TRPC6 ameliorates renal ischemic reperfusion injury by inducing Zn2+ influx and activating autophagy to resist necrosis
Introduction
Ischemia reperfusion injury (IRI) may occur following acute hemorrhage, severe trauma, toxic shock, or kidney transplantation (1,2). Due to the characteristics of high perfusion and high metabolism, the kidney is extremely sensitive to ischemia and hypoxia stimulation and prone to IRI (3), and research has shown renal IRI (RIRI) is one of the major factors affecting renal function recovery and long-term survival after renal ischemia or transplantation (4). Therefore, further exploration of the pathogenesis of RIRI and effective treatment strategies is of clinical significance.
As a member of the transient receptor potential family of calcium channels, transient receptor potential channel 6 (TRPC6) is a nonselective cation channel and a key molecule of slit diaphragm (SD) (5,6). It was reported that TRPC6 could be involved in the intracellular flow of Ca2+, Fe2+, and Zn2+ (7,8), and Zn2+ had an antioxidant role and could prevent renal cell apoptosis in IRI (9). Thus, we speculated that TRPC6 could restrain programmed cell necrosis by mediating Zn2+ influx and play a protective role in RIRI. However, the regulatory role of Zn2+ on programmed cell necrosis in RIRI is not entirely clear. Role of Zn2+ in the activation of phosphatidylinositol 3-kinase (PI3K)/Akt signaling pathway to protect against renal I/R injury has been investigated in published research (10). It has been observed that I/R injury results in significant increase in the serum levels of blood urea nitrogen (BUN) and creatinine and increase in the fractional excretion of sodium (FENa) which is decreased by zinc chloride (ZnCl2) supplementation (11,12). Besides, Zn2+ also improved glomerular filtration rate value, Cr-clearance, and urine flow rate beyond renal I/R induced renal injury (12,13). Furthermore, Major antioxidant enzymes including superoxide dismutase (SOD), catalase (CAT), glutathione peroxidase (GPX), and glutathione (GSH) decreased after renal I/R-induced injury. It has been suggested that Zn2+ supplementation stimulates the activity of these enzyme (14). However, it is still unclear whether Zn2+ is the key regulator of TRPC6 in renal ischemic reperfusion injury.
Autophagy is a highly conserved metabolic mechanism in the organic evolutionary process (15), and plays a fatal regulatory role in programmed cell necrosis (16). In addition, it has been recently disclosed that Zn2+ can induce autophagy (17) and can protect RIRI by accelerating autophagy (18). However, it is still unclear whether Zn2+ can participate in the suppression of TRPC6 on cell necrosis by regulating cell autophagy.
TRPC6 is a receptor-activated nonselective cation channel that is homogeneously expressed throughout the central nervous system and peripheral tissues, including the kidneys (19). It has been suggested to play a significant role in I/R injury of the lungs, retinas, and brain (20,21). Our previous study identified TRPC6 as an up-regulated differentially expressed gene in the pathogenesis of I/R injury, and protected against I/R injury, suggesting that it may be a potential novel target for therapy (22). However, the underlying mechanism of TRPC6 in renal I/R injury has not been investigated. In current study, we further verified the influences of TRPC6 on necrosis,Zn2+ influx, and autophagy in oxygen-glucose deprivation and re-oxygenation (OGD/R) HK-2 cells and RIRI model rats.
We present the following article in accordance with the ARRIVE reporting checklist (available at https://atm.amegroups.com/article/view/10.21037/atm-21-5837/rc).
Methods
Establishment of OGD/R HK-2 cells
HK-2 cells were purchased from the Shanghai Institute for Biological Sciences, Chinese Academy of Sciences. For the OGD/R model, HK-2 cells were grown in an airtight container with ischemia simulation medium (0.338 mmol/L Na2HPO4, 136.89 mmol/L NaCl, 5.37 mmol/L KCl, 0.44 mmol/L KH2PO4, 4.166 mmol/L NaHCO3, and 5 mmol/L D-glucose; pH =6.8), 5% CO2, and 95% N2. The airtight container was then plated into an anoxic incubator (5% CO2, 1% O2, and 94% N2) at 37 ℃ for 2 h, and the ischemia simulation medium was then replaced with conventional medium. Cells were then incubated under normal culture conditions (37 ℃ and 5% CO2) for 3 h (22).
Cell treatment
Negative control (NC), TRPC6 shRNAs (sh-TRPC6), and TRPC6-overexpressed plasmid (OE-TRPC6) were gained from HanBio Biotechnology (Shanghai, China). The sequence for TRPC6 shRNA was 5'-AAGAAGUUCUGUAAUUUCCAG-3' (sense) and 5'-GGAAAUUACAGAACUUCUUCU-3' (anti-sense). OGD/R HK-2 cells were then transfected with these plasmids with Lipofectamine 3000 (Invitrogen) for 48 h, and the cells were also treated with 0.3 mM ZnSO4, TPEN, rapamycin, or 3-MA, respectively.
