Towards precision medicine in epilepsy surgery
Introduction
Epilepsy is the second most common neurological disease worldwide, affecting people of all ages. Despite it has been recognized since antiquity, we continue to struggle to understand and treat this kind of paroxysmal brain disorder. In principle, the management of epilepsy requires comprehensive care to address the efficacy, side effects and quality of life issues. The mainstay of epilepsy management is drug therapy with antiepileptic drugs (AEDs). There are almost two-thirds of patients responding to mono-therapy or rational poly-therapy and achieve complete seizure control without major side effects. Whereas, up to a third of all patients with epilepsy are still refractory to medical therapy even in the context of the introduction of new drugs with considerable advantages in safety and tolerability over the last two decades (1,2). Currently, intensity studies are focused on improving the treatment of patients with drug-resistant or pharmaco-resistant epilepsy, which is associated with higher rates of physical and psychiatric comorbidities, cognitive decline and sudden death (3,4).
It has been widely accepted that epilepsy surgery is a highly effective therapeutic option in a selected subset of patients with refractory focal seizure (5,6). For instance, grade 1 evidences from prospective randomized controlled trials (RCT) showed the superiority of epilepsy surgery to pharmacotherapy for patients with medial temporal lobe epilepsy (MTLE) not responding to treatment with two AEDs (7,8). In addition to seizure, cognition, behavior, and quality of life also improved substantially after epilepsy surgery (9,10). Epilepsy surgery roots in the hypothesis of epileptogenic zone (EZ), which is typically defined as the area of cortex that is necessary and sufficient for initiating seizures and whose removal (or disconnection) is necessary for complete abolition of seizures (11,12). The risk and benefit assessment prior to epilepsy surgery needs to be considered carefully. The process of the pre-surgical evaluation requires a multimodality approach wherein each modality provides unique and complimentary information, consisting of clinical history, detailed analysis of semiology, long-term video-EEG recording, inter-ictal and ictal EEG analysis, neuroimaging, and neuropsychological assessment (13). Surgical resection of the EZ is the preferred procedure including antero-medial temporal lobectomy, tailed neocortical resection, lesional resection, and hemispherectomy. Nevertheless, disconnection (corpus callosotomy, multiple subpial transections) and neuro-modification procedures (vagal nerve stimulation, responsive neuro-stimulation, and transcranial stimulation) are most applicable to patients who are currently not candidates for resection epilepsy surgery, because their seizures arise from eloquent cortex, are multifocal/generalized or EZ cannot be identified with available approaches (14).
The influence of epilepsy surgical outcome is multifactorial, depending on epilepsy type, underlying pathology, and accurate localization of EZ by various clinical, neuroimaging, and neurophysiological investigations (15). The purposes of the pre-surgical evaluation are twofold: accurate delineation of the extent of the EZ and precise description about the relationship of EZ with eloquent cortex for complete and safe resection. Stepwise pre-surgical procedure starts from the basic and non-invasive investigations and progressing to more complicated and invasive approaches. However, since no single current available method can identify EZ reliably and each modality has its own limitation, comprehensive investigations had to be needed to assess the different dimensions of the EZ.
Admittedly, accurate localization of EZ still remains challenging, especially in patients with normal features on MRI (16), whereas substantial progress has been made in the methods of pre-surgical assessment in recent years, which widened the applicability of surgical treatment for children and adults with refractory seizure (17). Furthermore, advances also provide opportunity to achieve the minimal side effects and maximal benefit individually, which meets the need for the current concept of precision medicine in epilepsy surgery. In this review, we focus on some state-of-the-art pre-surgical investigations that contribute to the precision medicine.
Advances in neuroimaging have improved our ability to identify lesions causing focal seizure
Identification of structural lesion is one of the best prognostic factors for post-operative seizure freedom. MRI is an essential method for identifying an epileptogenic lesions underlying focal epilepsy (18). The sensitivity of MRI for detecting a structural abnormality is varied, relying on the pathological substrates, applied techniques, and the experience of the interpreting physician. Some types of epileptogenic lesion such as tumors, vascular malformations, infarcts, or post-traumatic defects are easily to be detected. Meanwhile, advances in MRI have improved our ability to identify subtle structural lesions causing focal seizure (18). For example, thin sections perpendicular to the longitudinal axis of the hippocampus are indispensable for detailed evaluation of hippocampal sclerosis, and three-dimensional (3D) MRI with voxels smaller than 1 mm is needed in order to detect subtle structural brain abnormalities. However, there are still epileptic lesions escaping visual detection even with specific, high-resolution imaging techniques, most commonly in patients with focal cortical dysplasia (FCD) (19,20).
