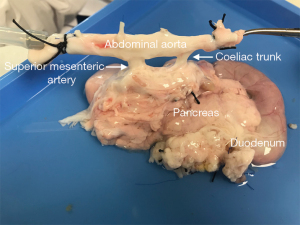
Packing and transport of all procured and perfused organs
Once the back-table perfusion and inspection of organs has been completed, the organs are individually placed in bags, e.g., a sterile PVC intestinal bag (Bunzl, Leicester, UK) containing 1–1.5 L of cooled preservation solution and placed in an icebox for transfer back to the laboratory. Alternatively, the kidneys can be perfused using a Hypothermic Machine Perfusion system (e.g., Kidney Assist Transport, Organ-Assist, Groningen, The Netherlands).
Results
Our group has performed 81 multiorgan procurements using the described methodology to produce successful, replicable and efficient procurement results summarized in Table 1. In our experience the time from exsanguination to the initiation of cold perfusion or warm ischemia time (WIT) typically takes between 12–20 min, but must be completed in less than 30 min to be acceptable for experimental normothermic perfusions. However, as previously published work has demonstrated, a WIT of less than 60 min has high survival rates (in a transplant model) when treated with normothermic machine perfusion (NMP) (10-12).
Table 1
Organ | Model | Device | Perfusate | Number of organs | Preservation length |
---|---|---|---|---|---|
Liver | NMP | OrganOx metra® | RBC and Gelofusine® | 10 | 12–26 hours |
Liver | SCS + MRI | Cold Storage | UW® Solution | 2 | 4–6 hours |
Kidney | HMP/NMP | Kidney AssistTM | Soltran®/RBC based perfusate | 162 | 4–24 hours |
Pancreas | HMP/NMP | Waves® machine/Kidney AssistTM | IGL-1®/IGL-2®/RBC based perfusate | 12 | 4–9 hours |
HMP, hypothermic machine perfusion; IGL-1, Institut Georges Lopez-1; IGL-2, Institut Georges Lopez-1; NMP, normothermic machine perfusion; MRI, magnetic resonance imaging; RBC, red blood cell; SCS, static cold storage; UW, University of Wisconsin.
A diagrammatic overview of the multiorgan procurement with critical timings is summarized in Figure 10.
Discussion
In this article we have described a bespoke model for abdominal multiorgan procurement using organs from large slaughterhouse animals (porcine), after a procedure that is similar to the DCD donor process. Procured organs can then be cold stored or perfused until arrival in the research laboratory where relevant analyses or targeted interventions will be carried out.
Aside from the clear ethical and economic advantages, this strategy has substantial scientific significance as it is an excellent model for DCD organs, which represent the fastest-growing source of organs for transplant, having doubled over the last 10 years in the UK (13). The expanding group of older, and higher risk donors have several limitations due to their susceptibility to ischemia reperfusion injury, increased complications peri-operatively and often worse long-term graft and patient survival. As this is a crucial area of transplant research that needs to be investigated, we consider this multiorgan procurement model using the slaughterhouse pig highly relevant, especially as in this model the time from exsanguination of the animal to cold perfusion closely mimics the WIT that occurs in clinical DCD organ retrieval.
Many research groups rely heavily on discarded human organs for ex situ perfusion research and for many years, this has been a valuable resource for transplant research. However, it is often unpredictable whether and when these organs become available; also, the variation as regards co-morbidity and condition or length of cold ischemic times is rather large adding to the generic heterogeneity already present. For these and other reasons discarded human organs have become an impracticable, less scientifically valuable, and often unreliable source of organs. Our model aims to overcome many of these challenges as it provides the ability to design well planned and controlled experiments in otherwise healthy organs which have been subjected to a specific insult, such as the warm ischemia associated with DCD retrieval, and therefore can provide valuable and reproducible conditions for investigators interested in DCD organ research. Slaughterhouse practices may differ globally, but this protocol, outlines the procedure in the UK context, however in cases of differing abattoir practices, the technique and procedures described here do not need to differ after investigators confirm animal death. Furthermore, the availability of vascular grafts from the animal facilitates vascular reconstruction to overcome potential variations in vascular anatomy that may impact the perfusion of the organ. Avoiding the use of pigs bred exclusively for large animal research in the laboratory, instead using animals that are already being used in the food industry, adheres to the core ethical principle of reduction. This approach is clearly preferable when considered in the context of the ‘3Rs’ (Reduction, Refinement, Replacement) (14) ethical principles that have been developed to underpin animal research in the past 50 years.
Despite its advantages, the model does have some limitations. Primarily, the brief high-temperature water bath at 60 °C (140 °F) for hair removal of the animal creates a sudden heat shock that may affect the tissues. This may result in aspiration of water rendering the lungs unsuitable, but may also add injury to the abdominal organs not normally seen in clinical DCD retrieval. However, given the swift en bloc retrieval, cold flush and application of topical ice, in our experience the organ temperature is rapidly reduced and the abdominal organs have performed well in experimental perfusions. With the location of the retrieval procedure in the abattoir, standard sterile operative conditions are not possible until the organs are transferred to the sterile back table. However, our procedure allows researchers to quickly retrieve abdominal organs, cold perfuse them, and move from the abattoir back-bench (prepared in a sterile fashion) to the more controlled environment of the laboratory, therefore limiting any opportunity for microbiological contamination of the organs. Additionally, as this is a DCD model and porcine blood tends to be hypercoagulable, to limit the development of micro-thrombi within the organ, we suggest adding 10,000 IU of heparin to each liter of the preservation solution to achieve a homogeneous perfusion of all organs.
