Jian Pi Tiao Gan Yin alleviates obesity phenotypes through mTORC1/SREBP1 signaling in vitro and in vivo
Introduction
Obesity is a prevalent phenomenon in modern society and is characterized by the accumulation of body fat due to chronic excessive energy intake against energy expenditure (1,2). Obesity is considered a serious risk factor for multiple metabolic syndromes and chronic diseases, such as type 2 diabetes (3), hypertension (4), cardiovascular diseases (5), and arthritis (6), and emerging evidence even implies a correlation between obesity and poor prognosis of cancer patients (7). Therefore, it is a primary issue to decipher mechanisms for obesity thoroughly and explore effective treatments.
Rapamycin (mTOR) mainly functions by interacting with different subunits, raptor and rictor, to form the mechanistic target of rapamycin complex 1 (mTORC1) and mTORC2 complexes, respectively (8). It has been reported that depletion of raptor abolishes mTORC1 activity (9). Accumulating studies have revealed that mTORC1 participates in various metabolic processes, such as lipogenesis (10), protein synthesis (11), autophagy (12), and energy metabolism (13). Peterson and colleagues reported that inhibition of mTORC1 in the liver could impair SREBP (sterol regulatory element-binding protein) function and cause resistance to high-fat and high-cholesterol diet-induced mouse hepatic steatosis and hypercholesterolemia (14). Notably, mTORC1 is notably activated in adipose tissues from high-fat diet (HFD)-fed and obese mice (15,16). Moreover, multiple studies have indicated that excessive activation of the mTORC1 complex leads to obesity (17,18), and inhibition of mTORC1 results in the browning of white adipose tissue (WAT), promotes thermogenesis and alleviates HFD-induced insulin resistance and obesity in a mouse model (19,20). Therefore, targeting mTOR signaling in adipose tissue is a promising approach for the treatment of obesity.
The dialectical mode of traditional Chinese medicine diagnosis of obesity includes stomach and intestine excess heat, spleen deficiency, liver qi stagnation, spleen and kidney yang deficiency, and liver and kidney yin deficiency (21). Jian Pi Tiao Gan Yin (JPTGY), a Chinese herb preparation, is used to treat obesity of liver qi stagnation and spleen deficiency. The JPTGY prescription consists of Huang-Qi [Radix Astragali, roots of Astragalus membranaceus (Fisch.) Bge. var. mongholicus (Bge.) Hsiao or Astragalus mem-branaceus (Fisch.) Bge., 30 g], Chai-Hu (Radix Bupleuri, the root of Bupleurum falcatum Linne, 12 g), Fu-Ling [Poria cocos (Schw.) Wolf., 15 g], Bai-Shao (Radix Paeoniae Alba, the root of Paeonia lactiflora Pall, 15 g), Yi-Mi (Coix seed, seed of Coix lacryma-jobi, 15 g), Dan-Shen (Salvia miltiorrhiza, the root and rhizome of Salvia miltiorrhiza Bge, 15 g), Pei-Lan (Herba Eupatorii, Eupatorium fortunei Turcz, 15 g), Jue-Ming-Zi [Semen Cassiae, the seed of Senna obtusifolia (L.) H. S. Irwin & Barneby, 15 g], Ze-Xie [Rhizoma Alismatis, the tuber of Alisma orientale (Sam.) Juzep (Alismaceae), 12 g], Shu-Da-Huang (Cooked Rhubarb, Radix et Rhizoma Rheum, the root and rhizome of Rheum palmatum, Rheum tanguticum and Rheum officinale, 6 g), and Shan-Zha (Crataegi Fructus, the fruit of Crataegus pinnatifida Bge. var. major N.E.Br., 12 g). In our previous study, JPTGY has a good weight loss effect on patients with simple obesity, which can increase the level of nesfatin-1 in serum and effectively improve lipid metabolism (22). JPTGY plays a therapeutic role by adjusting the intestinal flora in obese mice, its mechanism being related to the gut microbiota-fat signal axis (23). The mechanism of action of JPTGY in obesity remains unclear.