Establishment of RIRI model rats
A total of 36 male SD rats (age: 6–8 weeks; bodyweight: 201±38.3 g) were provided by the Experimental Animal Center of Army Medical University (Chongqing, China), and kept in a standard comfortable environment. Animal experiments were approved by the Animal Care and Use Committee of the Army Medical University [with two institutional licenses SCXK(YU)20170002 and SYXK(YU)20170002], in compliance with the guidelines of the Army Medical University for the care and use of animals. A protocol was prepared before the study without registration. These rats were divided into a sham group (n=6) and I/R group (n=30). After the abdominal cavity of rats in the IR group was opened, the bilateral renal pedicles were closed with vascular clips to block the renal blood supply for 30 min, after which the vascular clips were then removed to restore renal blood perfusion and the wound sutured. In the sham group, after opening the abdominal cavity, the kidney pedicle was not clamped, and the kidneys were exposed for 30 min before suturing. RIRI model rats were then treated with the TRPC6 agonist (OGA), TRPC6 inhibitor (SKF96365), SKF96365 and 15 mg/kg ZnSO4, SKF96365, and rapamycin.
Renal damage rating scale
The Histological Score of the Kidney (HSK) was microscopically evaluated by the same pathologist. The section of the damaged kidney was evaluated based on tubule cell swelling, nuclear pyknosis (apoptosis), and nucleolysis (necrosis). Renal injury was divided into 4 grades: 0= normal; 1 point = minor damage (less than 1/3 nuclear pyknosis or nucleolysis); 2 points = moderate damage (1/3 to 2/3); and 3 points = severe damage: greater than 2/3.
MTT assay
OGD/R HK-2 cells (1×105 cells/mL) in each group were evenly inoculated into 96-well plates and incubated for 48 h, then 20 µL MTT (5 mg/mL; Sigma-Aldrich, Cat. no. 298-93-1) was added to each cell before incubation for 3 h. After the supernatant was discarded, 150 µL DMSO was added to the cells and shaken for 10 min. A microplate analyzer was used to confirm the absorbance at 490 nm.
Flow cytometry
The cell necrosis rate was tested using an Annexin V- FITC/PE kit (Sigma). By referencing the instructions, the treated OGD/R HK-2 cells were collected and suspended with 100 µL 1× binding buffer, then disposed of with 5 µL FITC-Annexin V and 5 µL PE solution for 15 min in the dark. Finally, the number of necrotic cells was monitored using FACS Calibur flow cytometry (BD Biosciences).
Western blot
We first obtained total proteins from the treated OGD/R HK-2 cells or kidney tissues of RIRI model rats, which had also been ground, using RIPA buffer (Beyotime, Shanghai, China). After quantification, the protein (40 µg) was detached via electrophoresis on 10% SDS-PAGE, then transferred to PVDF membrane (Millipore). After sealing, the treated membranes were then soaked with primary antibodies at 4 ℃ overnight and secondary antibody for 2 h. Finally, the protein blots were visualized with the ECL system (Thermo Fisher Scientific). All antibodies were obtained from Abcam (USA).
Detection of Zn2+ and autophagy
Based on the specifications provided by the supplier, free Zn2+ was examined with a Zn2+ detection kit (sigma-Aldrich, Cat. No. MAK032) and cell autophagy was tested by the MDC method with an autophagy staining kit (Solarbio, cat. no. G1170).
H&E staining
Kidney tissues from rats were collected after sacrifice and subjected to a series of treatments including fixation (4% paraformaldehyde), dehydration (gradient alcohol), and paraffin embedding, before being cut into 4-µm slices. After dewaxing, the slices were further addressed with xylene I, xylene II, gradient ethanol, and distilled water, then dyed with Harris hematoxylin, 1% hydrochloric acid alcohol, 0.6% ammonia, and eosin. Through dehydration and transparency, the degree of kidney tissue injury was observed under a light microscope.
Immunohistochemistry (IHC) assay
Slices were processed with 3% hydrogen peroxide and EDTA after dewaxing, then disposed of primary antibodies (anti-RIP1 and anti-PARP-1) at 37 ℃ for 1 h and secondary antibody for 30 min. DAB, hematoxylin, and 0.1% hydrochloric acid were then added to slices, and after dehydration, the results were confirmed with a light microscope.
Statistical analysis
All data repeated three times are represented as mean ± SD. Data were counted with one-way analysis of variance and P<0.05 denoted that difference was statistically significant.
Results
TRPC6 markedly enhanced viability and resisted necrosis in OGD/R HK-2 cells
TRPC6 has been reported to participate in I/R injury of target organs, and we further explored its impact on RIRI. Firstly, we constructed an OGD/R cell model with HK-2 cells as previously reported (23), and, as displayed in Figure 1A, cell viability was observably reduced in the OGD/R group compared to the sham group. In addition, silence of TRPC6 caused a significant decrease in the viability of OGD/R HK-2 cells, while TRPC6 overexpression exerted an opposite effect to TRPC6 silencing on cell viability. Flow cytometry data revealed cell necrosis was markedly aggrandized in the OGD/R group compared to the sham group and could be signally motivated by TRPC6 silencing and decreased by TRPC6 overexpression in OGD/R HK-2 cells (Figure 1B). Necrosis-related proteins (Sirtuin-2, RIP1, and PARP-1) were also dramatically upregulated in the OGD/R group compared to the sham group, and the expression of these proteins could be notably up-regulated by TRPC6 silencing and prominently down-regulated by TRPC6 overexpression in OGD/R HK-2 cells (Figure 1C). On balance, our data disclosed that upregulation of TRPC6 could result in a remarkable increase in viability and a noteworthy decrease in necrosis of OGD/R HK-2 cells.
