The expression of HOXC10 is correlated with tumor-infiltrating immune cells in basal-like breast cancer and serves as a prognostic biomarker
Introduction
Breast cancer, which has the highest incidence of cancers in women and is the second leading cause of cancer-related deaths in women, is a heterogeneous disease with different biological and histological characteristics that lead to distinct prognoses and respond differently to specific therapies (1,2). Based on gene expression profiling, breast cancer can be divided into the following 5 intrinsic subtypes: normal-like, luminal A, luminal B, human epidermal growth factor receptor 2-enriched (Her-2+), and basal-like breast cancer (BLBC) (3). BLBC was so named because its gene expression profile is similar to that of basal cells (4,5). In clinical settings, the majority of BLBCs are triple-negative breast cancers, and estrogen receptor (ER), progesterone receptor (PR), and Her-2 are all negatively diagnosed by immunohistochemistry (6). As the most aggressive subtype of breast cancer, BLBC is characterized by early distant metastasis, a high resistant rate, and poor outcomes which comprises 8% to 20% of all breast cancer (7,8). However, as the molecular mechanisms underlying the aggressive characteristics of BLBC are not well understood, identifying new biomarkers for predicting patients’ prognoses and providing targets for effective targeted therapies remain challenging. BLBC has been classified as basal-like immune activated (BLIA), basal-like immune-suppressed (BLIS), luminal androgen receptor (LAR) and mesenchymal (MES), suggesting potential immune therapeutic targets for BLBC. Thus, it is necessary to clarify the relationship between BLBC and immune invasion, and find an immune related biomarker to indicate the prognosis of BLBC.
Homeobox genes (HOXs) make important contributions to cell differentiation and morphogenesis in multicellular organisms by encoding a family of evolutionarily conserved transcription factors that bind to the promoters of target genes (9). In mammals, 39-gene HOX clusters reside in 4 separate chromosomal linkage groups designated as homeobox gene A (HOXA), homeobox gene B (HOXB), homeobox gene C (HOXC), and homeobox gene D (HOXD) that share identical organization (10). It is confirmed that HOX genes act as oncogenes or tumor suppressors in the occurrence and progression of many different cancers (11-14). HOXC genes are located on chromosome 12q13.3 (15).
Homeobox gene C10 (HOXC10), a member of the HOXC clusters, participates in the assembly of replicative complexes, the development of the spinal cord, and the formation of neurons by encoding a transcription factor containing a highly conserved DNA binding homeodomain (16-18). There is increasing evidence that HOXC10 plays a vital role in the occurrence and development of several cancers by influencing cancer cell proliferation, apoptosis, invasion and avoidance of immune destruction (19-21). Zhai et al. (22) analyzed normal cervical epithelial tissue, cervical intraepithelial neoplasia, and invasive squamous cell carcinoma tissues by gene chip and found that HOXC10 expression was upregulated with the increase of cervical epithelial malignancy, which confirmed that HOXC10 protein was related to the progression of cervical cancer in vivo and in vitro. Researchers have also found that the high expression of HOXC10 protein is closely related to the poor prognosis of thyroid cancer (23). In breast cancer research, it has been shown that HOXC10 is highly expressed in breast cancer and also an estrogen-responsive gene which might be associated with hormone-induced cell differentiation, development and human diseases (18). However, the effects and mechanisms underlying HOXC10 in the prognoses of different subtypes of breast cancer, especially BLBC, remain unclear.
In the present study, the different expression levels of HOXC10 in different cancer and normal tissues were assessed by the Oncomine and Tumor Immune Estimation Resource (TIMER) databases. The correlations between HOXC10 and the prognoses of cancer patients, including those with different subtypes of breast cancer, were analyzed using the PrognoScan, Gene Expression Profiling Interactive Analysis (GEPIA) and Kaplan-Meier plotter databases. Further, the relationship between HOXC10 and the immune-infiltration levels of immune cells in different tumor microenvironments were investigated in the TIMER database. The findings of this study shed light on the predictive role of HOXC10 in the prognoses of patients with BLBC and also identify a potential mechanism related to immune infiltration.
We present the following article in accordance with the REMARK reporting checklist (available at https://atm.amegroups.com/article/view/10.21037/atm-21-6611/rc).
Methods
Analysis in the Oncomine database
The HOXC10 expression of various types of cancers was identified in the Oncomine database (https://www.oncomine.org/resource/login.html) (24). The threshold was determined according to the following values: a P value of 0.001, a fold change of 1.5, and the gene ranking of all. The data came from messenger ribonucleic acid (mRNA).
Survival analyses in the PrognoScan, GEPIA, and Kaplan-Meier plotter databases
To investigate the effects of HOXC10 in the prognosis of different types of cancer, the correlations between HOXC10 expression levels and the survival of cancer patients were analyzed using the PrognoScan (http://www.abren.net/PrognoScan/) (25), GEPIA (http://gepia.cancer-pku.cn/index.html) (26), and Kaplan-Meier plotter databases (http://kmplot.com/analysis/) (27). The Kaplan-Meier plotter database were analyzed by filtering the intrinsic subtypes of breast cancer including BLBC to evaluate the relationship between HOXC10 expression and the outcomes of breast cancer patients with different intrinsic subtypes. The threshold was determined according to the following values: a Cox P value <0.05 in the PrognoScan database, and a log-rank P value <0.05 in the GEPIA and Kaplan-Meier plotter databases. The study was conducted in accordance with the Declaration of Helsinki (as revised in 2013).
Correlation analyses between HOXC10 and immune cells in the TIMER, GEPIA, and Kaplan-Meier plotter databases
To systematically analyze the levels of immune invasion in diverse types of cancers and differences in the gene expression of different cancers, we used the TIMER database (https://cistrome.shinyapps.io/timer/) to evaluate data from The Cancer Genome Atlas (TCGA), which included 10,897 samples across 32 cancer types (28,29). First, we confirmed the expression of HOXC10 across data from 32 cancer tissues compared to data from paired normal tissues using the TIMER database. Second, we investigated the correlations of HOXC10 with typical infiltrating immune cells [i.e., B cells, cluster of differentiation (CD)4+ T cells, CD8+ T cells, neutrophils, macrophages, and dendritic cells (DCs)] using the TIMER algorithm. Third, we further analyzed the correlations between HOXC10 and genetic markers of immune cells in breast invasive carcinoma (BRCA) and different intrinsic subtypes of BRCA via correlation modules in the TIMER database. The gene markers of tumor-infiltrating immune cells included the markers of B cells, CD8+ T cells, general T cells, neutrophils, natural killer (NK) cells, monocytes, tumor-associated macrophages (TAMs), M1 macrophages, M2 macrophages, DCs, T-helper 1 (Th1) cells, T-helper (Th2) cells, follicular helper T (Tfh) cells, Th17 cells, regulatory cells (Tregs), and T cell exhaustion as described in previous studies (30,31).
