A mouse model of exertional heatstroke-related acute kidney injury
Introduction
Exertional heatstroke (EHS) is a life-threatening emergency defined as a core body temperature >40 ℃ and central nervous system dysfunction. EHS often occurs if an individual engages in intense exercise in a hot environment (1,2). The clinical mortality rate of EHS is high (approximately 70%) (3). EHS is likely to be complicated by acute kidney injury (AKI), which is referred to as EHS-related AKI or EHS-AKI (4,5). A study has shown that the risk factors for acute kidney injury in heatstroke patients included age 20–39 years, African American race, obesity, chronic kidney disease, congestive heart failure, and rhabdomyolysis (4). The incidence of AKI in EHS has been shown to be as high as 90.9% (6). AKI often manifests as oliguria, electrolyte imbalance, and elevated creatinine (Cr) levels, and requires continuous renal replacement therapy (6,7). The mechanism underlying the occurrence of AKI in EHS is not yet fully understood and may include hyperthermia, hypoperfusion, systemic inflammatory response syndrome (SIRS), disseminated intravascular coagulation (DIC), and rhabdomyolysis (8-12). Thus, a stable animal model is particularly important if the pathogenesis of EHS-AKI is to be studied in-depth.
The animal models of EHS reported in the literature include dog, rat, and mouse models (13-15). As the gene similarity between mice and humans is high, and the gene expression in mice is relatively easy to change, it may be more instructive to study EHS-AKI in mice than rats or dogs. As C57BL/6 is the most commonly used genetic background of transgenic mice, and most mouse models of EHS in the literature are also C57BL/6, we selected C57BL/6 mouse strains as the experimental objects (15-17).
To establish EHS mouse models, most studies make mice exercise in high temperatures and humidity, and the experiment is deemed successful when a target core body temperature is reached or corresponding neurological symptoms are exhibited (18,19). The shortcoming of this approach is that this experimental method may not necessarily cause AKI. For example, King et al. established an EHS mouse model under an environment temperature of 37.5 ℃/ with 50% room, humidity (Tenv 37.5 ℃/RH 50%), and the end points were a core body temperature ≥42.5 ℃ and loss of consciousness; however, the Cr level of the mice was not significantly increased. Further, the renal pathology results only showed glomerular vascular congestion, bleeding and thrombosis, but there were no typical AKI manifestations, such as tubular epithelial cell necrosis, a loss of brush borders, or cast formation (18). He et al. conducted EHS experiment on mice at Tenv 39.5 ℃/RH 65%, and while their renal pathology results showed necrosis of tubular epithelial cells at 1 day, they did not detect Cr levels, and thus the incidence and severity of AKI were not clear (20).
The kidney, as a paired organ in the body, receives and filters 20% of the cardiac output. One kidney contains up to 2.5 million nephrons, and has a strong compensatory capacity (21). Additionally, C57BL/6 mice have strong resistance to the induction of kidney injury (22). We believe that the continuous elevation of creatine kinase (CK) and myoglobin (MYO) in rhabdomyolysis plays an important role in EHS-induced AKI. However, as King and He (18,20) showered, since the half-life of CK in mice is only 1/10 of that of humans, the exercise-induced increase of CK decreases rapidly. Thus, single exercise-induced rhabdomyolysis in EHS mouse models may not mimic the pathological damage to renal tubules caused by the continued elevation of CK in humans.
Fifty percent of glycerol (in a dose of 5–10 mL/kg) is usually used to construct mouse models of rhabdomyolysis, as glycerol has a clear injury effect on muscle (23,24). In one study, CK remained at 3,000 U/L 24 h after the injection of 10 mL/kg glycerol, resulting in AKI (24). Additionally, preexisting muscle injury is known to increase circulating levels of the pro-inflammatory cytokine of interleukin 6 (IL-6), leading to further increases in core body temperature and IL-6 levels during subsequent exercise (25,26). Thus, we hypothesized that undertaking a classical EHS experiment after treatment with a low dose of glycerol would prolong the rise time of CK and promote the inflammatory response, thus aggravating kidney injury. Finally, the kidney and muscle injuries were assessed according to the serum levels of Cr, blood urea nitrogen (BUN), CK, MYO, IL-6, and pathology results. To test our hypothesis, we designed a mouse model that induced EHS-AKI. This experimental scheme can be used to establish a mouse model that simulates human EHS-AKI, and can be used in subsequent studies. We present the following article in accordance with the ARRIVE reporting checklist (available at https://atm.amegroups.com/article/view/10.21037/atm-22-715/rc).