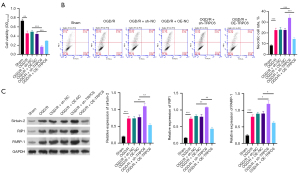
TRPC6 induced Zn2+ influx and autophagy in OGD/R-mediated HK-2 cells
TRPC6 has been reported to participate in Zn2+ influx, and Zn2+ can induce autophagy. In this study we further confirmed whether TRPC6 could affect Zn2+ influx and autophagy in OGD/R HK-2 cells. Firstly, the data signified that free Zn2+ was signally increased in the OGD/R group compared to the sham group, and silence of TRPC6 could result in a conspicuous decrease in free Zn2+, while TRPC6 overexpression could result in a distinct increase in free Zn2+ of OGD/R HK-2 cells (Figure 2A). Secondly, the results from MDC denoted that cell autophagy was memorably elevated in the OGD/R group compared to the sham group, and cell autophagy could be observably repressed by TRPC6 silencing and markedly accelerated by TRPC6 overexpression in OGD/R HK-2 cells (Figure 2B). Thirdly, Western blotting data showed that relative to the sham group, LC3II/I, beclin-1, ATG16L1, and ATG5 were dramatically upregulated, and p62 was prominently downregulated in the OGD/R group, and the changes in these proteins mediated by OGD/R could be notably attenuated by TRPC6 silencing and enhanced by TRPC6 overexpression in HK-2 cells (Figure 2C). Consequently, we proved that an increase of TRPC6 could also exhibit inductive effects in Zn2+ influx and autophagy of OGD/R-mediated HK-2 cells.
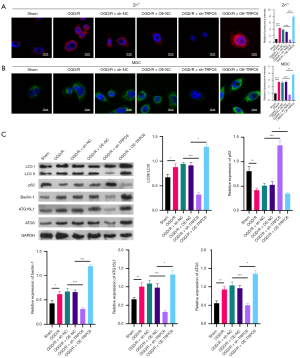
Increase of Zn2+ induced viability and reduced necrosis in HK-2 cells with OGD/R
Subsequently, we also identified the influence of Zn2+ on the viability and necrosis of OGD/R HK-2 cells. We first screened the optimal concentration of Zn2+ on OGD/R HK-2 cells through MTT, and the data manifested that cell viability was signally enhanced in 0.1, 0.3, and 0.5 mM ZnSO4 treatment groups versus that in the control group and was highest in the 0.3 mM ZnSO4 group (Figure 3A). Thus, 0.3 mM ZnSO4 was adopted to construct the Zn2+ treatment group in OGD/R HK-2 cells. We also applied Zn2+ chelating agent (TPEN) to treat OGD/R HK-2 cells, and as displayed in Figure 3B, cell viability in OGD/R HK-2 cells was markedly raised in the Zn2+ group and observably decreased in the TPEN group. Data also indicated that Zn2+ treatment memorably reduced the necrosis rate of OGD/R HK-2 cells while TPEN treatment notably heightened their necrosis rate (Figure 3C). Similarly, we proved that the levels of Sirtuin-2, RIP1, and PARP-1 could be prominently downregulated by Zn2+ treatment and dramatically upregulated by TPEN treatment in OGD/R HK-2 cells (Figure 3D). Therefore, these data showed that like TRPC6 overexpression, the introduction of Zn2+ could accelerate the viability of OGD/R HK-2 cells and prevent necrosis.
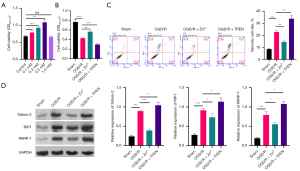
Increase of Zn2+ heightened autophagy in OGD/R HK-2 cells
Just as TRPC6 has a prominent induction role on autophagy of OGD/R HK-2 cells, we also explored the influence of Zn2+ on cell autophagy. The MDC data first exhibited that cell autophagy could be notably strengthened by Zn2+ addition and observably weakened by TPEN addition in OGD/R HK-2 cells (Figure 4A). Data also denoted that introduction of Zn2+ could cause a striking increase in LC3II/I, beclin-1, ATG16L1, and ATG5 levels, and an outstanding reduction in p62 level in OGD/R HK-2 cells, and the increase of TPEN could also cause the opposite effect of Zn2+ on the expression of these proteins (Figure 4B,4C). Overall, these data verified that the increase of Zn2+ alone also had a remarkable accelerative role on autophagy in OGD/R HK-2 cells.