In this work, we applied JPTGY to a HFD obesity mouse model and found that JPTGY promoted the browning of WAT and thermogenesis through mTORC1/SREBP1 signaling, which led to alleviated obesity. Our work provides novel supporting evidence for applying traditional Chinese medicine in the prevention and therapy of obesity. We present the following article in accordance with the ARRIVE reporting checklist (available at https://atm.amegroups.com/article/view/10.21037/atm-22-685/rc).
Methods
Mice model
To establish the obesity mouse model, C57BL/6J mice (3–4 weeks old, n=40) were obtained from Charles River Laboratory (Beijing, China). The experiment was carried out according to our previous study (24). Mice were randomly divided into 2 groups: the control group (n=10), which was fed a normal diet; and the obesity group (n=30), which was fed a standard HFD (60% HFD, Research Diet, D12492, USA). After eight weeks, a calculated obesity degree [(Wobesity − Wcontrol)/Wcontrol]] over 20% was regarded as a successful model. After that, the mice were divided into the following groups and treated as indicated for 4 weeks: control (treatment with saline), obesity (treatment with saline), and JPTGY (treatment with JPTGY at 12 g/6 mL/kg). JPTGY was obtained from Shandong Provincial Qianfoshan Hospital. Saline and JPTGY were administered by gavage twice a day at 8 am and 4 pm, respectively. Mice in the MHY group were intraperitoneally injected with mTOR agonist (MHY1485, MCE, USA) twice a week (2 mg/kg) at 12 am on Wednesday and Sunday. The experiment was performed in accordance with the National Research Council Guide for the Care and Use of Laboratory Animals (25) and was approved by Affiliated Hospital of Shandong University of Traditional Chinese Medicine (No. AWE-2020-046). A protocol was prepared before the study without registration.
Physiological observations
The body weight, food and water intake, and rectal temperature were recorded manually. Brown adipose tissue (BAT) and abdominal WAT were isolated from mice after euthanasia and weighed.
Serum parameters
Blood samples were collected at the end of experiments. Blood glucose was measured by an automatic biochemical analyzer (AU5800, Beckman, USA). Serum insulin, total cholesterol (TC) and total triglyceride (TG) were measured using a mouse insulin ELISA kit (SEKM-0141, Solarbio, Beijing China), a mouse TC ELISA kit (SP14914, Saipei, Wuhan, China) and a mouse TG ELISA kit (SP14979, Saipei, Wuhan, China), respectively.
Histological analysis
Liver tissues were isolated from mice after experiments, fixed in 4% polyformaldehyde, dehydrated, embedded in paraffin, and dissected into 4-μm slices. The tissue slices were stained with hematoxylin and eosin for 30 sec and Oil Red O working solution (C0157S, Beyotime) for 30 min. Images of five randomly selected sections of each group were photographed using an optical microscope (Olympus, Japan).
Cell culture and treatment
3T3-L1 cells were obtained from Cobioer Biosciences Co., Ltd. (Nanjing, China) and maintained in Dulbecco’s modified Eagle’s medium (DMEM, Gibco, USA) supplemented with 10% NBCS (Gibco). To induce cell differentiation into adipocytes, cells were grown to 100% confluence and cultured with a mixture of hormones consisting of 0.5 mM 3-isobutyl-1-methylxanthine (Sigma-Aldrich, St. Louis, MO, USA), 1 mg/mL insulin (Sigma) and 1 mM dexamethasone (Sigma-Aldrich) for 2 days. Subsequently, the cells were maintained in DMEM containing insulin (1 mg/mL) for another 6 days for absolute differentiation, and the medium was changed every 2 days. For cell transfection, differentiated 3T3-L1 cells were placed in 6-well plates and transfected with siSREBP, pcDNA3.1-SREBP or the corresponding negative controls (Gene Pharma, China). Cells were treated with palmitic acid (0.6 mM, Sigma-Aldrich) for 24 h to induce lipid accumulation.