FCD are intrinsically epileptogenic lesions frequently causing refractory seizure both in children and adults patients (21). FCD is histologically well defined by the presence of dysmorphic neurons in FCD IIa and additional balloon cells in FCD IIb (22,23). On MRI, FCDII are often characterized by a combination of increased cortical thickness, increased Flair signal within the dysplastic cortex and blurring of the gray and white matter junction. Detection of these lesions, especially during pre-surgical evaluation, is crucial as it significantly improves the chance of becoming seizure-free postoperatively. However, challenge is that FCD might be overlooked by conventional visual inspection because the changes on MRI are so subtle and the lesions may be only a few millimeters in size (18,20). Post-processing methods of quantitative structural MRI analysis including voxel-based morphometry (VBM), cortical thickness mapping, and structural covariance analysis have the potential to identify subtle lesions undetected in previously MRI-negative patients (9,24). Morphometric MRI analysis is a voxel-based method based on algorithms of the statistical parametric mapping (SPM) software (http://www.fil.ion.ucl.ac.uk/spm). The distribution of gray and white matter is analyzed on a voxel-wise basis and compared with a normal database of healthy subjects. VBM highlights brain regions with blurring of the grey—white matter junction and abnormal extension of grey matter into white matter (i.e., abnormal deep sulci) that are the typical MRI features of FCD (25). It has been shown that VBM method allows a more sensitive localization of subtle epileptic lesion when compared with conventional analysis (26).
On the other hand, metabolic image is able to provide different information of brain activities. Functional neuroimaging techniques, such as positron emission tomography (PET), single photon emission computed tomography (SPECT), have proved their usefulness in defining the EZ. Multiple studies have demonstrated metabolic changes mostly in areas tightly coupled with the region generating seizure which are concordant with intracranial EEG findings (27). Areas of functional deficit related to EZ on inter-ictal 18F-fluorodeoxyglucose (FDG)-PET are characterized by reduced inter-ictal metabolism. FDG-PET can reveal abnormalities that can prove otherwise difficulty to be detected by structural image, and lead to successful surgical intervention. Nevertheless, considering that PET is an imaging technique of comparatively low spatial resolution, the application of FDG-PET/MRI co-registration can enhance the detection, improving the spatial resolution (28,29). The combination of structural and functional brain imaging has yielded complementary information for guiding clinical decision (30) (Figure 1).
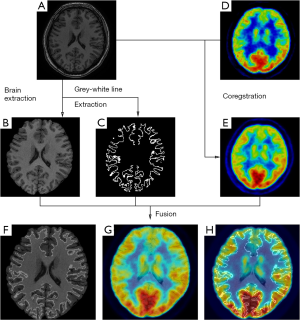
SPECT offer the unique information about cerebral blood flow using tracers (e.g., 99mTc-labeled compounds) that freely cross the blood brain barrier. In the settings of pre-surgical evaluation, ictal SPECT that reflects the specific focal increase of perfusion at the moment of the seizure onset has been established to be superior to inter-ictal SPECT. Furthermore, to improve the sensitivity to the localization of EZ, post-processing of imaging paradigm using computer-aided subtraction of ictal SPECT co-registered to MRI (SISCOM) has been developed by comparing patient ictal scan with inter-ictal to produce subtraction image that is later co-registered to and visualized on patient’s MRI (31,32). Patients with a SISCOM region of blood flow increasing concordant with the EZ are most likely to experience a significant reeducation in seizure if the focal cortical resection includes the region of blood-flow change (33). Thus, SISCOM demonstrated the robust usefulness for highlighting the EZ and better understanding the hemodynamic correlates involved in the generation and the propagation of the seizures (Figure 2).