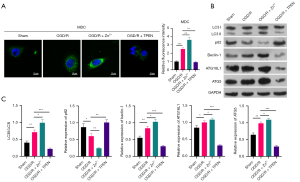
Autophagy activation accelerated viability and restrained necrosis in OGD/R HK-2 cells
As both TRPC6 and Zn2+ were seen to induce autophagy, we further investigated whether autophagy change alone could affect the viability and necrosis of OGD/R HK-2 cells. The autophagy activator (rapamycin) and autophagy inhibitor (3-MA) were utilized to treat OGD/R HK-2 cells, respectively, and MTT data showed that compared to the OGD/R + DMSO group, cell viability was memorably increased in the OGD/R + rapamycin group and signally degraded in the OGD/R + 3-MA group (Figure 5A). In addition, flow cytometry results showed that relative to the OGD/R + DMSO group, the necrosis rate was prominently lowered in the OGD/R + rapamycin group and markedly elevated in the OGD/R + 3-MA group (Figure 5B). The results also showed that Sirtuin-2, RIP1, and PARP-1 expressions were lower in the OGD/R + rapamycin group and higher in the OGD/R + 3-MA group than in the OGD/R + DMSO group (Figure 5C). MDC results also signified that cell autophagy could be notably induced by rapamycin and dramatically restrained by 3-MA in OGD/R HK-2 cells (Figure 5D). These results proved that activation of autophagy alone, like TRPC6 and Zn2+, could also alleviate RIRI.
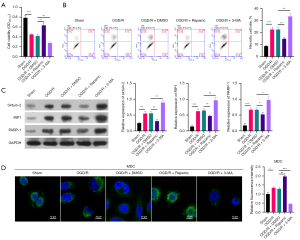
TRPC6 prevented necrosis of OGD/R HK-2 cells by promoting Zn2+ influx and activating autophagy
Based on the effect of TRPC6 overexpression, Zn2+ addition and autophagy activation on preventing necrosis and inducing autophagy in OGD/R HK-2 cells, the rescued experiment was then adopted to further explore the possible relationship between the three in cell viability and necrosis. Firstly, TRPC6 overexpression-mediated enhancement of cell viability could be markedly reversed by TPEN or 3-MA, and TRPC6 silencing-mediated suppression of cell viability could be memorably weakened by Zn2+ or rapamycin in OGD/R HK-2 cells (Figure 6A). Secondly, either TPEN or 3-MA could prominently attenuate the inhibition of cell necrosis mediated by TRPC6 overexpression, and either Zn2+ or rapamycin could dramatically reverse the induction of cell necrosis mediated by TRPC6 silencing in OGD/R HK-2 cells (Figure 6B). Thirdly, TRPC6 overexpression-mediated downregulation of Sirtuin-2, RIP1, and PARP-1 could also be signally blunted by TPEN or 3-MA, and TRPC6 silencing-mediated upregulation of Sirtuin-2, RIP1, and PARP-1 also could be significantly weakened by Zn2+ or rapamycin in OGD/R HK-2 cells (Figure 6C). Generally, these findings certified that TRPC6 could facilitate viability and suppress necrosis of OGD/R HK-2 cells by inducing Zn2+ influx and autophagy.
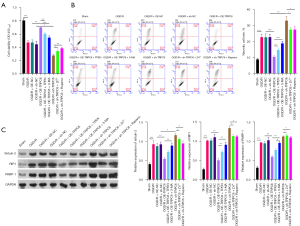
TRPC6 promoted Zn2+ influx and autophagy in OGD/R HK-2 cells by accelerating Zn2+ influx and autophagy
We also analyzed the regulatory relationships among TRPC6 overexpression, Zn2+ addition, and autophagy activation through rescued experiment. As displayed in Figure 7A, either TPEN or 3-MA could signally prevent the acceleration of Zn2+ influx mediated by TRPC6 overexpression, and either Zn2+ or rapamycin could notably reduce the suppression of Zn2+ influx mediated by TRPC6 silencing in OGD/R HK-2 cells (Figure 7A). Subsequently, Western blot data indicated that upregulation of LC3II/I, beclin-1, ATG16L1, and ATG5, and downregulation of p62 which were mediated by TRPC6 overexpression could be dramatically decreased by TPEN or 3-MA, and downregulation of LC3II/I, beclin-1, ATG16L1, ATG5, and upregulation of p62, which were mediated by TRPC6 silencing could be memorably reversed by Zn2+ or rapamycin in OGD/R HK-2 cells (Figure 7B). Consequently, the data disclosed that TRPC6 could also enhance Zn2+ influx and autophagy of OGD/R HK-2 cells by encouraging Zn2+ influx and autophagy.