Western blotting
Proteins were extracted from cells and abdominal adipose tissue using ice-cold RIPA buffer. Total proteins (30 μg) were separated by SDS–PAGE and then transferred onto PVDF membranes. The protein bands were incubated with primary antibodies against the following proteins: PGC-1α (ab188102, 1:1,000), UCP-1 (ab234430, 1:1,000), AGT (ab213705, 1:1,000), PSAT (ab232944, 1:1,000), EDNRA (ab117521, 1:1,000), mTOR (ab134903, 1:1,000), p-mTOR (ab109268, 1:1,000, Abcam), Raptor (ab40768, 1:1,000, Abcam), SREBP (ab28481, 1:1,000, Abcam), FAS (ab82419, 1:1,000, Abcam), PRDM16 (PA5-20872, 1:1,000, Thermo), PPARγ (ab272718, 1:1,000, Abcam), C/EBPα (ab32358, 1:1,000, Abcam), and GAPDH (ab8245, 1:1,000, Abcam). The next day, the bands were incubated with anti-mouse (ab6728) or anti-rabbit (ab6721) secondary antibodies for 1 h at room temperature. The visualization of proteins was detected using an ECL kit and a gel imaging system (Tanon Science & Technology Co., Ltd., China).
Serum preparation
Serum containing JPTGY was prepared for the treatment of 3T3L1 cells. In brief, male C57BL/6J mice (n=20) were fed a normal diet for two days and then randomly separated into two groups (control and JPTGY groups). The mice in the JPTGY group were administered JPTGY (12 g/6 mL/kg) through gavage at 8 am and 4 pm every day for 3 days, followed by fasting for 12 h. Thirty minutes after gavage with JPTGY again, the abdomen was opened, and aortic blood was collected. The blood samples were left to set for 2 h, followed by centrifugation at 3,000 rpm for 10 min. Then, the serum was collected and stored at −70 ℃ for further experiments. For in vitro study, cells were treated with 2.5%, 5%, or 10% JPTGY drug-containing serum (JPTGY serum) or serum obtained from control (control serum).
Statistical analysis
All data are shown as the mean ± standard deviation (SD) of triplicate experiments. The statistical analysis was conducted using GraphPad Prism 7 (GraphPad Software, Inc.). Data were statistically analyzed using Student’s t-test and one-way analysis of variance (ANOVA). P values <0.05 were considered significant.
Results
JPTGY treatment ameliorates HFD-induced fat accumulation and enhances the basal metabolic rate in mice
To assess the effect of JPTGY on obesity, we constructed an HFD-induced obesity mouse model and observed the enhanced body weight of obese mice compared with the control group (Figure 1A). We then validated that body weight (Figure 1B), food intake (Figure 1C), and water intake (Figure 1D) were slightly affected by JPTGY treatment in the mice. Interestingly, rectal temperature was increased in obese mice, and JPTGY treatment further induced this phenotype (Figure 1E). Blood glucose was enhanced in obese mice, and JPTGY attenuated the enhancement in the model (Figure 1F). Moreover, the levels of serum insulin (Figure 1G), TC (Figure 1H), and TG (Figure 1I) were increased in obese mice, while JPTGY treatment reversed these effects in the model. Similarly, the weight of abdominal adipose tissue was enhanced in obese mice, and JPTGY blocked this enhancement (Figure 1J). Consistently, fat degeneration, along with fat accumulation in liver tissues, was attenuated by JPTGY treatment in obese mice (Figure 1K,1L). Collectively, these results indicate that JPTGY treatment ameliorates HFD-induced fat accumulation and enhances the basal metabolic rate in mice.
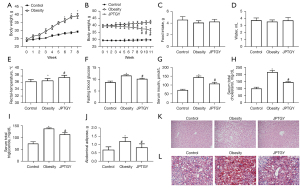
JPTGY treatment alters the protein expression levels of biomarkers in BAT and WAT in mice after treatment with JPTGY
Next, we further evaluated the impact of JPTGY on biomarkers of BAT and WAT. Significantly, the protein levels of BAT biomarkers, including PGC-1α and UCP-1 (Figure 2A), were repressed in obese mice, and treatment with JPTGY rescued the levels of BAT biomarkers in the mice. Meanwhile, the protein expression of WAT biomarkers, such as AGT, PSTA, and EDNRA, was induced in obese mice, while JPTGY treatment attenuated this induction (Figure 2B).