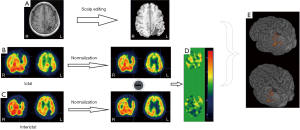
Broadband EEG has proved the critical usefulness in defining the EZ
EEG has long been the key tool for the diagnosis of epilepsy and remains at the heart of the pre-surgical evaluation. Conventionally, neurophysiologists largely focused on brain activities in the Berger frequency bands (0.5–30 Hz). Epileptic cortex is characterized by inter-ictal epileptiform discharges (IEDs) that manifest as transient spike, spike and wave or sharp wave typically in this frequency bands. IEDs are thought as the hallmark of epilepsy, and play a pivotal role in the clinical diagnosis and treatment (34). However, IEDs reflecting the irritative zone do not reliably localize the extent of EZ (35,36). Over the past decades, there is significant development on EEG technique with analog recording methods replaced by digital EEG. To date, EEG recorded from the human brain span a wide range of spatiotemporal scales that extend well beyond traditional clinical EEG, ranging from ultra-slow to HFOs. Broadband EEG holds the promise to deep understanding of the pathophysiology of epilepsy and to develop new clinical diagnostic methods (37,38).
If EEG is recorded with an infinite time constant, slow potentials could be recorded as the direct current (DC) shifts or infraslow activities (IFA). Ictal DC shifts have been observed in the animal epilepsy experiments previously. Recent development of epilepsy surgery and progress in recording techniques facilitate to investigate DC shifts in human brain directly. It has showed that ictal DC shifts most likely occur at the beginning of seizures together with maximal intensity and rapid ictal activities by terms of intracranial recording, reflecting both epileptic neuronal discharges and passively depolarized glial activity as the result of massive depolarization of the neurons with accumulated extracellular potassium (1,39). Compared to a careful interpretation of the conventional ictal EEG recording, ictal DC shifts could provide very important information about core epileptogenicity in patients with refractory focal seizure (40).
On the other hand, wider bandwidth and higher sampling rates are now available on many clinical EEG recording systems, facilitating the studies of HFOs. HFOs are characterized by transient, rhythmic events with evolving of amplitude and frequency ranging from 80 to 600 Hz. They are further classified in ripples (80–250 Hz) and fast ripples (FRs) (250–600 Hz). Ripples and FRs seem to have different pathophysiological mechanisms. HFOs in the 80–200 Hz (ripple) range may reflect inhibitory field potentials, which synchronize neuronal activity, thus facilitating information transfer over long distances. HFOs of 250–600 Hz, referred to as FRs, may reflect abnormal synchronous burst firing of principal neurons in areas of seizure onset. HFOs were studied more thoroughly after they were recorded with microelectrodes in epileptic rats and in patients, and this expanded when they were found with macro-electrodes with clinical significance (41-43). Initially HFOs were described mainly in TLE associated with hippocampal sclerosis in human. However, HFOs are also present in extra-temporal epilepsies associated with different types of lesions, such as tumors, FCD, and nodular heterotopias in epilepsy without an obvious lesion. It has been showed inter-ictal HFOs are increased in the EZ and that resection of HFO-generating tissue is associated with seizure-free outcomes (44). In particular, as above mentioned, non-lesional epilepsies represent a challenge for improvement of epilepsy surgery and HFOs could help map EZ in this type of epilepsy (45). Hence, inter-ictal HFOs could be reliable biomarkers of the seizure onset zone based on evidence from mounting studies, independently of the location and type of lesion (46) (Figure 3). Notably, studies from rodent epilepsy models find that HFOs are a potential biomarker of epileptogenesis for tracking the development of epilepsy.
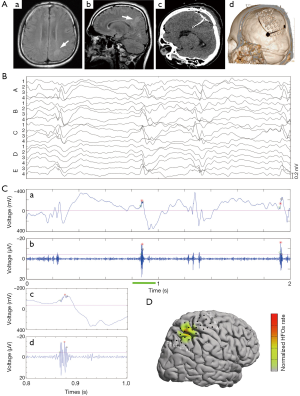
There is ongoing discussion on the differentiation between physiological and epileptic HFOs since normal neuronal circuits can generate physiological HFOs (47). For example, HFOs in the ripple band recorded on medial temporal structure are observed to be involved in memory formation and reactivation of previous experiences (48). It is assumed that combined analysis of IEDs and HFOs might provide new insights into the argument (49). Wang et al. classified neocortical HFOs into type I when superimposed on paroxysmal fast, spike, or sharp wave, and type II when independent of epileptiform discharges (50). It demonstrated that neocortical FRs and type I ripples are specific markers of the SOZ, whereas type II ripples are not. Recently, We studied a distinct electrophysiological pattern of gamma frequency oscillations preceded IEDs (gamma-IEDs) recorded with intracranial EEG brain areas in patients with TLE (51). It revealed that gamma-IEDs were strongly associated with electrodes in the seizure onset zone (SOZ) compared with the surrounding brain regions. The potential clinical application of gamma-IEDs for mapping pathologic brain regions is intriguing (Figure 4). Thus, we term the combination of HFO with IEDs as ‘HFO plus’, which seems to be more reliable and specific to describe the EZ than HFO or IED separately.