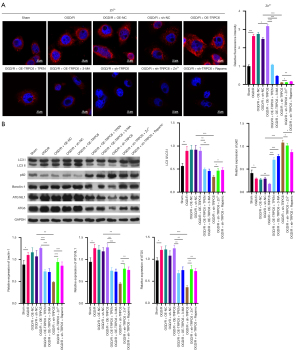
TRPC6 ameliorated kidney injury in RIRI model rats by regulating Zn2+ influx and autophagy
We further studied the impacts of TRPC6, Zn2+, and autophagy on kidney injury in vivo using an RIRI rat model (I/R group). After processing with the TRPC6 agonist (OGA), TRPC6 inhibitor (SKF96365), SKF96365, and ZnSO4, SKF96365, and rapamycin, the data exhibited that the damage rating scale of rats was observably elevated in the I/R group versus that in the sham group; relative to the I/R group, the damage rating scale was markedly lowered in the I/R + OGA group and prominently increased in the I/R + SKF96365 group; and compared to the I/R + SKF96365 group, either ZnSO4 or rapamycin could signally reduce the damage rating scale in I/R rats after administration with SKF96365 (Figure 8A). H&E staining results also signified that in the sham group, kidney cells were arranged neatly and the structure was complete, while in the I/R group, vast inflammatory cells were infiltrated in the interstitium of the kidney, the lumen of the renal tubules was dilated, the renal cells were exfoliated or necrotic, and the nuclei were displaced in different degrees. Further, in the I/R + OGA group, the degree of renal injury was observably reduced in I/R rats, and in the I/R + SKF96365 group, the degree of renal injury was markedly increased in I/R rats. After treatment with ZnSO4 or rapamycin, the degree of renal injury mediated by SKF96365 could also be memorably attenuated in I/R rats (Figure 8B). Thus, we concluded that TRPC6 could alleviate kidney injury of RIRI rats by inducing Zn2+ influx and autophagy.
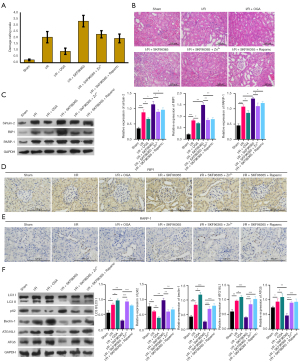
TRPC6 weakened necrosis and enhanced autophagy in RIRI model rats by promoting Zn2+ influx and autophagy
More importantly, we further verified the influences of TRPC6, Zn2+, and autophagy on necrosis and autophagy in the renal tissues of RIRI rats. As manifested in Figure 8B-8E, Sirtuin-2, RIP1, and PARP-1 expressions were memorably raised in I/R group relative to that in sham the group; compared with the I/R group, Sirtuin-2, RIP1, and PARP-1 expressions were also dramatically decreased in the I/R+OGA group and observably increased in the I/R + SKF96365 group; and compared to the I/R + SKF96365 group, either ZnSO4 or rapamycin could also prominently downregulate Sirtuin-2, RIP1, and PARP-1 expressions in I/R rats after administration with SKF96365. The data also displayed that LC3II/I, beclin-1, ATG16L1, and ATG5 expressions were higher and p62 was lower in the I/R group than in the sham group; OGA treatment could increase LC3II/I, beclin-1, ATG16L1, and ATG5 expressions and decrease p62 expression in I/R rats; and SKF96365 had an opposite effect to OGA on these autophagy-related proteins. At the same time, SKF96365-medaited downregulation of LC3II/I, beclin-1, ATG16L1, and ATG5, and upregulation of p62 could be notably reversed by ZnSO4 or rapamycin treatment in I/R rats (Figure 8F). In summary, these results revealed that TRPC6 also could improve RIRI in vivo by accelerating Zn2+ influx and autophagy.
Discussion
RIRI is one of the frequent risk factors causing acute kidney injury (AKI) (24). Clinically, RIRI is often associated with procedures such as cardiopulmonary surgery, kidney transplantation, and artery bypass surgery (25). As RIRI has a serious impact on the prognosis of patients, and there is no effective therapy strategy (26), it is of great clinical value to actively explore its pathological mechanism and effective targeted therapy strategies. Studies show that RIRI can cause multiple pathophysiological changes including leukocyte activation and endothelial cell dysfunction, causing inflammation and tissue injury (25,27), and renal tubular epithelial cells (such as HK-2 cells) are the main sites of IRI due to their active metabolism (28). In this study, we adopted an internationally recognized RIRI in vitro model (OGD/R) and I/R in vivo model to further investigate the possible pathological processes in RIRI. The results signified that OGD/R could result in a prominent inhibition in viability, Zn2+ influx, and autophagy and a noteworthy promotion in necrosis of OGD/R HK-2 cells, indicating the successful build of OGD/R HK-2 cells. In addition, we also discovered that the degree of kidney injury was also aggravated in RIRI model rats, further suggesting a successful RIRI model in rats had been established.
TRPC is a non-selective cationic infiltration channel widely expressed in the heart, kidney, brain, and other vital organs (29). In the kidney, TRPC6 is mainly expressed in mature podocytes, glomerular mesangial cells, and renal vascular smooth muscle cells, especially near SD (30). Studies have certified that overactivation of TRPC6 can increase the concentration of Ca2+ in podocytes, leading to their injury and dysfunction, and even glomerular disease (31,32). TRPC6 is also associated with glomerulosclerosis and diabetic nephropathy (33) and has been certified to be relevant to the podocyte response to ischemic injury (6). In the current study, we further confirmed that TRPC6 overexpression could be weakened and TRPC6 silencing could enhance the inhibition of viability, Zn2+ influx, and autophagy, and the induction of necrosis in OGD/R HK-2 cells or RIRI model rats. Thus, we proved that TRPC6 might serve an ameliorating action on RIRI.
