Impact of hydrogel stiffness on the induced neural stem cells modulation
Introduction
Neural stem cells (NSCs) can self-renew and differentiate, and are regarded as a promising therapy for neural injuries. In particular, induced neural stem cells (iNSCs) developed by directly reprogramming somatic cells through several transcription factors exhibit pluripotency to differentiate into neurons, astrocytes, and oligodendrocytes. It provides alternatives to induced pluripotent stem cells (iPSCs) with the advantages of easier culture and safer. Moreover, iNSCs also avoid many of the problems of immune rejection, cell source, and ethical issues (1), they are ideal seed cells for neural regeneration. However, the support and differentiation of iNSCs are mainly generated by complex growth factors with a long induction process (2,3), and apart from efficiency, the safety, controllability of differentiation, and long-term survival should be considered and require improvement. The effective regulation of the survival, proliferation, and differentiation of iNSCs has become a critical issue for its application.
It is well known that the cellular microenvironment, composed of extracellular matrix (ECM), bio-factors and physical cues, can directly modulate the fate of NSCs, including their survival, proliferation, migration, and differentiation (4). The mechanical properties of the ECM were found to control cellular fate independently (5,6). In particular, the hydrogels, as native ECM mimics with similar stiffness to brain tissue, were often used to support cells and regulate their behavior (7). For example, a soft matrix of 20 kPa, which was similar to brain tissue, induced bone marrow mesenchymal stem cells to differentiate into nerve cells (1). Moreover, iPSCs derived NSC was found differentiated into smooth muscle cells on the stiff surface, while became glial cells on the soft surface (8). It suggested the potential roles of stiffness in iNSC modulation. But inducing iNSC to major types of neural lineages was still a key problem in neural regeneration, that is, the formation of “brain-like tissue”. In this study, the effects of “brain-similar” matrix stiffness on the fates of iNSCs will be investigated. Hyaluronic acid (HA) was the main structural component in the brain ECM and often be used for highly hydrated matrix preparation in neural tissue engineering. So, the HA hydrogels were prepared to mimic brain ECM, and gradient stiffness will be used to test the impacts on iNSCs adhesion, proliferation, and differentiation. It is important for understanding the iNSCs regulation and their future applications.
We present the following article in accordance with the MDAR reporting checklist (available at https://dx.doi.org/10.21037/atm-21-6189).
Methods
Preparation of the hydrogel
Sodium hyaluronate (FREDA, China) of 0.6, 0.8, 1.0, 1.2, and 1.8 g samples was dissolved respectively in 10 mL ddH2O to prepare 0.6%, 0.8%, 1.0%, 1.2%, and 1.8% HA solutions, respectively. After adjusting pH to 3.5–4.75 by adding 1 mol/L HCl, adipic acid dihydrazide (ADH) was added and thoroughly stirred, and the mixture was then filled into 48-well plates, followed by adding 1-ethyl-3-(3-dimethylaminopropyl) carbodiimide hydrochloride (EDC) (HA: ADH: EDC =1:5:2) to crosslink for 3 days. Finally, the hydrogels were freeze-dried and UV sterilized for cell culture.
Morphology and stiffness detection of the hydrogel
For morphological testing, the structure of the freeze-dried hydrogels was detected, and pore size was measured by scanning electron microscopy (SEM).
The stiffness of wet hydrogel was quantitatively characterized by storage modulus and measured by rotational rheometer through the Small Amplitude Oscillatory Shear (SAOS) test. Briefly, hydrogels of different concentrations were crosslinked with the above method in a mold of 3 cm diameter to form a 1mm thin sheet and for the SAOS test with the following parameter settings: frequency sweep tests, frequency range from 0.1 to 100 rad/s, and strain =1%.
Culture of iNSCs
iNSCs were generated from reprogrammed human blood mononuclear cells following our previous method and transfected EGFP. The iNSCs was identitied by NSC markers Sox1, Sox2, OLIG2, PAX6, NESTIN, GFAP and Ki67 (9). Subsequently, iNSCs were seeded in 0.6%, 0.8%, 1.0%, 1.2%, and 1.8% HA hydrogels in 48-well plates at the concentration of 2×105 cells per well. Cells were cultured in proliferation medium [DMEM/F12: neurobasal =1:1, 1× N2, 1× B27, 2 mM GlutaMAX, 1% NEAA (Life Technologies, USA), 10 ng/mL rhLIF (Millipore, USA), 3 µM CHIR99021, and 2 µM SB431542 (Gene Operation, USA)] for the first 3 days, then changed into neural cell standard medium (DMEM/F12: neurobasal =1:1, 1× N2, 1× B27, 2 mM GlutaMAX, 1% NEAA) for the following 14 days.