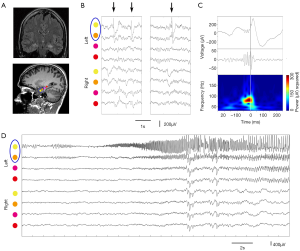
Intracranial EEG deepen our understanding of the spatiotemporal dynamics of seizure
There is no doubt that accurate localization of the EZ is a key to the success of resection surgery for intractable epilepsy. In the pre-surgical evaluation work-up, non-invasive investigations are sufficient for delineating the EZ in the majority of patients suffering from drug-resistant focal seizures, whereas intracranial implantation is needed when the results from non-invasive investigations are obviously discordant or mapping of cortical function is needed because of EZ adjacent or overlapping with eloquent cortex. In essential, it was clear that the placement of intracranial electrodes would be inadequate and surgical results would be probably poor if the localization hypotheses were wrong when considering the intracranial EEG (iEEG) method. Clinical iEEG including subdural grids, strips, depth, and stereoelectroencephalography (SEEG) have been in use for more than 50 years, yet also continues to hold an important role in the precise definition of EZ, offering the unique opportunity to record neural signal direct from brain issues compared with other investigations (52).
Among iEEG, SEEG was firstly developed by Talairach and Bancaud at Hospital Sainte Anne, Paris, and French. The clinical and scientific interest has grown throughout the years due to the advent of high-resolution imaging and robotic stereotactic systems, which contributed to the technical evolution of SEEG (53-55). As a rule, SEEG methodology firstly requires a comprehensive scrutiny of all available information obtained by the non-invasive investigation to formulate a coherent localization hypothesis (56). Different from depth electrodes, commonly used for lateralizing seizure onset, SEEG is aiming to define the EZ using a larger number of stereotaxic trajectories arranged according to the specific anatomo-electro-clinical requirements of each patient (57). Briefly, SEEG is targeted to the more likely structure(s) of ictal onset, the anatomic lesion (if present), and the possible propagation pathway to evaluate the spread of the discharge adequately (Figure 5). Despite the number of electrodes, SEEG is obtained with a very low complication rate (58).
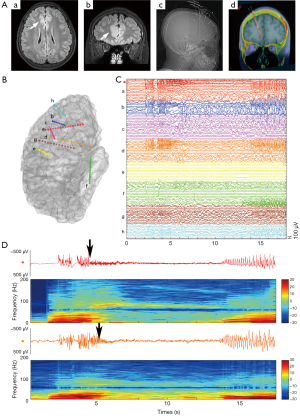
There has been a slight shift in the understanding of EZ in recent years. A substantial body of epilepsy research has shown that EZ influence brain areas crossing ensembles of functionally and anatomically connected brain areas (40). Classical concept of a highly localized structure that forms EZ has recently given way to the notion of a distributed network of modules with intrinsic properties that integrate in the presence of seizure. Since SEEG emphasizes the importance to study the spatiotemporal dynamics of seizure discharges, accounting for the dynamic, multidirectional spatiotemporal organization of the ictal discharges, it has greatly deepen our understanding of ictal clinical semiology of focal seizure by terms of offering stereotactic 3D view of the ictal discharges.
SEEG also offer the chance to perform the minimal invasive neurosurgical techniques in epilepsy surgery (59). There is a long history for the exploration of minimal invasive alternatives in classical open epilepsy surgery. In recent years, the advent of modern imaging techniques and increasing accuracy of stereotactic targeting has paved the way for this treatment modality. Recent promising results from retrospective and small case series have been reported (60,61). In detail, the procedure is based on findings provided by SEEG recording, and stereotactic radiofrequency thermocoagulation (RF-THC) that caused a permanent lesion by heating brain tissue was accomplished efficiently using the same electrodes employed for recording. There are mounting evidences that patients with hypothalamic hamartomas, grey-matter nodular heterotopy and hippocampal sclerosis might be good candidates for minimal invasive treatment with RF-THC (62). It also showed that RF-THC does not implicate additional risks beyond those of a standard SEEG implantation. Hence, SEEG is not only a powerful diagnostic tool for the definition of the EZ but a potential therapeutic technique in carefully selected cases.