A recent study also indicated that TRPC6 overexpression contributed to the accumulation of intracellular Zn2+ (34). Activation of TRPC6 could induce the mitochondria to release Zn2+ (35). Zinc element is one of the essential trace elements with abundant content, and it was reported that Zn2+ can participate in antioxidant reactions, nerve conduction, and regulation of the activities of various enzymes and growth factors (36). Studies have also demonstrated that Zn2+ is a key ion mediating cerebral ischemia injury (37). Reduction of zinc transporters could cause myocardial IRI (37); zinc preconditioning had a protective effect on RIRI in a preclinical sheep model (38); and Zn2+ could protect myocardial IRR by regulating endoplasmic reticulum stress (ERS) (39). In our study, we further testified that an increase of Zn2+ could enhance viability and autophagy and restrain necrosis in OGD/R HK-2 cells or RIRI model rats, which were mediated by TRPC6.
Autophagy is both a mechanism of cell death and a self-protection mechanism of cells (40), and has been proved to play a key role in tumor, aging, and neurodegeneration (41). In the process of autophagosome formation, multiple ATG proteins are needed, and studies have confirmed that up-regulation of Beclin1 expression can induce autophagy (42). Atg12 and LC3 also play an important role in the formation of autophagosomes, with the former activated by Atg7, initiating the binding process and allowing Atg12 to interact with other factors leading to the formation of the Atg12-Atg5-Atg16L1 complex. At the same time, ATG7 can also promote the modification of LC3 I to LC3 II (43). Studies have shown that the mechanism of necrosis is related to PAPR (44), and that activating PARP requires a large amount of ATP, and that the inhibition of autophagy to necrosis is mainly manifested in the maintenance of energy (45). Autophagy has also been reported to play a dual role in RIRI (46). While activation of autophagy during RIRI has been observed in many experiments in vivo and in vitro (47) it is still controversial whether up-regulated autophagy has a protective effect on the kidney or aggravates kidney injury. On the one hand, autophagy can degrade abnormal proteins and organelles in abnormal cells, preventing the accumulation of harmful substances. On the other hand, a high level of autophagy can damage organelles and cause them to transform into autophagy death (48). In our study, we also proved that cell autophagy was notably enhanced in both OGD/R HK-2 cells and RIRI model rats, and autophagy activation could induce autophagy and lower necrosis which were mediated by TRPC6 and Zn2+. Therefore, we speculated that cell autophagy was activated in OGD/R HK-2 cells or RIRI model rats to exert a role in self-protection, and overexpression of TRPC6 could further enhance the protective effect of autophagy on cells.
Conclusions
Our findings suggest TRPC6 can promote RIRI progression by activating Zn2+ influx and autophagy, which might provide new ideas and therapeutic targets for RIRI therapy.
Acknowledgments
Funding: This work was supported by National Natural Science Foundation of China (No. 81570610)
Footnote
Reporting Checklist: The authors have completed the ARRIVE reporting checklist. Available at https://atm.amegroups.com/article/view/10.21037/atm-21-5837/rc
Data Sharing Statement: Available at https://atm.amegroups.com/article/view/10.21037/atm-21-5837/dss
Conflicts of Interest: All authors have completed the ICMJE uniform disclosure form (available at https://atm.amegroups.com/article/view/10.21037/atm-21-5837/coif). The authors have no conflicts of interest to declare.
Ethical Statement: The authors are accountable for all aspects of the work in ensuring that questions related to the accuracy or integrity of any part of the work are appropriately investigated and resolved. Animal experiments were approved by the Animal Care and Use Committee of the Army Medical University [with two institutional licenses SCXK(YU)20170002 and SYXK(YU)20170002], in compliance with the guidelines of the Army Medical University for the care and use of animals.
Open Access Statement: This is an Open Access article distributed in accordance with the Creative Commons Attribution-NonCommercial-NoDerivs 4.0 International License (CC BY-NC-ND 4.0), which permits the non-commercial replication and distribution of the article with the strict proviso that no changes or edits are made and the original work is properly cited (including links to both the formal publication through the relevant DOI and the license). See: https://creativecommons.org/licenses/by-nc-nd/4.0/.