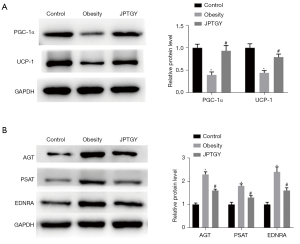
JPTGY suppresses mouse obesity through mTORC1 signaling in vivo
We then explored the potential mechanism of JPTGY-mediated obesity in mice. Given that mTORC1 signaling has been identified to regulate obesity, we investigated the effect of cotreatment of JPTGY with the mTOR activator MHY on obesity-related phenotypes in an HFD-induced obesity mouse model. The enhanced body weight of obese mice was repressed by JPTGY but increased by MHY (Figure 3A). Food intake (Figure 3B), water intake (Figure 3C), and rectal temperature (Figure 3D) were slightly affected by JPTGY treatment in the mice. Interestingly, the increased levels of fasting blood glucose (Figure 3E), serum insulin (Figure 3F), total cholesterol (Figure 3G), total triglycerides (Figure 3H), and abdominal adipose tissue (Figure 3I) in obese mice were suppressed by JPTGY treatment but enhanced by MHY treatment, in which MHY reversed the effect of JPTGY in the model.
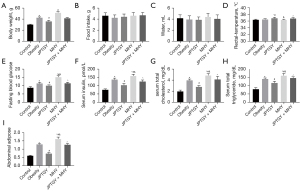
JPTGY regulates biomarkers of BAT and WAT through mTORC1 signaling in vivo
We also observed that the reduced expression of UCP-1 and PGC-1α was rescued by JPTGY treatment and was further inhibited by MHY treatment, in which MHY cotreatment attenuated the effect of JPTGY in the mice (Figure 4A). Meanwhile, the enhanced expression of AGT, PSTA, and EDNRA was inhibited by JPTGY treatment and was further increased by MHY treatment, while MHY cotreatment relieved the effect of JPTGY in the model (Figure 4A). In addition, mTOR phosphorylation and raptor, SREBP, and FAS expression levels were increased in obese mice, and JPTGY treatment inhibited but MHY upregulated the phenotypes of the mice, while MHY cotreatment blocked the impact of JPTGY in the model (Figure 4B).
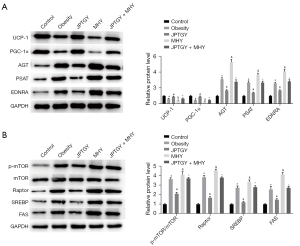
Serum containing JPTGY affects browning of 3T3-L1 cells
We then verified the function of JPTGY in the modulation of JPTGY in 3T3-L1 cells. To this end, 2.5%, 5%, or 10% serum containing JPTGY was used to treat 3T3-L1 cells, and the biomarkers of browning were analyzed in the cells. Significantly, the expression levels of UCP-1, PGC-1α, and PRDM16 were repressed and the expression levels of PARPγ and C/EBPα were enhanced in 3T3-L1 cells under palmitic acid treatment, while JPTGY treatment dose-dependently reversed these results in the cells (Figure 5).
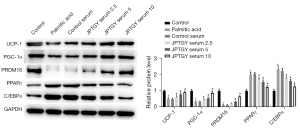
Serum containing JPTGY affects browning of 3T3-L1 cells through mTORC1 signaling
We found that the expression levels of UCP-1 and PGC-1α were inhibited in palmitic acid-treated 3T3-L1 cells and that JPTGY treatment rescued the expression, but MHY further repressed the expression in the cells, in which MHY cotreatment attenuated the effect of JPTGY in the system (Figure 6A). In addition, the phosphorylation of m-TOR and the expression levels of raptor, SREBP, and FAS were upregulated in palmitic acid-treated 3T3-L1 cells; treatment with JPTGY blocked their expression, but MHY further enhanced their expression, while cotreatment with MHY relieved the impact of JPTGY in the cells (Figure 6B).