Summary
Over past decade, advanced techniques have made a colossal impact on the diagnosis and management of epilepsies. Refinement of advances and future developments of modality would improve our understanding of the dynamics of brain with high spatial and temporal resolutions dramatically. Precision medicine in epilepsy surgery requires integrated broad range of techniques and approaches to ensure the success of subsequent surgery. We are on the way to pursue the best surgical treatment of medical refractory epilepsy individually.
Acknowledgements
Funding: The study was supported by National Natural Science Foundation of China (81271447 and 81571271); China-Japan Friendship Hospital Scientific Research Foundation (2015-1-QN-3).
Footnote
Conflicts of Interest: The authors have no conflicts of interest to declare.
References
- Kwan P, Schachter SC, Brodie MJ. Drug-resistant epilepsy. N Engl J Med 2011;365:919-26. [PubMed]
- Ben-Menachem E. Medical management of refractory epilepsy--practical treatment with novel antiepileptic drugs. Epilepsia 2014;55 Suppl 1:3-8. [PubMed]
- Begley CE, Beghi E, Beran RG, et al. ILAE commission on the burden of epilepsy, subcommission on the economic burden of epilepsy: Final report 1998-2001. Epilepsia 2002;43:668-73. [PubMed]
- Nevalainen O, Ansakorpi H, Simola M, et al. Epilepsy-related clinical characteristics and mortality: a systematic review and meta-analysis. Neurology 2014;83:1968-77. [PubMed]
- Gomez-Alonso J, Bellas-Lamas P. Surgical treatment for drug-resistant epilepsy. JAMA 2015;313:1572. [PubMed]
- Jehi L, Friedman D, Carlson C, et al. The evolution of epilepsy surgery between 1991 and 2011 in nine major epilepsy centers across the United States, Germany, and Australia. Epilepsia 2015;56:1526-33. [PubMed]
- Engel J Jr, McDermott MP, Wiebe S, et al. Early surgical therapy for drug-resistant temporal lobe epilepsy: a randomized trial. JAMA 2012;307:922-30. [PubMed]
- Wiebe S, Blume WT, Girvin JP, et al. A randomized, controlled trial of surgery for temporal-lobe epilepsy. N Engl J Med 2001;345:311-8. [PubMed]
- Fiest KM, Sajobi TT, Wiebe S. Epilepsy surgery and meaningful improvements in quality of life: results from a randomized controlled trial. Epilepsia 2014;55:886-92. [PubMed]
- Hamid H, Blackmon K, Cong X, et al. Mood, anxiety, and incomplete seizure control affect quality of life after epilepsy surgery. Neurology 2014;82:887-94. [PubMed]
- Jobst BC, Cascino GD. Resective epilepsy surgery for drug-resistant focal epilepsy: a review. JAMA 2015;313:285-93. [PubMed]
- Rosenow F, Lüders H. Presurgical evaluation of epilepsy. Brain 2001;124:1683-700. [PubMed]
- West S, Nolan SJ, Cotton J, et al. Surgery for epilepsy. Cochrane Database Syst Rev 2015;7:CD010541. [PubMed]
- Engel J Jr. Surgery for seizures. N Engl J Med 1996;334:647-52. [PubMed]
- Keezer MR, Bell GS, Duncan JS, et al. Promise and pitfalls of prognostic models for epilepsy surgery. Lancet Neurol 2015;14:683-4. [PubMed]
- Schuele SU, Lüders HO. Intractable epilepsy: management and therapeutic alternatives. Lancet Neurol 2008;7:514-24. [PubMed]
- Martinkovic L, Hecimovic H, Sulc V, et al. Modern techniques of epileptic focus localization. Int Rev Neurobiol 2014;114:245-78. [PubMed]
- Bernasconi N, Bernasconi A. Epilepsy: Imaging the epileptic brain--time for new standards. Nat Rev Neurol 2014;10:133-4. [PubMed]
- Bast T. Outcome after epilepsy surgery in children with MRI-negative non-idiopathic focal epilepsies. Epileptic Disord 2013;15:105-13. [PubMed]