The comprehensive GEPIA database, which comprises 9,736 tumor samples and 8,587 normal samples from TCGA and the Genotype-Tissue Expression Project (GTEx) programs (26), was also used to further confirm the significantly correlated genes in TIMER, which analyzes RNA-sequencing expression. The Spearman’s correlation test was selected, and all other default settings were accepted before analyzing the gene correlations.
Finally, to confirm the correlations between HOXC10 and biomarkers of immune cells in BRCA, we used a pan-cancer analysis of the Kaplan-Meier plotter database to export the different outcomes of patients with BRCA based on different levels of immune cells. The automatic best performing threshold was used as the cutoff. The results displayed the false discovery rates in addition to the P values.
Statistical analysis
The results of the Oncomine analysis are displayed with their P values, fold changes, and ranks. The survival curves were generated by the PrognoScan, GEPIA, and Kaplan-Meier plotter databases, and were displayed with the hazard ratio (HR) and P or Cox P values from the log-rank tests. The Spearman’s correlation analysis and statistical significance results were used to evaluate correlations between HOXC10 and immune infiltration or type markers of immune cells. For all the analyses in the present study, a P value <0.05 was considered statistically significant.
Results
The mRNA expression levels of HOXC10 in different human cancers
To study changes in HOXC10 expression between normal and tumor tissues, the HOXC10 mRNA levels of different tumors and normal tissues of multiple cancer types were analyzed first using the Oncomine database, from which 147 data sets were selected, including 30,079 samples. The analysis showed that the expression levels of HOXC10 were significantly higher in brain and central nervous system (CNS), breast, cervical, colorectal, esophageal, gastric, lung and sarcoma cancers tissues than normal tissues (see Figure 1A). Additionally, the expression levels of HOXC10 were lower in kidney, melanoma, and ovarian cancer tissues than normal tissues in some data sets. The detailed results of HOXC10 expression in different cancer types are summarized in Table S1.
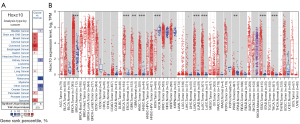
To further evaluate HOXC10 expression in human cancers, we analyzed the expression levels of HOXC10 between different tumor and normal tissues based on the RNA-sequencing data of multiple malignancies in TCGA database. Figure 1B shows the differential expression of HOXC10 between the tumor and adjacent normal tissues across all TCGA tumors. The expression levels were significantly higher in BRCA, esophageal carcinoma (ESCA), glioblastoma multiforme (GBM), head and neck squamous cell carcinoma (HNSC), lung adenocarcinoma (LUAD), lung squamous cell carcinoma (LUSC), stomach adenocarcinoma (STAD), and thyroid carcinoma (THCA) tissues than adjacent normal tissues. However, HOXC10 expression was significantly lower in kidney chromophobe (KICH) and prostate adenocarcinoma (PRAD) tissues than adjacent normal tissues (see Figure 1B).
Predictive effect of HOXC10 in the prognosis of patients with different cancers
Based on the differences in HOXC10 expression between tumor and normal tissues, we first analyzed the associations between HOXC10 and survival across different cancers using the PrognoScan database (see Table S2). Only Cox P values <0.05 are shown in Figure 2A. HOXC10 expression significantly affected the prognosis of 3 type cancers (i.e., breast, brain and ovarian cancer). GSE1378 and GSE1379, 2 cohorts of 60 patients with breast cancer, respectively, indicated that a high level of HOXC10 expression was significantly associated with a high risk of relapse in breast cancer patient [GSE1378, relapse-free survival (RFS) HR =1.27%, 95% confidence interval (CI): 1.03–1.56, Cox P=0.024856; GSE1379, RFS HR =1.25%, 95% CI: 1.01–1.54, Cox P=0.036064; see Figure 2B,2C]. Further, the high expression of HOXC10 in ovarian cancer and brain cancer was related to a poor prognosis (see Figure 2D-2F).
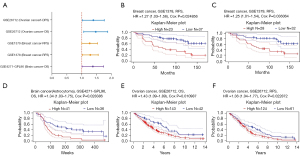
Second, we used GEPIA database to analyze TCGA data to evaluate the predictive value of HOXC10 in human cancers. Notably, the high or low expression of HOXC10 made no significant difference in the disease-free survival (DFS) of BRCA patients. However, BRCA patients with a low expression of HOXC10 had better overall survival (OS) than those with a high expression of HOXC10 (see Figure S1A). In addition, a high expression of HOXC10 in adrenocortical carcinoma (ACC), colon adenocarcinoma (COAD), and stomach adenocarcinoma (STAD) was correlated to poor DFS and OS (see Figure S1B-D). However, the high expression of HOXC10 in kidney renal clear cell carcinoma (KIRC) indicated a favorable prognosis (see Figure S1E).
Third, we analyzed large databases, including the Gene Expression Omnibus (GEO), European Genome-Phenome Archive (EGA), and TCGA databases, using the Kaplan-Meier plotter database to explore the potential prognostic relationship between HOXC10 and different cancers (see Figure S2). In breast cancer, the median OS and post-progression survival (PPS) times of patients with lower levels of HOXC10 expression were 120 and 43.2 months, respectively, while that of patients with higher levels of HOXC10 expression were 85.2 and 26.8 months, respectively, and the differences were significant (P=0.00353 and P=0.03, respectively; see Figure S2A). The same phenomenon was observed in lung cancer and gastric cancer (see Figure S2C,D). Conversely, a higher expression of HOCX10 was related to better RFS in ovarian cancer patients (P=0.038; see Figure S2B).
Prognostic potential of HOXC10 in BLBC
The above-mentioned 3 analyses confirmed that the expression level of HOXC10 could be used to predict the prognosis of patients with breast cancer. To further evaluate the relationship between HOXC10 expression and the outcomes of breast cancer patients with different intrinsic subtypes, the Kaplan-Meier plotter database was analyzed by filtering the intrinsic subtype of breast cancer. There was no significant difference between patients with high HOXC10 expression and those with low HOXC10 expression in luminal A, luminal B, and Her-2 + breast cancer (see Figure S3). Notably, the median OS of patients with high and low HOXC10 expression in BLBC was 55.4 and 115 months, respectively, and the difference was significant (HR =1.97%, 95% CI: 1–3.87; P=0.045; see Figure 3A). The same prognostic effect of HOXC10 was also found in relation to the PPS of BLBC patients (HR =2.14%, 95% CI: 1.02–4.47; P=0.04; see Figure 3B). There is no significant difference in RFS and DMFS (See Figure 3C,3D). The analysis results showed that the increased expression of HOXC10 in BLBC was related to a poor prognosis, which indicates that HOXC10 can serve as a biomarker for the prognosis of BLBC patients.