Cell proliferation by CCK-8 assay
The cell proliferation in different hydrogels was assessed by CCK-8 kits (Dojindo, Japan) on days 3, 7, 10, and 14 of standard medium culture. Briefly, 300 µL 10% CCK-8 solution per well were incubated for 3 hours, then 100 µL solution per well was sucked out to a 96-well plate for the 450 nm absorbance value test.
Cell differentiation by immunofluorescence staining
The proliferation and differentiation of iNSCs in different hydrogels were examined by immunofluorescence staining. The cells were fixed with 4% paraformaldehyde, and Sox2 was used to mark iNSCs (1:800, Santa Cruz, CA, USA) and Ki67 (1:500, Millipore, USA) to show the proliferation. Tuj-1 (1:500, Sigma, USA) and NeuN (1:500, Invitrogen, USA) were used for neuron differentiation, and GFAP (1:200, ZSGB-BIO, China) and O4 (1:1,000, R&D, USA) were used for astrocytes and oligodendrocytes. After routine primary and secondary antibodies staining, cells were photographed under confocal microscopy and quantified by ImageJ software, while the 0.6% hydrogel degraded in 14 days and cells of this group were not stained.
Statistical analysis
The data were expressed as mean ± SD and analyzed with IBM SPSS Statistics 26. The hydrogel pore size was analyzed by ANOVA, and the OD value was examined by chi-square test. The differences were considered significant when P<0.05 (confidence level of 95%).
Results
Morphology of the hydrogels
The freeze-dried hydrogel presented a loose, porous sponge structure (Figure 1A), with uniform pore size under SEM, and the pore sizes gradually decreased with the increase of HA concentration (Figure 1B, Table 1).
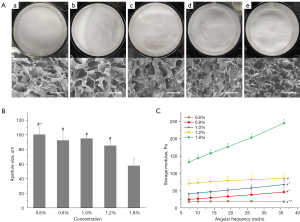
Table 1
Concentration | Aperture size (μm) | Storage modulus (Pa) |
---|---|---|
0.60% | 100.380±10.267#^ | 17.990–20.021+*^ |
0.80% | 92.424±11.766# | 28.778–46.900+* |
1.00% | 94.980±2.274# | 41.065–77.621+* |
1.20% | 85.236±6.655# | 70.542–85.8923+ |
1.80% | 57.849±10.212 | 136.277–250.3633 |
#, significant difference (P<0.05) from 0.6% HA; ^, significant difference (P<0.05) from 1.0% HA; *, significant difference (P<0.05) from 1.2% HA; +, significant difference (P<0.05) from 1.8% HA. HA, hyaluronic acid.
Stiffness of the hydrogel
Rheometer results showed that the stiffness of HA hydrogels expressed by storage modulus, increased with increasing concentration (Figure 1C). When angular frequency increased, the stiffness of the 0.6–1.2% gradient HA hydrogels increased gradually, while that of the 1.8% group increased rapidly and obviously. There were significant differences in HA hydrogels with different concentrations (P<0.05).
Adhesion and proliferation of iNSCs on hydrogels
After being seeded in hydrogels, many iNSCs aggregated into spheres and were seen to adhere to the surface or inside the hydrogel in each group under fluorescence microscopy (Figure 2A). After culture in the hydrogel for 14 days in standard medium, iNSCs of all 0.8–1.8% HA hydrogel groups expressed Sox2 and Ki67 on immunofluorescence staining, indicating the cells maintained stem cell proliferative properties (Figure 2B-2D, Table 2). As 0.6% hydrogels degraded, the results were not shown. However, the degree of proliferation was different in hydrogels with different stiffness. The proportion of Sox2 positive stem cells was higher in 1.8% hydrogel, while lower in softer hydrogels with a trend of differentiation, especially in 1.2% hydrogel.
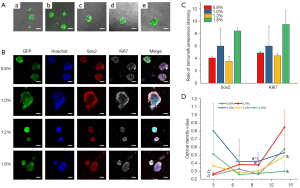
Table 2
Concentration | Sox2 (%) | Ki67 (%) |
---|---|---|
0.80% | 4.169±0.164 | 4.986±0.272 |
1.00% | 6.093±2.801 | 6.093±3.233 |
1.20% | 3.631±0.821 | 4.524±0.351 |
1.80% | 8.530±0.547 | 9.582±2.212 |
iNSCs, induced neural stem cells; HA, hyaluronic acid.
The CCK-8 test showed the iNSCs proliferated rapidly with similar trends in 1.0%, 1.2%, and 1.8% HA hydrogels on the 3rd day. The OD value of the 1.0% hydrogel group was the highest, indicating this middle-stiffness hydrogel was more suitable for cell short-term proliferation. However, when culture time extended to 14 days, the cell proliferation in 0.6% and 0.8% hydrogel showed continuously rising trends, indicating that hydrogels with lower stiffness were more suitable for long-term cell proliferation. In particular, iNSCs in 0.8% HA hydrogel grew the fastest at the end of culture (Figure 2D).