Methods
Experimental animals
Specific pathogen-free male wild-type C57BL/6 mice, aged 8–9 weeks, with body weights of 23–25 g, were selected (Beijing SiPeiFu Biotechnology Co. Ltd., China). A study has shown that female mice have greater resistance to EHS (15), only male mice were used in this study. The mice were housed in separate cages (4 mice per cage) under Tenv 23 ℃/RH 55%±5% with a 12-h light/dark cycle, and had free access to standard chow and water. All experimental protocols involving animals were approved by the Animal Care and Use Committee of the Chinese People’s Liberation Army General Hospital, in compliance with the National Institutes of Health Guide for the Care and Use of Laboratory Animals. A protocol was prepared before the study without registration.
Experimental design and animal preparation
The mice were randomly divided into the following 6 groups: Saline Control group, Glycerol Control group, Saline + Sham heat exercise (SHE) group, Saline + Heat exercise (HE) group, Glycerol + SHE group, and Glycerol + HE group. The Saline Control group (n=8) and Glycerol Control group (n=8) were used to provide a 0-h baseline before exercise for the Saline + SHE group (n=40), Saline + HE group, Glycerol + SHE group (n=40) and Glycerol + HE group, respectively. In the other 4 groups, 8 mice were sacrificed under anesthesia to collect samples at 6 h, 12 h, 1 day, 2 days, or 3 days after exercise. Blood, kidney and muscle samples were collected (see Figure 1). As the Saline + HE group and the Glycerol + HE group both had certain mortality, 59 mice and 93 mice, respectively, were included in these 2 groups for the experiment. Except for the Control group, 8 mice were included in each of the other 4 groups to observe the survival rate.
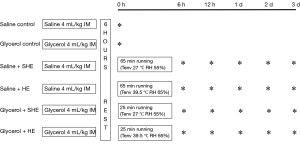
Before starting the experiments, all the mice in each group were subjected to adaptive training. Training was performed in Tenv 27±0.5 ℃/RH 55%±5% for a period of 2 weeks. The methods were as follows: the mice were run on a treadmill (YLS-10B mouse wheel fatigue tester) for 2 h every day at a speed of 15 rpm. After the completion of the adaptive training, the mice were rested for 2 days, and then began the experiment (20). The mice were deprived of water and fed freely for 24 h before the experiment, and weighed 1 h before the experiment.
Saline Control group
The mice were intramuscularly injected with 4 mL/kg of 0.9% saline in the medial thigh of left hind limb, and samples were taken 6 h later.
Glycerol Control group
The mice were intramuscularly injected with 4 mL/kg of 50% glycerol (comprising 0.9% saline and glycerol at a 1:1 volume ratio) in the medial thigh of the left hind limb, and samples were collected 6 h later.
Saline + SHE group
The mice were injected with 4 mL/kg of 0.9% saline in the medial thigh of the left hind limb, and exercise began 6 h later. After the intramuscular injection of saline or glycerol, the mice needed to rest for a period before exercise. The exercise uniformly began after 6 h. The environmental conditions of the chamber were Tenv 27 ℃/RH 55%, the exercise speed was 15 rpm, and the exercise time was 65 min (the average exercise time of the Saline + HE group). After exercise, the mice were taken out and put in the condition of Tenv 23 ℃/RH 55%±5% for recovery with free access to food and water.
Saline + HE group
The mice were injected with 4 mL/kg of 0.9% saline in the medial thigh of the left hind limb, and exercise began 6 h later. The environmental conditions were Tenv 39.5 ℃/RH 65%. The mice were placed on the treadmill wheel, and the forced running protocol was started. The exercise speed was 15 rpm. The behavior of the mice was observed, and the rectal temperature was taken. The core temperature (Tc) of the body was measured by inserting a thermal probe (BW-TH1101, Billion, Shanghai, China) 2 cm into the rectum of the mice. The endpoints were defined as follows: (I) Tc ≥42.5 ℃; and (II) a loss of consciousness: the mice had no reaction after 3 weeks of continuous rotation of the wheel (15,18). The model was considered to be successfully established if the 2 factors were satisfied at the same time. At the end of the experiment, the mice were removed and placed in a Tenv 23 ℃/RH 55%±5% environment for recovery, with free access to food and water.