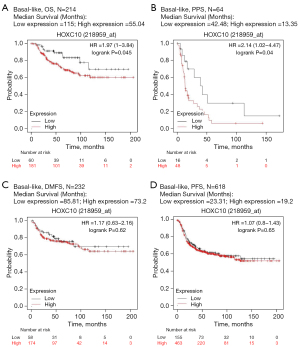
HOXC10 expression affects the survival time of lymph-node positive or grade III breast cancer patients
In addition to the different intrinsic subtypes that could affect the prognosis, many clinicopathological factors are related to the survival of patients with breast cancer. We used the Kaplan-Meier plotter database to examine the relationships between HOXC10 and the prognosis of breast cancer with different clinicopathological characteristics (see Table 1). Notably, the elevated expression of HOXC10 had adverse effects on both the OS and PPS of lymph-node positive breast cancer patients (OS: HR =1.79%, 95% CI: 1.09–2.94; P=0.0206; PPS: HR =1.39%, 95% CI: 1.09–1.77; P=0.0072). Moreover, high HOXC10 expression negatively affected the OS of grade III breast cancer patients (HR =1.55%, 95% CI: 1.03–2.33; P=0.0034). Thus, HOXC10 appears to affect the prognosis of lymph-node positive or advanced-stage (i.e., grade III) breast cancer patients.
Table 1
Clinicopathological characteristics | Overall survival (n=1,402) | Progression-free survival (n=3,955) | |||||
---|---|---|---|---|---|---|---|
N | Hazard ratio | P value | N | Hazard ratio | P value | ||
ER status | |||||||
ER positive | 548 | 1.06 (0.71–1.58) | 0.7903 | 2061 | 1.03 (0.85–1.25) | 0.7426 | |
ER negative | 251 | 1.31 (0.77–2.23) | 0.3143 | 801 | 1.17 (0.9–1.52) | 0.2398 | |
PR status | |||||||
PR positive | 83 | 0.61 (0.15–2.46) | 0.4857 | 589 | 1.02 (0.69–1.51) | 0.9309 | |
PR negative | 89 | 0.86 (0.31–2.42) | 0.7761 | 549 | 1.32 (0.93–1.87) | 0.121 | |
Her-2 status | |||||||
Her-2 positive | 129 | 0.79 (0.38–1.64) | 0.5245 | 252 | 0.9 (0.55–1.45) | 0.6581 | |
Her-2 negative | 130 | 0.78 (0.31–1.94) | 0.5876 | 800 | 1.17 (0.86–1.6) | 0.3078 | |
Lymph-node status | |||||||
LN positive | 313 | 1.79 (1.09–2.94) | 0.0206 | 1133 | 1.39 (1.09–1.77) | 0.0072 | |
LN negative | 594 | 0.89 (0.59–1.34) | 0.5738 | 2020 | 1.06 (0.87–1.29) | 0.5466 | |
Grade | |||||||
I | 161 | 0.61 (0.23–1.58) | 0.3049 | 345 | 1 (0.55–1.83) | 0.99 | |
II | 387 | 1.05 (0.64–1.7) | 0.85 | 901 | 1.13 (0.85–1.5) | 0.4049 | |
III | 503 | 1.55 (1.03–2.33) | 0.034 | 903 | 1.19 (0.92–1.54) | 0.1792 | |
TP53 status | |||||||
Mutated | 111 | 1.5 (0.57–3.97) | 0.4106 | 188 | 1.72 (0.92–3.2) | 0.0851 | |
Wild type | 187 | 0.65 (0.32–1.29) | 0.2102 | 273 | 1.09 (0.66–1.79) | 0.7383 |
ER, estrogen receptor; PR, progesterone receptor; Her-2, human epidermal growth factor receptor 2; LN, lymph node; TP53, tumor protein p53.
The transcription of HOXC10 is correlated with the immune-infiltration level and immune marker set in BLBC
Tumor-infiltrating lymphocytes are independent predictors of the sentinel lymph-node status and survival of cancer patients (31). Previous research has shown that tumor infiltration is related to the prognosis of breast cancer patients (32-34). The effect of HOXC10 transcription on the recruitment of immune cells and the cancer microenvironment remains unclear. Thus, we investigated the relationship between the immune-infiltration and transcription levels of HOXC10 in different types of cancer (see Figure S4), including different subtypes of breast cancer (see Figure 4) using the TIMER database to understand the mechanism underlying HOXC10 and the outcomes of breast cancer patients. There were no significant correlations between immune-infiltration of B cells, CD8+ T cells, CD4+ T cells, macrophages, neutrophils, DCs and transcription levels of HOXC10 in BRCA (see Figure 4A) and luminal breast cancer (see Figure 4D). However, HOXC10 was positively correlated with the immune infiltration of macrophages in BLBC (Cor =0.207; P=0.0198; see Figure 4B), but was negatively correlated with the immune infiltration of macrophages in Her-2+ breast cancer (Cor = –0.276; P=0.0357; see Figure 4C).
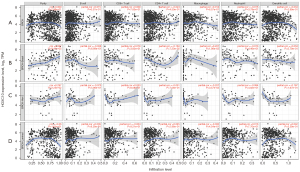
Further, the relationships between HOXC10 expression levels and the genetic markers of different immune cells, including B cells, CD8+ T cells, general T cells, neutrophils, NK cells, monocytes, TAMs, M1 macrophages, M2 macrophages, DCs, Th1 cells, Th2 cells, Tfh cells, Th17 cells, Treg, and T cell exhaustion in BRCA were explored using the TIMER database, which showed that there were no significant correlations between HOXC10 and these biomarkers (see Figure 5 and Table 2). Next, the GEPIA database was used to explore the relationship between the biomarkers of monocytes, TAMs, M1 and M2 macrophage and HOXC10 expression in BRCA (see Figure S5). The results showed that the CD86, macrophage-colony stimulating factor 1 receptor (CSF1R) of monocytes, the chemokine (C-C motif) ligand 2 (CCL2) of TAMs, the CD163, v-set and immunoglobulin domain–containing 4 (VSIG4), and membrane spanning 4-domains A4A (MS4A4A) of M2 macrophages were negatively correlated with HOXC10 expression in BRCA.