References
- Ni X, Liao Y, Li L, et al. Therapeutic role of long non-coding RNA TCONS_00019174 in depressive disorders is dependent on Wnt/β-catenin signaling pathway. J Integr Neurosci 2018;17:125-32. [Crossref] [PubMed]
- Yan HF, Tuo QZ, Yin QZ, et al. The pathological role of ferroptosis in ischemia/reperfusion-related injury. Zool Res 2020;41:220-30. [Crossref] [PubMed]
- Kezić A, Stajic N, Thaiss F. Innate Immune Response in Kidney Ischemia/Reperfusion Injury: Potential Target for Therapy. J Immunol Res 2017;2017:6305439. [Crossref] [PubMed]
- Shiva N, Sharma N, Kulkarni YA, et al. Renal ischemia/reperfusion injury: An insight on in vitro and in vivo models. Life Sci 2020;256:117860. [Crossref] [PubMed]
- Dietrich A, Gudermann T. TRPC6. Handb Exp Pharmacol 2007;125-41. [Crossref] [PubMed]
- Shen B, Mei M, Pu Y, et al. Necrostatin-1 Attenuates Renal Ischemia and Reperfusion Injury via Meditation of HIF-1α/mir-26a/TRPC6/PARP1 Signaling. Mol Ther Nucleic Acids 2019;17:701-13. [Crossref] [PubMed]
- Mwanjewe J, Grover AK. Role of transient receptor potential canonical 6 (TRPC6) in non-transferrin-bound iron uptake in neuronal phenotype PC12 cells. Biochem J 2004;378:975-82. [Crossref] [PubMed]
- Chevallet M, Jarvis L, Harel A, et al. Functional consequences of the over-expression of TRPC6 channels in HEK cells: impact on the homeostasis of zinc. Metallomics 2014;6:1269-76. [Crossref] [PubMed]
- Guo L, Li P, Meng C, et al. Protective effect of zinc on mouse renal ischemia-reperfusion injury by anti-apoptosis and antioxidation. Curr Pharm Biotechnol 2014;15:577-82. [Crossref] [PubMed]
- Rao K, Sethi K, Ischia J, et al. Protective effect of zinc preconditioning against renal ischemia reperfusion injury is dose dependent. PLoS One 2017;12:e0180028. [Crossref] [PubMed]
- Moslemi F, Talebi A, Nematbakhsh M. Protective Effect of Zinc Supplementation on Renal Ischemia/Reperfusion Injury in Rat: Gender-related Difference. Int J Prev Med 2019;10:68. [Crossref] [PubMed]
- Barekat F, Talebi A, Nematbakhsh M. The protective roles of zinc and estradiol in renal ischemia/reperfusion injury in ovariectomized rats. J Nephropathol 2018;7:88-92. [Crossref]
- Ogawa T, Mimura Y. Antioxidant effect of zinc on acute renal failure induced by ischemia-reperfusion injury in rats. Am J Nephrol 1999;19:609-14. [Crossref] [PubMed]
- Hadj Abdallah N, Baulies A, Bouhlel A, et al. Zinc mitigates renal ischemia-reperfusion injury in rats by modulating oxidative stress, endoplasmic reticulum stress, and autophagy. J Cell Physiol 2018;233:8677-90. [Crossref] [PubMed]
- Levine B, Kroemer G. Biological Functions of Autophagy Genes: A Disease Perspective. Cell 2019;176:11-42. [Crossref] [PubMed]
- Yan X, Zhou R, Ma Z. Autophagy-Cell Survival and Death. Adv Exp Med Biol 2019;1206:667-96. [Crossref] [PubMed]
- Pottier M, Dumont J, Masclaux-Daubresse C, et al. Autophagy is essential for optimal translocation of iron to seeds in Arabidopsis. J Exp Bot 2019;70:859-69. [PubMed]
- Li L, Liu Z, Jiang YY, et al. Acetylcholine suppresses microglial inflammatory response via α7nAChR to protect hippocampal neurons. J Integr Neurosci 2019;18:51-6. [PubMed]
- Riccio A, Medhurst AD, Mattei C, et al. mRNA distribution analysis of human TRPC family in CNS and peripheral tissues. Brain Res Mol Brain Res 2002;109:95-104. [Crossref] [PubMed]
- Weissmann N, Sydykov A, Kalwa H, et al. Activation of TRPC6 channels is essential for lung ischaemia-reperfusion induced oedema in mice. Nat Commun 2012;3:649. [Crossref] [PubMed]
- Du W, Huang J, Yao H, et al. Inhibition of TRPC6 degradation suppresses ischemic brain damage in rats. J Clin Invest 2010;120:3480-92. [Crossref] [PubMed]
- Wang X, Teng L, Li A, et al. TRPC6 channel protects retinal ganglion cells in a rat model of retinal ischemia/reperfusion-induced cell death. Invest Ophthalmol Vis Sci 2010;51:5751-8. [Crossref] [PubMed]
- Shen B, He Y, Zhou S, et al. TRPC6 May Protect Renal Ischemia-Reperfusion Injury Through Inhibiting Necroptosis of Renal Tubular Epithelial Cells. Med Sci Monit 2016;22:633-41. [Crossref] [PubMed]
- Li JR, Ou YC, Wu CC, et al. Ischemic preconditioning improved renal ischemia/reperfusion injury and hyperglycemia. IUBMB Life 2019;71:321-9. [Crossref] [PubMed]