Glycerol + SHE group
The mice were injected with 4 mL/kg of 50% glycerol in the medial thigh of the left hind limb, and exercise began 6 h later. The environmental condition of the chamber was Tenv 27 ℃/RH 55%, the exercise speed was 15 rpm, and the exercise time was 25 min (the average exercise time of the Glycerol + HE group). After exercise, the mice were taken out and put in the environment of Tenv 23 ℃/RH 55%±5% for recovery, with free access to food and water.
Glycerol + HE group
The mice were injected with 4 mL/kg of 50% glycerol in the medial thigh of the left hind limb, and exercise began 6 h later. The environmental conditions were Tenv 39.5 ℃/RH 65%, and the exercise speed was 15 rpm. The criteria for the end points were the same as those of the Saline + HE group. At the end of the experiment, the mice were removed and placed in an environment of Tenv 23 ℃/RH 55%±5% for recovery, with free access to food and water.
Biochemical detection
Orbital blood samples (0.6 mL) were collected and centrifuged twice (3,500 rpm, 4 ℃, 10 min). The serum was collected and detected within 2 h. Corresponding reagent kits were used to measure Cr (S03076, Rayto, China), BUN (S03036, Rayto, China), CK (S03024, Rayto, China), IL-6 (88-7064, ThermoFisher, USA), and MYO (MM-0518M1, MEIMIAN, China) levels.
Kidney pathological examination
Kidney tissues were fixed in 10% formalin for 24 h, followed by conventional dehydration and paraffin embedding. Kidney tissue sections with a thickness of 4 µm were stained with standard periodic acid-Schiff (PAS). The pathological manifestations of the kidneys were scored independently by 2 kidney pathologists. The severity of acute tubular necrosis was quantified by calculating the percentages of tubules showing cell necrosis, brush border loss, cast formation, and tubular dilatation as follows: 0: none; 1: <25%; 2: 25–50%; 3: 50–75%; and 4: >75% (27). At a magnification of ×400, 10 fields of view were randomly selected from each slide for evaluation. The left vastus medialis of each mouse was collected and fixed in 10% formalin for 24 h, followed by routine dehydration and paraffin embedding. Hematoxylin and eosin (H&E) staining was conducted and observed at ×200 magnification.
Statistical analysis
The data analysis was performed using the GraphPad Prism 7.0 software package. The survival rate is expressed as a percentage. Normally distributed data are expressed as the mean ± standard deviation. Non-normally distributed data are expressed as median ± interquartile ranges. The Kaplan-Meier method was used for the survival analysis, and the log-rank test was used for comparisons. A 1- or 2-way analysis of variance (ANOVA) was used, followed by Dunnett’s or Tukey’s multiple comparison test to clarify the significant differences between groups. The t-test was used for data with a normal distribution, and the Mann-Whitney test was used for data with a non-normal distribution. A P value <0.05 was considered statistically significant.
Results
Survival rate, experiment time, and endpoint temperature of mice
The mice in the Saline + SHE group and Glycerol + SHE group all survived for 3 days. The survival rates of the mice in the Saline + HE group were 87.50%, 75.00%, 62.50%, 62.50%, and 62.50% at 6 h, 12 h, 1 day, 2 days, and 3 days, respectively, and those of the Glycerol + HE group were 75.00%, 62.50%, 50.00%, 37.50%, and 25.00%, respectively. The survival analysis showed that there were no significant differences between the Saline + HE group and Glycerol + HE group (P=0.21; see Figure 2).
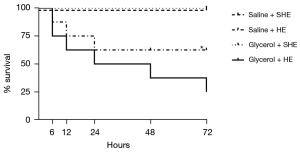
The Tc at the end point of the Glycerol + HE group (44.28±0.59 ℃; P<0.05) was higher than that of the Saline + HE group (43.34±0.38 ℃). Additionally, the Glycerol + HE group [25.50±(20.00–41.75) min; P<0.05] reached the end point in a shorter time than the Saline + HE group [65.50±(43.25–77.00) min].