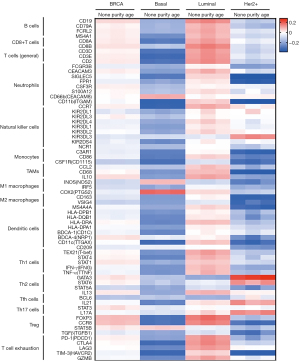
Table 2
Description | Gene markers | Breast cancer | BLBC | |||||||||
---|---|---|---|---|---|---|---|---|---|---|---|---|
None | Purity | None | Purity | |||||||||
COR | P | COR | P | COR | P | COR | P | |||||
B cells | CD19 | 0.0465 | 0.12308 | 0.0503 | 0.11279 | –0.172 | 0.04211 | –0.1652 | 0.06136 | |||
CD79A | 0.0138 | 0.64678 | 0.0227 | 0.47361 | –0.1649 | 0.05163 | –0.1511 | 0.08733 | ||||
FCRL2 | 0.0207 | 0.49323 | 0.0292 | 0.35728 | –0.161 | 0.05743 | –0.1554 | 0.07873 | ||||
MS4A1 | 0.0188 | 0.53424 | 0.0286 | 0.36739 | –0.2399 | 0.0043 | –0.2414 | 0.00585 | ||||
CD8+ T cells | CD8A | 0.0242 | 0.42181 | 0.0309 | 0.33097 | –0.2799 | 0.00085 | –0.2709 | 0.00196 | |||
CD8B | 0.0363 | 0.22867 | 0.0387 | 0.22265 | –0.1258 | 0.13842 | –0.1072 | 0.22644 | ||||
T cells (general) | CD3D | 0.0117 | 0.69912 | 0.016 | 0.61489 | –0.2878 | 0.00059 | –0.2829 | 0.00121 | |||
CD3E | 0.021 | 0.48641 | 0.0258 | 0.41581 | –0.3114 | 0.00019 | –0.3066 | 0.00043 | ||||
CD2 | 0.0252 | 0.40456 | 0.0291 | 0.35933 | –0.2909 | 0.00051 | –0.2858 | 0.00107 | ||||
Neutrophils | FCGR3B | –0.034 | 0.26644 | –0.0377 | 0.23494 | –0.1739 | 0.03987 | –0.1804 | 0.04076 | |||
CEACAM3 | 0.0183 | 0.54328 | 0.026 | 0.41242 | –0.2023 | 0.01651 | –0.2066 | 0.01885 | ||||
SIGLEC5 | –0.012 | 0.6846 | –0.0172 | 0.5872 | –0.2804 | 0.00083 | –0.2746 | 0.00169 | ||||
FPR1 | –0.036 | 0.23721 | –0.0355 | 0.26384 | –0.2003 | 0.01777 | –0.1853 | 0.03568 | ||||
CSF3R | 0.0216 | 0.47348 | 0.0183 | 0.56378 | –0.1501 | 0.07673 | –0.1374 | 0.12035 | ||||
S100A12 | 0.0048 | 0.87368 | 0.0098 | 0.75699 | –0.1344 | 0.11347 | –0.12 | 0.17561 | ||||
CEACAM8 | –0.005 | 0.86922 | 0.0104 | 0.7435 | 0.1001 | 0.23947 | 0.10664 | 0.22904 | ||||
ITGAM | –0.007 | 0.82108 | –0.0196 | 0.53738 | –0.3327 | 6.49E–05 | –0.3285 | 0.00016 | ||||
CCR7 | 0.0193 | 0.52247 | 0.0213 | 0.50218 | –0.3262 | 9.14E–05 | –0.3249 | 0.00019 | ||||
Natural killer cells | KIR2DL1 | –0.061 | 0.04302 | –0.0382 | 0.22844 | –0.1815 | 0.03183 | –0.1982 | 0.02435 | |||
KIR2DL3 | 0.0073 | 0.8086 | 0.024 | 0.44993 | –0.125 | 0.14118 | –0.1431 | 0.10572 | ||||
KIR2DL4 | –0.035 | 0.24025 | –0.0307 | 0.33266 | –0.1852 | 0.02849 | –0.1901 | 0.03092 | ||||
KIR3DL1 | –0.027 | 0.37454 | –0.0272 | 0.39137 | –0.2063 | 0.01448 | –0.2099 | 0.01696 | ||||
KIR3DL2 | –0.01 | 0.74839 | 0.0031 | 0.92324 | –0.1862 | 0.02758 | –0.1939 | 0.0277 | ||||
KIR3DL3 | –0.057 | 0.00702 | –0.0469 | 0.0364 | –0.0763 | 0.20286 | –0.0786 | 0.2083 | ||||
KIR2DS4 | –0.044 | 0.14704 | –0.0237 | 0.45493 | –0.2407 | 0.00417 | –0.2528 | 0.00385 | ||||
NCR1 | –0.038 | 0.20267 | –0.0369 | 0.24511 | –0.1494 | 0.07804 | –0.1478 | 0.09458 | ||||
Monocytes | C3AR1 | –0.039 | 0.19612 | –0.0422 | 0.18389 | –0.2644 | 0.00165 | –0.2638 | 0.0026 | |||
CD86 | –0.028 | 0.34912 | –0.0299 | 0.34683 | –0.2717 | 0.00121 | –0.2669 | 0.0023 | ||||
CSF1R | –0.112 | 0.00021 | –0.1096 | 0.0005 | –0.2974 | 0.00038 | –0.2959 | 0.0007 | ||||
TAMs | CCL2 | –0.027 | 0.36706 | –0.0231 | 0.46604 | –0.1282 | 0.13095 | –0.1297 | 0.14264 | |||
CD68 | 0.0105 | 0.72878 | 0.0033 | 0.91686 | –0.2297 | 0.00643 | –0.2253 | 0.01038 | ||||
IL10 | 0.0428 | 0.15599 | 0.0439 | 0.16637 | –0.2085 | 0.01357 | –0.2019 | 0.0219 | ||||
M1 macrophages | NOS2 | –0.084 | 0.00551 | –0.0871 | 0.006 | 0.0824 | 0.33338 | 0.0881 | 0.32081 | |||
IRF5 | –0.072 | 0.01708 | –0.0742 | 0.0192 | –0.1965 | 0.02011 | –0.1702 | 0.05396 | ||||
PTGS2 | –0.038 | 0.21377 | –0.041 | 0.196 | 0.1884 | 0.02594 | 0.18034 | 0.04097 | ||||
M2 macrophages | CD163 | –0.058 | 0.05648 | –0.0558 | 0.07849 | –0.2163 | 0.0104 | –0.2144 | 0.01482 | |||
VSIG4 | –0.097 | 0.00123 | –0.0978 | 0.002 | –0.2077 | 0.01395 | –0.2056 | 0.01959 | ||||
MS4A4A | –0.019 | 0.5244 | –0.0183 | 0.56427 | –0.1845 | 0.02926 | –0.1854 | 0.0356 | ||||
Dendritic cells | HLA–DPB1 | –0.037 | 0.2167 | –0.0404 | 0.20339 | –0.2307 | 0.00621 | –0.2245 | 0.01067 | |||
HLA–DQB1 | –0.025 | 0.41401 | –0.0405 | 0.20181 | –0.2494 | 0.00304 | –0.2451 | 0.00521 | ||||
HLA–DRA | 0.0105 | 0.72911 | 0.0062 | 0.84426 | –0.2521 | 0.00273 | –0.2544 | 0.00372 | ||||
HLA–DPA1 | –0.015 | 0.62853 | –0.016 | 0.61391 | –0.2102 | 0.0128 | –0.2063 | 0.01914 | ||||
CD1C | –0.025 | 0.40064 | –0.0197 | 0.53471 | –0.2507 | 0.00282 | –0.2557 | 0.00345 | ||||