Differentiation of iNSCs by immunofluorescence staining
The immunofluorescence staining results (Figure 3A-3C, Table 3) show that iNSCs on hydrogels of different concentrations could differentiate into neurons and glial cells. Cells in 0.8% hydrogel were more likely to differentiate into Tuj-1 and NeuN positive neurons and could also differentiate into a relatively high proportion of O4-positive oligodendrocytes and less GFAP-positive astrocytes. The ratio of neurons/glial cells in 0.8% hydrogel was higher than other hydrogels, suggesting that low-stiffness hydrogel was the most conducive to neuron differentiation. In 1.0% hydrogel, iNSCs were less differentiated with a meager differentiation rate but higher Sox2 positive rate (Figure 3D), indicating the iNSCs tended to maintain their stemness. In 1.2% hydrogel, iNSCs differentiated most easily, with the highest rates of Tuj-1 and NeuN positive neurons, GFAP positive astrocytes, and O4 positive oligodendrocytes. In 1.8% hydrogel, cells differentiated less, and were mainly GFAP and O4 positive, suggesting that iNSCs in hydrogels with higher stiffness were inclined to differentiate into glial cells.
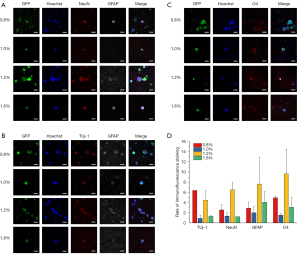
Table 3
Concentration | Tuj-1 (%) | NeuN (%) | GFAP (%) | O4 (%) |
---|---|---|---|---|
0.80% | 6.425±0.003 | 2.604±1.007 | 2.956±1.216 | 4.982±0.284 |
1.00% | 0.980±0.564 | 1.397±0.693 | 2.051±1.141 | 1.577±0.265 |
1.20% | 4.525±1.821 | 6.550±1.425 | 7.667±5.239 | 9.673±4.847 |
1.80% | 1.355±0.124 | 1.279±0.012 | 4.117±2.077 | 3.129±1.864 |
iNSCs, induced neural stem cells; HA, hyaluronic acid.
Discussion
iNSCs achieved by reprograming have great therapeutic potential for neural injuries, and the effective control of their fate and function is crucial for their application. Soft tissue of the central nervous system has a modulus of elasticity between 150–1,500 Pa (10), and in this study, soft HA hydrogels were used to mimic brain tissue for regulating the growth and differentiation of iNSCs. HA is a major component of brain ECM and has natural advantages in regulating cellular growth, differentiation, and migration (11). HA hydrogels of different stiffness were obtained by adjusting the concentration from 0.6% to 1.8%, with a modulus of elasticity between 25–250 Pa.
The results showed the proliferation of iNSCs was regulated by hydrogel stiffness. iNSCs survived and maintained proliferation in all hydrogels but this effect was greatest in softer 0.8–1.0% HA hydrogels with a gradual upward trend, suggesting softer HA hydrogel are suitable for long-term iNSCs proliferation and culture. Moreover, the cells grew faster in 1.0% hydrogel initially, suggesting this stiffness was more suitable for the rapid adhesion of iNSCs. Similar results showed 1.0% HA hydrogel mimicked the mechanical behavior of brain tissue, and favored NSCs differentiation to neurons (12) and effectively supporting NSC growth (13).
Stiffness has been proven to play an important role in inducing the differentiation of neural crest stem cells (NCSCs) (8). Our results found that iNSCs in soft “brain-like” HA hydrogel could differentiate into neurons, astrocytes, and oligodendrocytes, and the stiffness of hydrogel mediated the differentiation direction and rate. The stiffness of the hydrogels was similar to that of brain tissue, which will be helpful to induce iNSCs to form the neural lineages. In 0.8% hydrogel, the neurons and the ratio of neurons/glial cells were the highest, suggesting that a soft matrix is more suitable for iNSCs differentiating into neurons. In 1.8% hydrogel, iNSCs were prone to maintain stem cell features with a low differentiation rate and showed a tendency to differentiate into glial cells. Another study showed iPSCs derived NSC can separately differentiate into mesenchymal cells and glias on stiff and soft surface mimicking the vascular environment (8). So it can be seen that stiffness in microenvironment plays a decisive role in iNSCs differentiation. As a mechanical stimulus, stiffness might interact with the integrins on the cell membrane and affect cell functions directly (10). The mechanical stimulation sensor of the cell membrane then interacts and transduces the mechanical signal into the NSC differentiation program (14), suggesting stiffness could effectively and individually regulate the proliferation and differentiation of iNSCs.