Biochemical analysis
There were no significant differences in Cr, BUN, CK, and MYO kevels between the Saline Control group and Glycerol Control group at the 0-h baseline. However, the IL-6 level of the Glycerol Control group was higher than that of the Saline Control group (P<0.05; see Table 1).
Table 1
Group | Cr (μmol/L) | BUN (mmol/L) | CK (U/L) | MYO (pg/mL) | IL-6 (pg/mL) |
---|---|---|---|---|---|
Saline Control | 25.85±5.31 | 8.53±1.18 | 848.20±387.60 | 2329.00±941.20 | 2.96±1.38 |
Glycerol Control | 25.15±5.14# | 8.50±0.87# | 856.20±342.80# | 2368.00±595.00# | 22.55±10.01* |
The data are expressed as mean ± standard deviation (mean ± SD). There were two study groups: (I) Saline Control group (n=8); and (II) Glycerol Control group (n=8). *, P<0.05; #, P>0.05 (Saline Control group vs. Glycerol Control group), t-test. Cr, creatinine; BUN, blood urea nitrogen; CK, creatine kinase; MYO, myoglobin; IL-6, interleukin-6.
The Cr level of the Glycerol + HE group peaked at 12 h, and then decreased gradually, reaching 141.00±40.29 µmol/L at 12 h, and 78.01±32.54 µmol/L at 1 day, but was significantly higher than that of the Glycerol Control group at the baseline (P<0.05; see Figure 3A). The Cr level was also highest in the Saline + HE group at 12 h (40.47±12.46 µmol/L), and was higher than that in the Saline Control group at the baseline (P<0.05; see Figure 3A). There was no significant increase in the Cr levels of the Saline + SHE group and Glycerol + SHE group compared to the baseline at each time point. The Cr levels of the Glycerol + HE group were higher at 6 h, 12 h, and 1 day than those of the Saline + HE group, and higher at 6 h, 12 h, 1 day, and 2 days than those of the Glycerol + SHE group (P<0.05; see Figure 3A). AKI was diagnosed as a Cr increase 1.5 times greater than that of the baseline within 7 days in accordance with the Kidney Disease Improving Global Outcomes (KDIGO) criteria (28). Based on this analysis, the proportion of Cr higher than 1.5 times the baseline level at the peak of 12 h was 37.50% in the Saline + HE group, and 100.00% in the Glycerol + HE group.
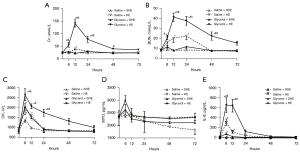
In relation to BUN, the Glycerol + HE group also peaked at 12 h (41.24±11.50 mmol/L), and then gradually decreased, but was significantly increased at 6 h, 12 h, 1 day and 2 days compared to the baseline (all P<0.05; see Figure 3B). The BUN levels of the Saline + HE group also reached 20.56±12.21 mmol/L at 12 h and 22.57±10.60 mmol/L at 1 day, and these levels were significantly higher than the baseline (P<0.05; see Figure 3B). There was no significant increase in the BUN levels of the Saline + SHE group and Glycerol + SHE group compared to the baseline at each time point. The BUN levels of the Glycerol + HE group were higher at 12 h, 1 day, and 2 days than those of the Saline + HE group, and higher at 6 h, 12 h, 1 day, and 2 days than those of the Glycerol + SHE group (P<0.05; see Figure 3B).
Compared to the baseline, the CK level of each group reached different degrees after exercise. Notably, the CK level of the Saline + SHE group peaked at 6 h (1,385.00±326.10 U/L) (P<0.05; see Figure 3C). The CK level of the Saline + HE group (1,947.00±856.30 U/L) and Glycerol + SHE group (2,058.00±372.40 U/L) also peaked at 6 h, and remained significantly different at 12 h compared to the baseline (P<0.05; see Figure 3C). However, the CK levels of the Glycerol + HE group were still higher than the baseline at 12 h and 1 day, but peaked at 6 h (2,637.00±848.10 U/L) (P<0.05; see Figure 3C). The CK levels of the Glycerol + HE group were higher at 6 h and 1 day than those of the Saline + HE group, and higher at 6 h, 12 h, and 1 day than those of the Glycerol + SHE group (P<0.05; see Figure 3C). The MYO changes after exercise in each group are shown in Figure 3D. Notably, there was no significant increase compared to the baseline (P>0.05), and no statistically significant differences between the groups.