NRP1 | 0.0331 | 0.27264 | 0.0326 | 0.30492 | –0.0458 | 0.59081 | –0.0313 | 0.7245 | ||||
ITGAX | –0.009 | 0.76693 | –0.0067 | 0.83264 | –0.3097 | 0.00021 | –0.3043 | 0.00048 | ||||
CD209 | –0.043 | 0.1503 | –0.0448 | 0.15788 | –0.0325 | 0.70262 | –0.038 | 0.66897 | ||||
Th1 cells | TBX21 | 0.0062 | 0.83834 | 0.0108 | 0.73436 | –0.2319 | 0.00593 | –0.2268 | 0.00987 | |||
STAT4 | 0.0121 | 0.68963 | 0.0168 | 0.59651 | –0.2586 | 0.0021 | –0.2569 | 0.00338 | ||||
STAT1 | 0.0311 | 0.302 | 0.0318 | 0.31629 | –0.1806 | 0.03288 | –0.1666 | 0.05929 | ||||
IFNG | –0.019 | 0.51824 | –0.0212 | 0.50352 | –0.228 | 0.00674 | –0.229 | 0.00903 | ||||
TNF | –0.03 | 0.31225 | –0.0202 | 0.52431 | –0.227 | 0.0071 | –0.2341 | 0.0077 | ||||
Th2 cells | GATA3 | 0.0266 | 0.37862 | 0.0412 | 0.19378 | –0.1227 | 0.14842 | –0.1324 | 0.13453 | |||
STAT6 | 0.0116 | 0.70183 | 0.0208 | 0.51217 | –0.1279 | 0.1319 | –0.0999 | 0.25956 | ||||
STAT5A | –0.09 | 0.00288 | –0.0804 | 0.0112 | –0.1911 | 0.02387 | –0.1723 | 0.05092 | ||||
IL13 | 0.0287 | 0.342 | 0.0292 | 0.35764 | –0.1848 | 0.0288 | –0.1868 | 0.03401 | ||||
Tfh cells | BCL6 | –0.131 | 1.26E–05 | –0.12 | 0.0001 | –0.1123 | 0.18628 | –0.1259 | 0.15487 | |||
IL21 | –0.015 | 0.61721 | –0.0136 | 0.66722 | –0.2584 | 0.00206 | –0.2468 | 0.00481 | ||||
Th17 cells | STAT3 | 0.0293 | 0.33211 | 0.0473 | 0.13584 | –0.0419 | 0.62252 | –0.0566 | 0.52339 | |||
IL17A | –0.017 | 0.56991 | –0.0114 | 0.71881 | –0.0662 | 0.43707 | –0.0541 | 0.5426 | ||||
Tregs | FOXP3 | 0.0801 | 0.00785 | 0.0874 | 0.0058 | –0.2933 | 0.00046 | –0.2842 | 0.00114 | |||
CCR8 | 0.0756 | 0.01218 | 0.085 | 0.0073 | –0.2948 | 0.00041 | –0.2825 | 0.00118 | ||||
STAT5B | 0.0232 | 0.44267 | 0.0398 | 0.20948 | 0.0554 | 0.51494 | 0.06682 | 0.45131 | ||||
TGFB1 | –0.04 | 0.18603 | –0.0551 | 0.08212 | –0.1915 | 0.02354 | –0.1844 | 0.03652 | ||||
T-cell exhaustion | PDCD1 | 0.016 | 0.59556 | 0.0218 | 0.49221 | –0.2002 | 0.01772 | –0.1935 | 0.028 | |||
CTLA4 | 0.0043 | 0.88702 | 0.0106 | 0.73839 | –0.3091 | 0.00022 | –0.3061 | 0.00044 | ||||
LAG3 | 0.0032 | 0.91633 | –0.001 | 0.97483 | –0.2495 | 0.00303 | –0.2439 | 0.00546 | ||||
HAVCR2 | –0.024 | 0.42419 | –0.0263 | 0.4076 | –0.3194 | 0.00013 | –0.3172 | 0.00027 | ||||
GZMB | –0.017 | 0.56991 | –0.0136 | 0.66762 | –0.2446 | 0.00359 | –0.2426 | 0.0056 |
BLBC, basal-like breast cancer; COR, correlation coefficient; TAM, tumor-associated macrophage; Th, T-helper; Tfh, follicular helper T; Treg, regulatory cells; CD, cluster of differentiation; FCRL2, Fc receptor like 2; MS4A1, membrane spanning 4-domains A1; FCGR3B, Fc gamma receptor IIIb; CEACAM, CEA cell adhesion molecule; SIGLEC5, sialic acid binding Ig like lectin 5; FPR1, formyl peptide receptor 1; CSF3R, colony stimulating factor 3 receptor; S100A12, S100 calcium binding protein A12; ITGAM, ntegrin subunit alpha M; CCR, C-C motif chemokine receptor; KIR2DL, killer cell immunoglobulin like receptor, two Ig domains and long cytoplasmic tail; KIR3DL, killer cell immunoglobulin-like receptor with three domains and long cytoplasmic tail; KIR2DS4, killer cell immunoglobulin like receptor, two Ig domains and short cytoplasmic tail 4; NCR1, natural cytotoxicity triggering receptor 1; C3AR1, complement C3a receptor 1; CSF1R, colony stimulating factor 1 receptor; CCL2, C-C motif chemokine ligand 2; IL, interleukin; NOS2, nitric oxide synthase 2; IRF5, interferon regulatory factor 5; PTGS2, prostaglandin-endoperoxide synthase 2; VSIG4, V-set and immunoglobulin domain containing 4; MS4A4A, membrane spanning 4-domains A4A; HLA-DPB1, major histocompatibility complex, class II, DP beta 1; HLA-DQB1, major histocompatibility complex, class II, DQ beta 1; HLA-DRA, major histocompatibility complex, class II, DR alpha; HLA-DPA1, major histocompatibility complex, class II, DP alpha 1; NRP1, neuropilin 1; ITGAX, integrin subunit alpha X; TBX21, T-box transcription factor 21; STAT, signal transducer and activator of transcription; IFNG, interferon gamma; TNF, tumor necrosis factor; GATA3, GATA binding protein 3; BCL6, B cell leukemia transcription repressor; FOXP3, forkhead box P3; TGFB1, transforming growth factor beta 1; PDCD1, programmed cell death 1; CTLA4, cytotoxic T-lymphocyte associated protein 4; LAG3, lymphocyte activating 3; HAVCR2, hepatitis A virus cellular receptor 2; GZMB, granzyme B.