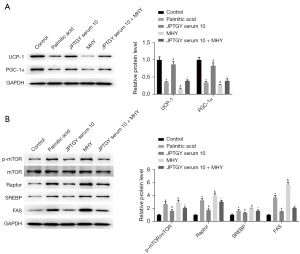
JPTGY regulates 3T3-L1 cell function via SREBP
Interestingly, we further observed that the overexpression of SREBP failed to affect the expression of UCP-1 and PGC-1α in control and palmitic acid-treated 3T3-L1 cells, while the overexpression of SREBP blocked JPTGY-induced expression of UCP-1 and PGC-1α in 3T3-L1 cells (Figure 7A). Moreover, overexpression enhanced SREBP and FAS expression in control and palmitic acid-treated 3T3-L1 cells and rescued JPTGY-inhibited expression of SREBP and FAS in the cells (Figure 7B).
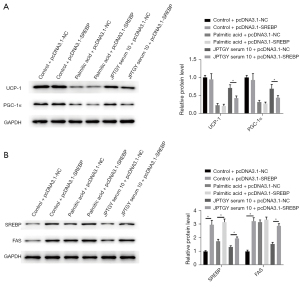
Consistently, the depletion of SREBP by siRNA enhanced the expression of UCP-1 and PGC-1α in palmitic acid-treated but not control 3T3-L1 cells and increased JPTGY-induced expression of UCP-1 and PGC-1α in 3T3-L1 cells (Figure 8A). In addition, the silencing of SREBP suppressed the expression of SREBP and FAS in control and palmitic acid-treated 3T3-L1 cells and further inhibited JPTGY-repressed SREBP and FAS expression in the cells (Figure 8B).
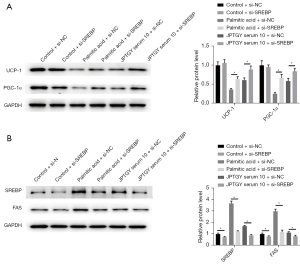
Discussion
Multiple traditional Chinese preparations have been identified to modulate obesity. For example, Jiao-Tai-Wan regulates insulin resistance and inflammation in an obesity rat model with chronic partial sleep deprivation (26). Shaofu Zhuyu decoction ameliorates obesity-induced systemic inflammation and hepatic steatosis by regulating inflammatory cytokine and adipokine levels in the circulation and various tissues (27). In the present work, JPTGY suppressed obesity in HFD-induced obese mice.
The mechanical investigation of this study showed that the enhanced levels of fasting blood glucose, serum insulin, TC, TG, and abdominal adipose tissue in obese mice were suppressed by JPTGY treatment. Adipose tissue mainly consists of WAT, BAT, and beige adipose tissue (28). Obesity occurs due to the accumulation of WAT (29). Lipogenesis involves fatty acid synthesis and TG synthesis (30). In BAT, PGC1-α, which coactivates members of PPARγ that modulate the expression of UCP1, enhances thermogenesis (31). A previous study showed that spirulina maxima 70% ethanol extract treatment reduces obesity by upregulating the expression of proteins in the thermogenic program, including PRDM16, PGC-1α, and UCP1, in WAT and BAT (32). Our findings were consistent in vitro and in vivo. The increased expression levels of adipogenic-specific proteins, including SREBP, C/EBPα, and PPARγ, and the lipogenic enzyme FAS, are associated with HFD-induced obesity (33). In the present work, the expression levels of SREBP, PPARγ, C/EBPα, and FAS were decreased in JPTGY-treated 3T3-L1 cells. Similarly, muscat bailey A grape stalk extract attenuated adipogenic differentiation by downregulating C/EBPα and PPARγ (34). Diphlorethohydroxycarmalol regulates lipid metabolism by reducing the expression levels of adipogenic-specific proteins and lipogenic enzymes, including PPARγ, C/EBPα, SREBP-1c, FABP4, and FAS, in epididymal adipose tissue (35).