- Shen Y, Qiu T, Liu XH, et al. Renal ischemia-reperfusion injury attenuated by splenic ischemic preconditioning. Eur Rev Med Pharmacol Sci 2018;22:2134-42. [PubMed]
- Zhao H, Alam A, Soo AP, et al. Ischemia-Reperfusion Injury Reduces Long Term Renal Graft Survival: Mechanism and Beyond. EBioMedicine 2018;28:31-42. [Crossref] [PubMed]
- Zhang T, Song N, Fang Y, et al. Delayed Ischemic Preconditioning Attenuated Renal Ischemia-Reperfusion Injury by Inhibiting Dendritic Cell Maturation. Cell Physiol Biochem 2018;46:1807-20. [Crossref] [PubMed]
- Xu J, Ma L, Fu P. Eriocitrin attenuates ischemia reperfusion-induced oxidative stress and inflammation in rats with acute kidney injury by regulating the dual-specificity phosphatase 14 (DUSP14)-mediated Nrf2 and nuclear factor-κB (NF-κB) pathways. Ann Transl Med 2021;9:350. [Crossref] [PubMed]
- Hall G, Wang L, Spurney RF. TRPC Channels in Proteinuric Kidney Diseases. Cells 2019;9:44. [Crossref] [PubMed]
- Staruschenko A, Spires D, Palygin O. Role of TRPC6 in Progression of Diabetic Kidney Disease. Curr Hypertens Rep 2019;21:48. [Crossref] [PubMed]
- Bai Y, Yu X, Chen H, et al. Structural basis for pharmacological modulation of the TRPC6 channel. Elife 2020;9:53311. [Crossref] [PubMed]
- Jardin I, Nieto J, Salido GM, et al. TRPC6 channel and its implications in breast cancer: an overview. Biochim Biophys Acta Mol Cell Res 2020;1867:118828. [Crossref] [PubMed]
- Lin BL, Matera D, Doerner JF, et al. In vivo selective inhibition of TRPC6 by antagonist BI 749327 ameliorates fibrosis and dysfunction in cardiac and renal disease. Proc Natl Acad Sci U S A 2019;116:10156-61. [Crossref] [PubMed]
- Gibon J, Tu P, Bohic S, et al. The over-expression of TRPC6 channels in HEK-293 cells favours the intracellular accumulation of zinc. Biochim Biophys Acta 2011;1808:2807-18. [Crossref] [PubMed]
- Tu P, Gibon J, Bouron A. The TRPC6 channel activator hyperforin induces the release of zinc and calcium from mitochondria. J Neurochem 2010;112:204-13. [Crossref] [PubMed]
- Wei G, Hough CJ, Li Y, et al. Characterization of extracellular accumulation of Zn2+ during ischemia and reperfusion of hippocampus slices in rat. Neuroscience 2004;125:867-77. [Crossref] [PubMed]
- Helal GK. Systemic administration of Zn2+ during the reperfusion phase of transient cerebral ischaemia protects rat hippocampus against iron-catalysed postischaemic injury. Clin Exp Pharmacol Physiol 2008;35:775-81. [Crossref] [PubMed]
- O'Kane D, Gibson L, May CN, et al. Zinc preconditioning protects against renal ischaemia reperfusion injury in a preclinical sheep large animal model. Biometals 2018;31:821-34. [Crossref] [PubMed]
- Wang G, Huang H, Zheng H, et al. Zn2+ and mPTP Mediate Endoplasmic Reticulum Stress Inhibition-Induced Cardioprotection Against Myocardial Ischemia/Reperfusion Injury. Biol Trace Elem Res 2016;174:189-97. [Crossref] [PubMed]
- Wang Y, Zhang H. Regulation of Autophagy by mTOR Signaling Pathway. Adv Exp Med Biol 2019;1206:67-83. [Crossref] [PubMed]
- Ravanan P, Srikumar IF, Talwar P. Autophagy: The spotlight for cellular stress responses. Life Sci 2017;188:53-67. [Crossref] [PubMed]
- Choi ME. Autophagy in Kidney Disease. Annu Rev Physiol 2020;82:297-322. [Crossref] [PubMed]
- Kocaturk NM, Akkoc Y, Kig C, et al. Autophagy as a molecular target for cancer treatment. Eur J Pharm Sci 2019;134:116-37. [Crossref] [PubMed]
- Srinivasan S, Treacy R, Herrero T, et al. Discovery and Verification of Extracellular miRNA Biomarkers for Non-invasive Prediction of Pre-eclampsia in Asymptomatic Women. Cell Rep Med 2020;1:100013. [Crossref] [PubMed]
- Ou L, Lin S, Song B, et al. The mechanisms of graphene-based materials-induced programmed cell death: a review of apoptosis, autophagy, and programmed necrosis. Int J Nanomedicine 2017;12:6633-46. [Crossref] [PubMed]
- Decuypere JP, Ceulemans LJ, Agostinis P, et al. Autophagy and the Kidney: Implications for Ischemia-Reperfusion Injury and Therapy. Am J Kidney Dis 2015;66:699-709. [Crossref] [PubMed]
- Kaushal GP, Shah SV. Autophagy in acute kidney injury. Kidney Int 2016;89:779-91. [Crossref] [PubMed]
- Hou J, Rao M, Zheng W, et al. Advances on Cell Autophagy and Its Potential Regulatory Factors in Renal Ischemia-Reperfusion Injury. DNA Cell Biol 2019;38:895-904. [Crossref] [PubMed]
(English Language Editor: B. Draper)