Further, we explored the correlations between HOXC10 and genetic markers in different subtypes of BRCA. As Figure 5 shows, in contrast to its positive correlation with HOXC10 expression in luminal breast cancer, the correlations between genetic markers of immune cells in BLBC and Her-2+ were mostly negative (see Table 2 and Table S3). Specifically, after the correlation adjustment by purity, the complement C3a receptor 1 (C3AR1), CD86 and CSF1R of monocytes, the CD68 and IL10 of TAMs, the CD163, VSIG4 and MS4A4A of M2 macrophage, and the interferon regulatory factor 5 (IRF5) of M1 macrophages were found to be significantly correlated with the expression levels of HOXC10 in BLBC (see Table 2). Thus, HOXC10 appears to be closely related to macrophage polarization in BLBC, which further confirms that expressions in BLBC are correlated to immune infiltration.
Prognostic effect of HOXC10 expressions in BRCA based on immune cells
Both the TIMER and GEPIA databases were used to analyze the relationship between HOXC10 and biomarkers of immune cells in BRCA, but the results differed. To confirm the correlation, we used the Kaplan-Meier plotter database to analyze the relationship between HOXC10 and the OS of patients with BRCA based on different levels of immune cells. The results showed that high HOXC10 expressions of BRCA in enriched CD4+ memory T cells (HR =1.6, P=0.024), enriched macrophages (HR =1.71, P=0.034), enriched NK cells (HR =2.07, P=0.032), and enriched Th2 cells (HR =1.83, P=0.029) was negatively correlated with OS time, while the high expression of HOXC10 had no significant correlation with OS in decreased subgroups of the immune-cells (see Figure 6). There was no significant correlation between high HOXC10 and the outcomes of BRCA patients with enriched B cells, regulatory T cells, and Th1 cells. However, compared to the low HOXC10 expression group, the high HOXC10 expression group had a poorer prognosis regardless of whether or not they had enriched or decreased CD8+ memory T cells, eosinophils, and MES stem cells (see Figure S6). We also analyzed the prognostic potential of RFS in BRCA based on the different immune-cell subgroup (see Figure S7). All the results indicated that a high level of HOXC10 affects the survival of BRCA patients, which may be due to the infiltration of some immune cells.
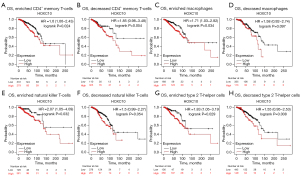
Discussion
HOXC10, which is known to regulate the rib formation of mammals (35,36), is a key regulator in the development, proliferation, invasion, and metastasis of cancer cells in lung cancer (19), glioma (20), gastric cancer (37), thyroid cancer (23), and squamous cell carcinomas (22). In the present study, we examined the abnormal expression and predictive effect of HOXC10 in different types of cancers, especially in different subtypes of breast cancer. We also evaluated the correlations between HOCX10 and cancer immune infiltrates to clarify whether HOXC10 affected the prognosis of breast cancer patients.
To examine the effects of HOXC10 on different types of cancer, we used 2 independent online databases (i.e., Oncomine and TIMER) to analyze the differential expression of HOXC10 in tumor and adjacent normal tissues. The Oncomine analysis showed that the expression levels of HOXC10 were significantly higher in brain and CNS, breast, cervical, colorectal, esophageal, gastric, lung, and sarcoma cancer tissues than normal tissues (see Figure 1A). Conversely, the expression of HOXC10 was observed to be lower in kidney, melanoma, and ovarian cancer tissues than normal tissues in some data sets. The results of the TIMER database analysis indicated that HOXC10 expression was significantly higher in BRCA, ESCA, GBM, HNSC, LUAD, LUSC, STAD, and THCA tissues than adjacent normal tissues, while HOXC10 expression was significantly lower in KICH and PRAD tissues than adjacent normal tissues (see Figure 1B). To explore the systematic prognostic landscape of HOXC10 in patients with different types of cancer, we used the PrognoScan, GEPIA, and Kaplan-Meier plotter databases to compare the different survival times of patients with high or low expression levels of HOXC10. Our results suggested that HOXC10 may play opposite roles depending on the cancer cell type. Similarly, the overexpression of HOXC10, as a proto-oncogene, has been found to be correlated with the poor prognosis of patients with esophageal squamous cell carcinoma (ESCC) (38), and gastric cancer (39). We found that the higher expression of HOCX10 was related to better RFS in ovarian cancer patients (P=0.038; see Figure S2B).
In breast cancer research, it has been shown that HOXC10 is highly expressed in breast cancer patients (18,40). Similarly, all the analyses of the online Oncomine, TIMER, PrognoScan, GEPIA and Kaplan-Meier plotter databases indicated that HOXC10 acts as an oncogene in breast cancer, and the higher expression of this marker is related to a poor prognosis (see Figures 1,2). In drug-resistance research, HOXC10 has been reported to contribute to the chemotherapy resistance of breast cancer via DNA repair by binding to cyclin-dependent kinase 7 and activating the nuclear factor kappa B (NF-κB) pathway (40). Moreover, HOXC10, the promoter of which contains the ER response element, was also involved in the aromatase inhibitor-resistance of ER positive breast cancer via the regulation of ERα and ERβ (41). The activity of HOXC10 is upregulated through estrogen by recruiting the mixed-lineage leukemia (MLL) 3 and MLL4 to the estrogen response factor in the promoter region of HOXC10 (18). In addition, it has been confirmed that the high expression of the HOXC10 protein is related to the poor prognosis of ER-negative breast cancer patients (40). However, the specific role of HOXC10 in BLBC remains poorly understood. The Kaplan-Meier plotter database was analyzed by filtering the intrinsic subtype of breast cancer to evaluate the relationship between HOXC10 expression and the outcomes of patients with BLBC. The median OS of BLBC patients with high HOXC10 expression was significantly shorter than that of those with low expression (HR =1.97%, 95% CI: 1–3.87; P=0.045; see Figure 3A). The prognostic effect of HOXC10 was also shown in relation to the PPS of BLBC patients (HR =2.14%, 95% CI: 1.02–4.47, P=0.04; see Figure 3B), which indicates that HOXC10 could serve as a biomarker for predicting the prognosis of BLBC patients.