Targeting mTOR may potentially relieve the development of obesity (36). Empagliflozin relieves obesity-associated heart dysfunction by modulating sestrin2-regulated mTOR signaling in an HFD-induced obesity mouse model (37). Rapamycin attenuates age-related obesity by modulating mTOR signaling (38). Rubrofusarin-6-β-gentiobioside represses weight gain and lipid accumulation by targeting mTOR signaling (39). Maternal obesity is associated with the stimulation of mTOR signaling and placental insulin in a mouse model (40). In the present work, mTOR phosphorylation was increased in obese mice, and JPTGY treatment was inhibited, while MHY cotreatment blocked the impact of JPTGY in vitro and in vivo. Further research is needed to clarify the anti-obesity signaling pathway of JPTGY.
We identified that JPTGY relieved obesity phenotypes through mTORC1/SREBP1 signaling in vitro and in vivo. JPTGY may benefit the attenuation of obesity. We concluded that JPTGY possesses strong in vitro and in vivo anti-obesity activities and it could be used as a potential therapeutic agent for attenuating obesity.
Acknowledgments
Funding: This work was supported by the National Natural Science Foundation of China (No. 81704026) and the Natural Science Foundation of Shandong Province (No. ZR2016HB04).
Footnote
Reporting Checklist: The authors have completed the ARRIVE reporting checklist. Available at https://atm.amegroups.com/article/view/10.21037/atm-22-685/rc
Data Sharing Statement: Available at https://atm.amegroups.com/article/view/10.21037/atm-22-685/dss
Conflicts of Interest: All authors have completed the ICMJE uniform disclosure form (available at https://atm.amegroups.com/article/view/10.21037/atm-22-685/coif). All authors report that this study was supported by the National Natural Science Foundation of China (81704026) and the Natural Science Foundation of Shandong Province (ZR2016HB04). The authors have no other conflicts of interest to declare.
Ethical Statement: The authors are accountable for all aspects of the work in ensuring that questions related to the accuracy or integrity of any part of the work are appropriately investigated and resolved. The experiment was performed in accordance with the National Research Council Guide for the Care and Use of Laboratory Animals and approved by Affiliated Hospital of Shandong University of Traditional Chinese Medicine (No. AWE-2020-046).
Open Access Statement: This is an Open Access article distributed in accordance with the Creative Commons Attribution-NonCommercial-NoDerivs 4.0 International License (CC BY-NC-ND 4.0), which permits the non-commercial replication and distribution of the article with the strict proviso that no changes or edits are made and the original work is properly cited (including links to both the formal publication through the relevant DOI and the license). See: https://creativecommons.org/licenses/by-nc-nd/4.0/.