Conclusions
We consider multiorgan procurement in slaughterhouse pigs a highly clinically relevant research model that will allow the effective and efficient retrieval of viable organs after circulatory death to be assessed, preserved and/or (re)perfused. The model constitutes an important contribution to the field of pre-clinical organ perfusion research where ethically and economically efficient experiments are in high demand are required. The model facilitates projects of organ preservation and reconditioning, but also allows decellularization and bioengineering work to be conducted. We feel that this approach may be a valuable and affordable addition to the rapidly growing field of research in organ donation, perfusion and transplantation.
Acknowledgments
The authors would like to acknowledge all members of the team at the abattoir who wish to remain anonymous. Without their dedicated support and patience, the research work carried out over the past three years would not have been possible. They allow us to disrupt their busy job for the sake of research and with no direct personal gain for which we are very grateful. This work has been presented in poster form at the American Transplant Congress 2020.
Funding: None.
Footnote
Reporting Checklist: The authors have completed the ARRIVE reporting checklist. Available at https://dx.doi.org/10.21037/atm-21-2494
Conflicts of Interest: All authors have completed the ICMJE uniform disclosure form (available at https://dx.doi.org/10.21037/atm-21-2494). The authors have no conflicts of interest to declare.
Ethical Statement: The authors are accountable for all aspects of the work in ensuring that questions related to the accuracy or integrity of any part of the work are appropriately investigated and resolved. Experiments were performed in compliance with the Welfare of Animals at the Time of Killing (England) Regulations 2015 (WATOK) and EU regulation 1099/2009, therefore did not require a personal or project licence to perform.
Open Access Statement: This is an Open Access article distributed in accordance with the Creative Commons Attribution-NonCommercial-NoDerivs 4.0 International License (CC BY-NC-ND 4.0), which permits the non-commercial replication and distribution of the article with the strict proviso that no changes or edits are made and the original work is properly cited (including links to both the formal publication through the relevant DOI and the license). See: https://creativecommons.org/licenses/by-nc-nd/4.0/.
References
- Baehr A, Klymiuk N, Kupatt C. Evaluating Novel Targets of Ischemia Reperfusion Injury in Pig Models. Int J Mol Sci 2019;20:4749. [Crossref] [PubMed]
- Dondelinger RF, Ghysels MP, Brisbois D, et al. Relevant radiological anatomy of the pig as a training model in interventional radiology. Eur Radiol 1998;8:1254-73. [Crossref] [PubMed]
- Dawson HD, Smith AD, Chen C, et al. An in-depth comparison of the porcine, murine and human inflammasomes; lessons from the porcine genome and transcriptome. Vet Microbiol 2017;202:2-15. [Crossref] [PubMed]
- Thulin JD, Bradfield JF, Bergdall VK, et al. The cost of self-imposed regulatory burden in animal research. FASEB J 2014;28:3297-300. [Crossref] [PubMed]
- Prescott MJ, Lidster K. Improving quality of science through better animal welfare: the NC3Rs strategy. Lab Anim (NY) 2017;46:152-6. [Crossref] [PubMed]
- Grosse-Siestrup C, Fehrenberg C, von Baeyer H, et al. Multiple-organ harvesting for models of isolated hemoperfused organs of slaughtered pigs. ALTEX 2002;19:9-13. [PubMed]
- Ferrer J, Scott WE 3rd, Weegman BP, et al. Pig pancreas anatomy: implications for pancreas procurement, preservation, and islet isolation. Transplantation 2008;86:1503-10. [Crossref] [PubMed]
- Blum MF, Liu Q, Soliman B, et al. Comparison of normothermic and hypothermic perfusion in porcine kidneys donated after cardiac death. J Surg Res 2017;216:35-45. [Crossref] [PubMed]
- Wind J, Faut M, van Smaalen TC, et al. Variability in protocols on donation after circulatory death in Europe. Crit Care 2013;17:R217. [Crossref] [PubMed]
- Reddy SP, Bhattacharjya S, Maniakin N, et al. Preservation of porcine non-heart-beating donor livers by sequential cold storage and warm perfusion. Transplantation 2004;77:1328-32. [Crossref] [PubMed]
- Vogel T, Brockmann JG, Pigott D, et al. Successful transplantation of porcine liver grafts following 48-hour normothermic preservation. PLoS One 2017;12:e0188494. [Crossref] [PubMed]
- Schön MR, Kollmar O, Wolf S, et al. Liver transplantation after organ preservation with normothermic extracorporeal perfusion. Ann Surg 2001;233:114-23. [Crossref] [PubMed]
- Dutkowski P, Schlegel A, de Oliveira M, et al. HOPE for human liver grafts obtained from donors after cardiac death. J Hepatol 2014;60:765-72. [Crossref] [PubMed]
- Rusche B. The 3Rs and animal welfare - conflict or the way forward? ALTEX 2003;20:63-76. [PubMed]