In our results, the analysis of the Kaplan-Meier plotter database based on different intrinsic subtypes of breast cancer showed that the elevated expression of HOXC10 had adverse effects on both OS and PPS in lymph-node positive breast cancer patients (P=0.0206 and P=0.0072, respectively), which indicates that tumor infiltration might be related to the prognosis of breast cancer. Some researchers have compared the expression levels of HOXC10 between lymph-node positive and lymph-node negative breast cancer and found that HOXC10 expression was upregulated in patients with positive lymph nodes (42). The related prognosis and possible immune mechanisms of HOXC10 in BRCA, especially in BLBC, are still ambiguous. Thus, we investigated whether HOXC10 was positively correlated to the immune infiltration of macrophages in BLBC (see Figure 4B). The C3AR1, CD86 and CSF1R of monocytes, the CD68 and IL10 of TAMs, the CD163, VSIG4 and MS4A4A of M2 macrophages, and the IRF5 of M1 macrophages were significantly correlated with the expression levels of HOXC10 in BLBC (see Table 2). Thus, HOXC10 appears to be closely related to macrophage polarization in BLBC, which further confirms that expressions in BLBC are correlated to immune infiltration. Recent studies have examined the possible mechanisms by which HOXC10 is related to the immune infiltration and the poor prognosis of patients with different types of cancer. In glioma cells, HOXC10 was found to induce the expression of immunosuppressive genes, including transforming growth factor-β (TGF-β) programmed death ligand 2, CCL2, and tryptophan 2,3-dioxygenase (20). When the expression of HOXC10 in gastric cancer cells was overexpressed by transfected overexpression plasmids in vitro and in vivo, the expression of interleukin-6, tumor necrosis factor-α (TNF-α), TGF-β, and the epidermal growth factor related to the tumor microenvironment were increased (43). In hepatocellular carcinoma, interleukin-1β was found to induce the expression of HOXC10, which in turn promoted metastasis by upregulating 3-phosphoinositide dependent protein kinase-1 and vasodilator-stimulated phosphoprotein expression (44). Li et al. found that HOXC10 interacted with its antisense transcript partner lncHOXC-AS3 to regulate the osteogenesis of MES stromal cells derived from the bone marrow of patients with multiple myeloma (45). In oral squamous cell carcinoma, HOXC10 was found to induce migration and invasion by regulating the drosophila wingless gene and mouse DNA integration site-1 gene family (Wnt)/epithelial-MES transition signaling pathway (46). Thus, interactions between HOXC10 and the tumor microenvironment could be related to correlations between HOXC10 expression and immune infiltration and the poor prognosis of BLBC patients.
In summary, increased HOXC10 expression was found to be correlated with poor prognosis and increased immune-infiltration levels of macrophages in BLBC. HOXC10 expression may contribute to the regulation of monocytes, TAMs, M1 macrophages, and M2 macrophages. Thus, HOXC10 appears to play an important role in immune-cell infiltration and could serve as a prognosis biomarker in patients with BLBC.
Acknowledgements
Funding: This study was supported by the National Natural Science Foundation of China (Grant No. 81702623) and the Science & Technology Development Fund of Tianjin Education Commission for Higher Education, Tianjin, China.
Footnote
Reporting Checklist: The authors have completed the REMARK reporting checklist. Available at https://atm.amegroups.com/article/view/10.21037/atm-21-6611/rc
Conflicts of Interest: All authors have completed the ICMJE uniform disclosure form (available at https://atm.amegroups.com/article/view/10.21037/atm-21-6611/coif). The authors have no conflicts of interest to declare.
Ethical Statement: The authors are accountable for all aspects of the work in ensuring that questions related to the accuracy or integrity of any part of the work are appropriately investigated and resolved. The study was conducted in accordance with the Declaration of Helsinki (as revised in 2013).
Open Access Statement: This is an Open Access article distributed in accordance with the Creative Commons Attribution-NonCommercial-NoDerivs 4.0 International License (CC BY-NC-ND 4.0), which permits the non-commercial replication and distribution of the article with the strict proviso that no changes or edits are made and the original work is properly cited (including links to both the formal publication through the relevant DOI and the license). See: https://creativecommons.org/licenses/by-nc-nd/4.0/.
References
- Siegel RL, Miller KD, Jemal A. Cancer statistics, 2020. CA Cancer J Clin 2020;70:7-30. [Crossref] [PubMed]
- Lu JT, Tan CC, Wu XR, et al. FOXF2 deficiency accelerates the visceral metastasis of basal-like breast cancer by unrestrictedly increasing TGF-β and miR-182-5p. Cell Death Differ 2020;27:2973-87. [Crossref] [PubMed]
- Li H, Zhu Y, Burnside ES, et al. Quantitative MRI radiomics in the prediction of molecular classifications of breast cancer subtypes in the TCGA/TCIA data set. NPJ Breast Cancer 2016; [Crossref] [PubMed]
- Sørlie T, Perou CM, Tibshirani R, et al. Gene expression patterns of breast carcinomas distinguish tumor subclasses with clinical implications. Proc Natl Acad Sci U S A 2001;98:10869-74. [Crossref] [PubMed]
- Perou CM, Sørlie T, Eisen MB, et al. Molecular portraits of human breast tumours. Nature 2000;406:747-52. [Crossref] [PubMed]
- Kreike B, van Kouwenhove M, Horlings H, et al. Gene expression profiling and histopathological characterization of triple-negative/basal-like breast carcinomas. Breast Cancer Res 2007;9:R65. [Crossref] [PubMed]
- Rakha EA, Reis-Filho JS, Ellis IO. Basal-like breast cancer: a critical review. J Clin Oncol 2008;26:2568-81. [Crossref] [PubMed]
- Bartmann C, Wischnewsky M, Stüber T, et al. Pattern of metastatic spread and subcategories of breast cancer. Arch Gynecol Obstet 2017;295:211-23. [Crossref] [PubMed]
- Faiella A, Zappavigna V, Mavilio F, et al. Inhibition of retinoic acid-induced activation of 3' human HOXB genes by antisense oligonucleotides affects sequential activation of genes located upstream in the four HOX clusters. Proc Natl Acad Sci U S A 1994;91:5335-9. [Crossref] [PubMed]
- Zeltser L, Desplan C, Heintz N. Hoxb-13: a new Hox gene in a distant region of the HOXB cluster maintains colinearity. Development 1996;122:2475-84. [Crossref] [PubMed]
- Cantile M, Cindolo L, Napodano G, et al. Hyperexpression of locus C genes in the HOX network is strongly associated in vivo with human bladder transitional cell carcinomas. Oncogene 2003;22:6462-8. [Crossref] [PubMed]