- So EL, Lee RW. Epilepsy surgery in MRI-negative epilepsies. Curr Opin Neurol 2014;27:206-12. [PubMed]
- Berg AT. Paediatric epilepsy surgery: making the best of a tough situation. Brain 2015;138:4-5. [PubMed]
- Blümcke I, Thom M, Aronica E, et al. The clinicopathologic spectrum of focal cortical dysplasias: a consensus classification proposed by an ad hoc Task Force of the ILAE Diagnostic Methods Commission. Epilepsia 2011;52:158-74. [PubMed]
- Palmini A, Najm I, Avanzini G, et al. Terminology and classification of the cortical dysplasias. Neurology 2004;62:S2-8. [PubMed]
- Martin P, Bender B, Focke NK. Post-processing of structural MRI for individualized diagnostics. Quant Imaging Med Surg 2015;5:188-203. [PubMed]
- Wagner J, Weber B, Urbach H, et al. Morphometric MRI analysis improves detection of focal cortical dysplasia type II. Brain 2011;134:2844-54. [PubMed]
- Wang ZI, Jones SE, Jaisani Z, et al. Voxel-based morphometric magnetic resonance imaging (MRI) postprocessing in MRI-negative epilepsies. Ann Neurol 2015;77:1060-75. [PubMed]
- Chassoux F, Rodrigo S, Semah F, et al. FDG-PET improves surgical outcome in negative MRI Taylor-type focal cortical dysplasias. Neurology 2010;75:2168-75. [PubMed]
- Rubí S, Setoain X, Donaire A, et al. Validation of FDG-PET/MRI coregistration in nonlesional refractory childhood epilepsy. Epilepsia 2011;52:2216-24. [PubMed]
- Salamon N, Kung J, Shaw SJ, et al. FDG-PET/MRI coregistration improves detection of cortical dysplasia in patients with epilepsy. Neurology 2008;71:1594-601. [PubMed]
- Lee KK, Salamon N. [18F] fluorodeoxyglucose-positron-emission tomography and MR imaging coregistration for presurgical evaluation of medically refractory epilepsy. AJNR Am J Neuroradiol 2009;30:1811-6. [PubMed]
- Bianchin MM, Wichert-Ana L, Velasco TR, et al. Imaging epilepsy with SISCOM. Nat Rev Neurol 2011;7:1-2. [PubMed]
- Valenti MP, Froelich S, Armspach JP, et al. Contribution of SISCOM imaging in the presurgical evaluation of temporal lobe epilepsy related to dysembryoplastic neuroepithelial tumors. Epilepsia 2002;43:270-6. [PubMed]
- von Oertzen TJ, Mormann F, Urbach H, et al. Prospective use of subtraction ictal SPECT coregistered to MRI (SISCOM) in presurgical evaluation of epilepsy. Epilepsia 2011;52:2239-48. [PubMed]
- Gorji A, Speckmann EJ. Epileptiform EEG spikes and their functional significance. Clin EEG Neurosci 2009;40:230-3. [PubMed]
- Dworetzky BA, Reinsberger C. The role of the interictal EEG in selecting candidates for resective epilepsy surgery. Epilepsy Behav 2011;20:167-71. [PubMed]
- Jobst BC, Engel J Jr. Is it time to replace epileptic spikes with fast ripples? Neurology 2015;85:114-5. [PubMed]
- Vanhatalo S, Voipio J, Kaila K. Full-band EEG (fbEEG): a new standard for clinical electroencephalography. Clin EEG Neurosci 2005;36:311-7. [PubMed]
- Ren L, Terada K, Baba K, et al. Ictal very low frequency oscillation in human epilepsy patients. Ann Neurol 2011;69:201-6. [PubMed]
- Ikeda A, Yazawa S, Kunieda T, et al. Scalp-recorded, ictal focal DC shift in a patient with tonic seizure. Epilepsia 1997;38:1350-4. [PubMed]
- Kramer MA, Cash SS. Epilepsy as a disorder of cortical network organization. Neuroscientist 2012;18:360-72. [PubMed]
- Bragin A, Engel J Jr, Wilson CL, et al. High-frequency oscillations in human brain. Hippocampus 1999;9:137-42. [PubMed]
- Buzsáki G, Horváth Z, Urioste R, et al. High-frequency network oscillation in the hippocampus. Science 1992;256:1025-7. [PubMed]