References
- Canale MP, Manca di Villahermosa S, Martino G, et al. Obesity-related metabolic syndrome: mechanisms of sympathetic overactivity. Int J Endocrinol 2013;2013:865965. [Crossref] [PubMed]
- Ghaben AL, Scherer PE. Adipogenesis and metabolic health. Nat Rev Mol Cell Biol 2019;20:242-58. [Crossref] [PubMed]
- Kohlgruber A, Lynch L. Adipose tissue inflammation in the pathogenesis of type 2 diabetes. Curr Diab Rep 2015;15:92. [Crossref] [PubMed]
- Kotsis V, Stabouli S, Papakatsika S, et al. Mechanisms of obesity-induced hypertension. Hypertens Res 2010;33:386-93. [Crossref] [PubMed]
- Kachur S, Lavie CJ, de Schutter A, et al. Obesity and cardiovascular diseases. Minerva Med 2017;108:212-28. [Crossref] [PubMed]
- Van Raemdonck K, Umar S, Szekanecz Z, et al. Impact of obesity on autoimmune arthritis and its cardiovascular complications. Autoimmun Rev 2018;17:821-35. [Crossref] [PubMed]
- Basen-Engquist K, Chang M. Obesity and cancer risk: recent review and evidence. Curr Oncol Rep 2011;13:71-6. [Crossref] [PubMed]
- Szwed A, Kim E, Jacinto E. Regulation and metabolic functions of mTORC1 and mTORC2. Physiol Rev 2021;101:1371-426. [Crossref] [PubMed]
- Sengupta S, Peterson TR, Laplante M, et al. mTORC1 controls fasting-induced ketogenesis and its modulation by ageing. Nature 2010;468:1100-4. [Crossref] [PubMed]
- Lee G, Zheng Y, Cho S, et al. Post-transcriptional Regulation of De Novo Lipogenesis by mTORC1-S6K1-SRPK2 Signaling. Cell 2017;171:1545-1558.e18. [Crossref] [PubMed]
- Villa E, Sahu U, O'Hara BP, et al. mTORC1 stimulates cell growth through SAM synthesis and m6A mRNA-dependent control of protein synthesis. Mol Cell 2021;81:2076-2093.e9. [Crossref] [PubMed]
- Davis OB, Shin HR, Lim CY, et al. NPC1-mTORC1 Signaling Couples Cholesterol Sensing to Organelle Homeostasis and Is a Targetable Pathway in Niemann-Pick Type C. Dev Cell 2021;56:260-276.e7. [Crossref] [PubMed]
- Tangseefa P, Martin SK, Chin PY, et al. The mTORC1 complex in pre-osteoblasts regulates whole-body energy metabolism independently of osteocalcin. Bone Res 2021;9:10. [Crossref] [PubMed]
- Peterson TR, Sengupta SS, Harris TE, et al. mTOR complex 1 regulates lipin 1 localization to control the SREBP pathway. Cell 2011;146:408-20. [Crossref] [PubMed]
- Khamzina L, Veilleux A, Bergeron S, et al. Increased activation of the mammalian target of rapamycin pathway in liver and skeletal muscle of obese rats: possible involvement in obesity-linked insulin resistance. Endocrinology 2005;146:1473-81. [Crossref] [PubMed]
- Paschoal VA, Amano MT, Belchior T, et al. mTORC1 inhibition with rapamycin exacerbates adipose tissue inflammation in obese mice and dissociates macrophage phenotype from function. Immunobiology 2017;222:261-71. [Crossref] [PubMed]
- Carnevalli LS, Masuda K, Frigerio F, et al. S6K1 plays a critical role in early adipocyte differentiation. Dev Cell 2010;18:763-74. [Crossref] [PubMed]
- Laplante M, Sabatini DM. mTOR signaling in growth control and disease. Cell 2012;149:274-93. [Crossref] [PubMed]
- Liu M, Bai J, He S, et al. Grb10 promotes lipolysis and thermogenesis by phosphorylation-dependent feedback inhibition of mTORC1. Cell Metab 2014;19:967-80. [Crossref] [PubMed]
- Wada S, Neinast M, Jang C, et al. The tumor suppressor FLCN mediates an alternate mTOR pathway to regulate browning of adipose tissue. Genes Dev 2016;30:2551-64. [Crossref] [PubMed]
- Yan RH, Liu XM, Bai J, et al. Clinical efficacy of simple obesity treated by catgut implantation at acupoints. Chin J Integr Med 2015;21:594-600. [Crossref] [PubMed]
- Feng B, Zhang H, Xu X, et al. Clinical study on treating simple obesity with Jianpi Tiaogan Yin. Journal of Shandong University of Traditional Chinese Medicine 2014;548-50.
- Gu Y, Wang Z, Feng B. Effect of Spleen-Invigorating and Liver-Regulating Potion on Gut Microbiota of Obese Mice Based on High-Throughput Sequencing Data. Journal of Guangzhou University of Traditional Chinese Medicine 2020;37:2194-9.
- Feng B, Gu Y, Meng D, et al. Effects of Jianpi tiaogan Decoction on core temperature / autonomic activity and browning of white fat in simple obesity mice. Chinese Archives of Traditional Chinese Medicine 2018;36:2340-4.