Conclusions
Our study showed that HA hydrogel stiffness affected the proliferation and differentiation of iNSCs. These results suggested the physical microenvironment, especially stiffness, could be used as an effective and secure strategy to regulate iNSCs and improve their survival and differentiation for future neural repair.
Acknowledgments
Funding: The authors acknowledge the financial support from the National Foundation for Natural Scientific Research of China (No. 61827809) and Research Funding of Capital Medical University for Undergraduate Students (No. XSKY2020005, D2KT2021018).
Footnote
Reporting Checklist: The authors have completed the MDAR reporting checklist. Available at https://dx.doi.org/10.21037/atm-21-6189
Data Sharing Statement: Available at https://dx.doi.org/10.21037/atm-21-6189
Conflicts of Interest: All authors have completed the ICMJE uniform disclosure form (available at https://dx.doi.org/10.21037/atm-21-6189). The authors have no conflicts of interest to declare.
Ethical Statement: The authors are accountable for all aspects of the work in ensuring that questions related to the accuracy or integrity of any part of the work are appropriately investigated and resolved.
Open Access Statement: This is an Open Access article distributed in accordance with the Creative Commons Attribution-NonCommercial-NoDerivs 4.0 International License (CC BY-NC-ND 4.0), which permits the non-commercial replication and distribution of the article with the strict proviso that no changes or edits are made and the original work is properly cited (including links to both the formal publication through the relevant DOI and the license). See: https://creativecommons.org/licenses/by-nc-nd/4.0/.
References
- Saha K, Keung AJ, Irwin EF, et al. Substrate modulus directs neural stem cell behavior. Biophys J 2008;95:4426-38. [Crossref] [PubMed]
- Omrani MR, Yaqubi M, Mohammadnia A. Transcription Factors in Regulatory and Protein Subnetworks during Generation of Neural Stem Cells and Neurons from Direct Reprogramming of Non-fibroblastic Cell Sources. Neuroscience 2018;380:63-77. [Crossref] [PubMed]
- Lange C, Turrero Garcia M, Decimo I, et al. Relief of hypoxia by angiogenesis promotes neural stem cell differentiation by targeting glycolysis. EMBO J 2016;35:924-41. [Crossref] [PubMed]
- Morita Y, Kawase N, Ju Y, et al. Mesenchymal stem cell-induced 3D displacement field of cell-adhesion matrices with differing elasticities. J Mech Behav Biomed Mater 2016;60:394-400. [Crossref] [PubMed]
- Rammensee S, Kang MS, Georgiou K, et al. Dynamics of Mechanosensitive Neural Stem Cell Differentiation. Stem Cells 2017;35:497-506. [Crossref] [PubMed]
- Engler AJ, Sen S, Sweeney HL, et al. Matrix elasticity directs stem cell lineage specification. Cell 2006;126:677-89. [Crossref] [PubMed]
- García-Parra P, Maroto M, Cavaliere F, et al. A neural extracellular matrix-based method for in vitro hippocampal neuron culture and dopaminergic differentiation of neural stem cells. BMC Neurosci 2013;14:48. [Crossref] [PubMed]
- Zhu Y, Li X, Janairo RRR, et al. Matrix stiffness modulates the differentiation of neural crest stem cells in vivo. J Cell Physiol 2019;234:7569-78. [Crossref] [PubMed]
- Yuan Y, Tang X, Bai YF, et al. Dopaminergic precursors differentiated from human blood-derived induced neural stem cells improve symptoms of a mouse Parkinson's disease model. Theranostics 2018;8:4679-94. [Crossref] [PubMed]
- Iwashita M, Ohta H, Fujisawa T, et al. Brain-stiffness-mimicking tilapia collagen gel promotes the induction of dorsal cortical neurons from human pluripotent stem cells. Sci Rep 2019;9:3068. [Crossref] [PubMed]
- Wight TN. Provisional matrix: A role for versican and hyaluronan. Matrix Biol 2017;60-61:38-56. [Crossref] [PubMed]
- Wang TW, Spector M. Development of hyaluronic acid-based scaffolds for brain tissue engineering. Acta Biomater 2009;5:2371-84. [Crossref] [PubMed]
- Ren Y, Zhou Z, Cui F, et al. Hyaluronic Acid/Polylysine Hydrogel as a Transfer System for Transplantation of Neural Stem Cells. J Bioact Compat Pol 2009;24:56-62. [Crossref]
- Dupont S, Morsut L, Aragona M, et al. Role of YAP/TAZ in mechanotransduction. Nature 2011;474:179-83. [Crossref] [PubMed]
(English Language Editor: B. Draper)