- Worrell GA, Gardner AB, Stead SM, et al. High-frequency oscillations in human temporal lobe: simultaneous microwire and clinical macroelectrode recordings. Brain 2008;131:928-37. [PubMed]
- Höller Y, Kutil R, Klaffenböck L, et al. High-frequency oscillations in epilepsy and surgical outcome. A meta-analysis. Front Hum Neurosci 2015;9:574. [PubMed]
- Gloss D, Nolan SJ, Staba R. The role of high-frequency oscillations in epilepsy surgery planning. Cochrane Database Syst Rev 2014;1:CD010235. [PubMed]
- Zijlmans M, Jiruska P, Zelmann R, et al. High-frequency oscillations as a new biomarker in epilepsy. Ann Neurol 2012;71:169-78. [PubMed]
- Engel J Jr, Bragin A, Staba R, et al. High-frequency oscillations: what is normal and what is not? Epilepsia 2009;50:598-604. [PubMed]
- Axmacher N, Elger CE, Fell J. Ripples in the medial temporal lobe are relevant for human memory consolidation. Brain 2008;131:1806-17. [PubMed]
- Jacobs J, Vogt C, LeVan P, et al. The identification of distinct high-frequency oscillations during spikes delineates the seizure onset zone better than high-frequency spectral power changes. Clin Neurophysiol 2016;127:129-42. [PubMed]
- Wang S, Wang IZ, Bulacio JC, et al. Ripple classification helps to localize the seizure-onset zone in neocortical epilepsy. Epilepsia 2013;54:370-6. [PubMed]
- Ren L, Kucewicz MT, Cimbalnik J, et al. Gamma oscillations precede interictal epileptiform spikes in the seizure onset zone. Neurology 2015;84:602-8. [PubMed]
- Andrzejak RG, David O, Gnatkovsky V, et al. Localization of epileptogenic zone on pre-surgical intracranial EEG recordings: toward a validation of quantitative signal analysis approaches. Brain Topogr 2015;28:832-7. [PubMed]
- Cardinale F. Talairach methodology in the era of 3D multimodal imaging: "The song remains the same," but catchier, and therefore more helpful for clinical decision making and surgical planning in epilepsy surgery. Epilepsia 2015;56:976-7. [PubMed]
- González-Martínez J, Bulacio J, Thompson S, et al. Technique, results, and complications related to robot-assisted stereoelectroencephalography. Neurosurgery 2016;78:169-80. [PubMed]
- Zuluaga MA, Rodionov R, Nowell M, et al. SEEG trajectory planning: combining stability, structure and scale in vessel extraction. Med Image Comput Comput Assist Interv 2014;17:651-8. [PubMed]
- González-Martínez J. Convergence of stereotactic surgery and epilepsy: the stereoelectroencephalography method. Neurosurgery 2015;62 Suppl 1:117-22. [PubMed]
- Gnatkovsky V, de Curtis M, Pastori C, et al. Biomarkers of epileptogenic zone defined by quantified stereo-EEG analysis. Epilepsia 2014;55:296-305. [PubMed]
- Cardinale F, Cossu M. SEEG has the lowest rate of complications. J Neurosurg 2015;122:475-7. [PubMed]
- Quigg M, Harden C. Minimally invasive techniques for epilepsy surgery: stereotactic radiosurgery and other technologies. J Neurosurg 2014;121 Suppl:232-40. [PubMed]
- Catenoix H, Mauguière F, Montavont A, et al. Seizures outcome after stereoelectroencephalography-guided thermocoagulations in malformations of cortical development poorly accessible to surgical resection. Neurosurgery 2015;77:9-14; discussion 14-5. [PubMed]
- Cossu M, Fuschillo D, Casaceli G, et al. Stereoelectroencephalography-guided radiofrequency thermocoagulation in the epileptogenic zone: a retrospective study on 89 cases. J Neurosurg 2015;123:1358-67. [PubMed]
- Chang EF, Englot DJ, Vadera S. Minimally invasive surgical approaches for temporal lobe epilepsy. Epilepsy Behav 2015;47:24-33. [PubMed]