- Shah N, Sukumar S. The Hox genes and their roles in oncogenesis. Nat Rev Cancer 2010;10:361-71. [Crossref] [PubMed]
- Abate-Shen C. Deregulated homeobox gene expression in cancer: cause or consequence? Nat Rev Cancer 2002;2:777-85. [Crossref] [PubMed]
- Tan Z, Chen K, Wu W, et al. Overexpression of HOXC10 promotes angiogenesis in human glioma via interaction with PRMT5 and upregulation of VEGFA expression. Theranostics 2018;8:5143-58. [Crossref] [PubMed]
- Apiou F, Flagiello D, Cillo C, et al. Fine mapping of human HOX gene clusters. Cytogenet Cell Genet 1996;73:114-5. [Crossref] [PubMed]
- Falaschi A, Abdurashidova G, Biamonti G. DNA replication, development and cancer: a homeotic connection? Crit Rev Biochem Mol Biol 2010;45:14-22. [Crossref] [PubMed]
- Marchetti L, Comelli L, D'Innocenzo B, et al. Homeotic proteins participate in the function of human-DNA replication origins. Nucleic Acids Res 2010;38:8105-19. [Crossref] [PubMed]
- Ansari KI, Hussain I, Kasiri S, et al. HOXC10 is overexpressed in breast cancer and transcriptionally regulated by estrogen via involvement of histone methylases MLL3 and MLL4. J Mol Endocrinol 2012;48:61-75. [Crossref] [PubMed]
- Tang XL, Ding BX, Hua Y, et al. HOXC10 Promotes the Metastasis of Human Lung Adenocarcinoma and Indicates Poor Survival Outcome. Front Physiol 2017;8:557. [Crossref] [PubMed]
- Li S, Zhang W, Wu C, et al. HOXC10 promotes proliferation and invasion and induces immunosuppressive gene expression in glioma. FEBS J 2018;285:2278-91. [Crossref] [PubMed]
- Xie X, Xiao Y, Huang X. Homeobox C10 knockdown suppresses cell proliferation and promotes cell apoptosis in osteosarcoma cells through regulating caspase 3. Onco Targets Ther 2018;11:473-82. [Crossref] [PubMed]
- Zhai Y, Kuick R, Nan B, et al. Gene expression analysis of preinvasive and invasive cervical squamous cell carcinomas identifies HOXC10 as a key mediator of invasion. Cancer Res 2007;67:10163-72. [Crossref] [PubMed]
- Feng X, Li T, Liu Z, et al. HOXC10 up-regulation contributes to human thyroid cancer and indicates poor survival outcome. Mol Biosyst 2015;11:2946-54. [Crossref] [PubMed]
- Rhodes DR, Kalyana-Sundaram S, Mahavisno V, et al. Oncomine 3.0: genes, pathways, and networks in a collection of 18,000 cancer gene expression profiles. Neoplasia 2007;9:166-80. [Crossref] [PubMed]
- Mizuno H, Kitada K, Nakai K, et al. PrognoScan: a new database for meta-analysis of the prognostic value of genes. BMC Med Genomics 2009;2:18. [Crossref] [PubMed]
- Tang Z, Li C, Kang B, et al. GEPIA: a web server for cancer and normal gene expression profiling and interactive analyses. Nucleic Acids Res 2017;45:W98-W102. [Crossref] [PubMed]
- Györffy B, Lanczky A, Eklund AC, et al. An online survival analysis tool to rapidly assess the effect of 22,277 genes on breast cancer prognosis using microarray data of 1,809 patients. Breast Cancer Res Treat 2010;123:725-31. [Crossref] [PubMed]
- Li T, Fan J, Wang B, et al. TIMER: A Web Server for Comprehensive Analysis of Tumor-Infiltrating Immune Cells. Cancer Res 2017;77:e108-10. [Crossref] [PubMed]
- Tomczak K, Czerwińska P, Wiznerowicz M. The Cancer Genome Atlas (TCGA): an immeasurable source of knowledge. Contemp Oncol (Pozn) 2015;19:A68-77. [Crossref] [PubMed]
- Pan JH, Zhou H, Cooper L, et al. LAYN Is a Prognostic Biomarker and Correlated With Immune Infiltrates in Gastric and Colon Cancers. Front Immunol 2019;10:6. [Crossref] [PubMed]
- Tu L, Guan R, Yang H, et al. Assessment of the expression of the immune checkpoint molecules PD-1, CTLA4, TIM-3 and LAG-3 across different cancers in relation to treatment response, tumor-infiltrating immune cells and survival. Int J Cancer 2020;147:423-39. [Crossref] [PubMed]
- Denkert C, von Minckwitz G, Darb-Esfahani S, et al. Tumour-infiltrating lymphocytes and prognosis in different subtypes of breast cancer: a pooled analysis of 3771 patients treated with neoadjuvant therapy. Lancet Oncol 2018;19:40-50. [Crossref] [PubMed]
- Tekpli X, Lien T, Røssevold AH, et al. An independent poor-prognosis subtype of breast cancer defined by a distinct tumor immune microenvironment. Nat Commun 2019;10:5499. [Crossref] [PubMed]
- Stanton SE, Disis ML. Clinical significance of tumor-infiltrating lymphocytes in breast cancer. J Immunother Cancer 2016;4:59. [Crossref] [PubMed]
- Carlson MR, Komine Y, Bryant SV, et al. Expression of Hoxb13 and Hoxc10 in developing and regenerating Axolotl limbs and tails. Dev Biol 2001;229:396-406. [Crossref] [PubMed]
- Hostikka SL, Gong J, Carpenter EM. Axial and appendicular skeletal transformations, ligament alterations, and motor neuron loss in Hoxc10 mutants. Int J Biol Sci 2009;5:397-410. [Crossref] [PubMed]
- Yao S, He L, Zhang Y, et al. HOXC10 promotes gastric cancer cell invasion and migration via regulation of the NF-κB pathway. Biochem Biophys Res Commun 2018;501:628-35. [Crossref] [PubMed]
- Suo D, Wang Z, Li L, et al. HOXC10 upregulation confers resistance to chemoradiotherapy in ESCC tumor cells and predicts poor prognosis. Oncogene 2020;39:5441-54. [Crossref] [PubMed]
- Miwa T, Kanda M, Umeda S, et al. Homeobox C10 Influences on the Malignant Phenotype of Gastric Cancer Cell Lines and its Elevated Expression Positively Correlates with Recurrence and Poor Survival. Ann Surg Oncol 2019;26:1535-43. [Crossref] [PubMed]
- Sadik H, Korangath P, Nguyen NK, et al. HOXC10 Expression Supports the Development of Chemotherapy Resistance by Fine Tuning DNA Repair in Breast Cancer Cells. Cancer Res 2016;76:4443-56. [Crossref] [PubMed]
- Pathiraja TN, Nayak SR, Xi Y, et al. Epigenetic reprogramming of HOXC10 in endocrine-resistant breast cancer. Sci Transl Med 2014;6:229ra41. [Crossref] [PubMed]
- Abba MC, Sun H, Hawkins KA, et al. Breast cancer molecular signatures as determined by SAGE: correlation with lymph node status. Mol Cancer Res 2007;5:881-90. [Crossref] [PubMed]
- Li J, Tong G, Huang C, et al. HOXC10 promotes cell migration, invasion, and tumor growth in gastric carcinoma cells through upregulating proinflammatory cytokines. J Cell Physiol 2020;235:3579-91. [Crossref] [PubMed]
- Dang Y, Chen J, Feng W, et al. Interleukin 1β-mediated HOXC10 Overexpression Promotes Hepatocellular Carcinoma Metastasis by Upregulating PDPK1 and VASP. Theranostics 2020;10:3833-48. [Crossref] [PubMed]
- Li B, Han H, Song S, et al. HOXC10 Regulates Osteogenesis of Mesenchymal Stromal Cells Through Interaction with Its Natural Antisense Transcript lncHOXC-AS3. Stem Cells 2019;37:247-56. [Crossref] [PubMed]
- Dai BW, Yang ZM, Deng P, et al. HOXC10 promotes migration and invasion via the WNT-EMT signaling pathway in oral squamous cell carcinoma. J Cancer 2019;10:4540-51. [Crossref] [PubMed]