- The National Academies Collection: Reports funded by National Institutes of Health. Guide for the Care and Use of Laboratory Animals. Washington (DC): National Academies Press (US), 2011.
- Zou X, Huang W, Lu F, et al. The effects of Jiao-Tai-Wan on sleep, inflammation and insulin resistance in obesity-resistant rats with chronic partial sleep deprivation. BMC Complement Altern Med 2017;17:165. [Crossref] [PubMed]
- Hong M, Jung J, Park HS, et al. Shaofu Zhuyu decoction ameliorates obesity-mediated hepatic steatosis and systemic inflammation by regulating metabolic pathways. PLoS One 2017;12:e0178514. [Crossref] [PubMed]
- Wu J, Cohen P, Spiegelman BM. Adaptive thermogenesis in adipocytes: is beige the new brown? Genes Dev 2013;27:234-50. [Crossref] [PubMed]
- Vishvanath L, Gupta RK. Contribution of adipogenesis to healthy adipose tissue expansion in obesity. J Clin Invest 2019;129:4022-31. [Crossref] [PubMed]
- Moreno-Indias I, Tinahones FJ. Impaired adipose tissue expandability and lipogenic capacities as ones of the main causes of metabolic disorders. J Diabetes Res 2015;2015:970375. [Crossref] [PubMed]
- Boström P, Wu J, Jedrychowski MP, et al. A PGC1-α-dependent myokine that drives brown-fat-like development of white fat and thermogenesis. Nature 2012;481:463-8. [Crossref] [PubMed]
- Seo YJ, Kim KJ, Choi J, et al. Spirulina maxima Extract Reduces Obesity through Suppression of Adipogenesis and Activation of Browning in 3T3-L1 Cells and High-Fat Diet-Induced Obese Mice. Nutrients 2018;10:712. [Crossref] [PubMed]
- Kang MC, Ding Y, Kim HS, et al. Inhibition of Adipogenesis by Diphlorethohydroxycarmalol (DPHC) through AMPK Activation in Adipocytes. Mar Drugs 2019;17:44. [Crossref] [PubMed]
- Kim BM, Cho BO, Jang SI. Muscat Bailey A grape stalk extract ameliorates high-fat diet-induced obesity by downregulating PPARγ and C/EPBα in mice. Int J Mol Med 2019;43:489-500. [PubMed]
- Ding Y, Wang L, Im S, et al. Anti-Obesity Effect of Diphlorethohydroxycarmalol Isolated from Brown Alga Ishige okamurae in High-Fat Diet-Induced Obese Mice. Mar Drugs 2019;17:637. [Crossref] [PubMed]
- Wang Y, Diao S, Hu M, et al. Methylation of Hypothalamic Tsc1-mTOR Signaling in Regulation of Obesity and Obesity Resistance. Biomed Res Int 2020;2020:8723869. [Crossref] [PubMed]
- Sun X, Han F, Lu Q, et al. Empagliflozin Ameliorates Obesity-Related Cardiac Dysfunction by Regulating Sestrin2-Mediated AMPK-mTOR Signaling and Redox Homeostasis in High-Fat Diet-Induced Obese Mice. Diabetes 2020;69:1292-305. [Crossref] [PubMed]
- Yang SB, Tien AC, Boddupalli G, et al. Rapamycin ameliorates age-dependent obesity associated with increased mTOR signaling in hypothalamic POMC neurons. Neuron 2012;75:425-36. [Crossref] [PubMed]
- Han YH, Kee JY, Park SH, et al. Rubrofusarin-6-beta-gentiobioside inhibits lipid accumulation and weight gain by regulating AMPK/mTOR signaling. Phytomedicine 2019;62:152952. [Crossref] [PubMed]
- Rosario FJ, Powell TL, Jansson T. Activation of placental insulin and mTOR signaling in a mouse model of maternal obesity associated with fetal overgrowth. Am J Physiol Regul Integr Comp Physiol 2016;310:R87-93. [Crossref] [PubMed]