Compared to the baseline, the IL-6 levels of the Saline + SHE group and Glycerol + SHE group peaked at 6 h (P<0.05, Figure 3E), and then gradually decreased. The IL-6 levels also peaked at 6 h in the Saline + HE group (310.90±181.10 pg/mL) and the Glycerol + HE group (649.30±408.20 pg/mL), and these levels were significantly higher than the baseline (P<0.05), and still differed at 12 h. The IL-6 levels of the Glycerol + HE group were higher at 6 h and 12 h than those of the Saline + HE group and Glycerol + SHE group (P<0.05; see Figure 3E).
Pathological examination
Neither the Saline Control group nor the Glycerol Control group showed significant impairment in renal and muscle pathology (see Figure 4).
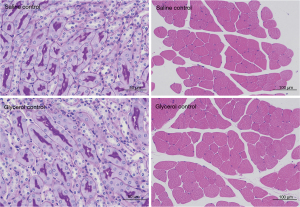
Neither the Saline + SHE group nor the Glycerol + SHE group showed significant kidney damage at any time point; thus, no injury score was calculated. In the Glycerol + HE group, typical renal injuries, such as tubular epithelial necrosis, tubular dilatation, and cast formation, were observed (see Figure 5; the black arrows indicate tubule epithelial cell necrosis, and the yellow arrows indicate tubule dilation). The pathological scores of the Glycerol + HE group at each time point were 0.79, 1.29, 1.58, 0.85, and 0.77, respectively. Slight kidney injury was also observed in the Saline + HE group, and the pathological scores at each time point were 0.00, 0.13, 0.41, 0.00, and 0.00, respectively.
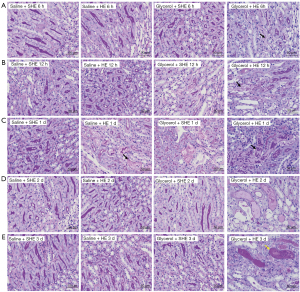
There were no obvious lesions in the left vastus medialis muscle pathology at each time point in the Saline + SHE group and the Saline + HE group. The Glycerol + SHE and Glycerol + HE groups showed local muscle damage at each time point (see Figure 6; the black arrows indicate the fragmentation of muscle fiber nuclei, the yellow arrows indicate the infiltration of lymphocytes and neutrophils, and the red arrows indicate an increased number of fibroblasts).
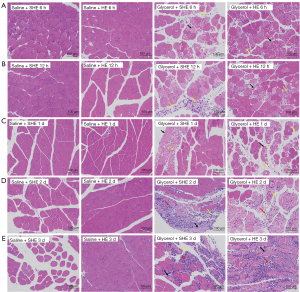
Discussion
AKI is an important organ function injury of EHS, and is closely related to death caused by EHS (4). This study sought to establish a stable EHS-AKI mouse model. Under our model scheme: (I) the mice were intramuscularly injected with 4 mL/kg of 50% glycerol in their left hind limbs 6 h before exercise; (II) the environmental condition was Tenv 39.5 ℃/RH 65%; (III) the end point was Tc ≥42.5 ℃, and a loss of consciousness. The results showed that the Cr and BUN levels of the Glycerol + HE group were significantly increased, and the mice in this group had typical AKI pathology results, including tubular epithelial cell necrosis, renal tubule dilatation, and cast formation. The results showed that a stable EHS-AKI model was successfully established. Despite optimizing an EHS-AKI model, the results indicated that this was a relatively mild model. Thus, a more severe model may be needed to evaluate the effect of drug therapy in subsequent experiments.
At present, most animal studies on EHS focus on EHS-related intestinal, heart, and liver injury, but research on EHS-related AKI has been limited (29-32). This may be because the classical EHS model does not actually simulate the renal pathological changes of human EHS-AKI; however, continuous attempts have been made to optimize EHS conditions and establish stable EHS-AKI models (18,20). Our results showed that while the Cr level at 12 h and the BUN levels at 12 h and 1 day of the Saline + HE group differed significantly from the baseline results, the increase was very low. Further, there was only slight renal pathological injury at 12 h and 1 day, with the scores of 0.13 and 0.41, respectively. According to the clinical criteria of the KDIGO, AKI is diagnosed if Cr level increases ≥26.5 µmol/L within 48 h, or is 1.5 times higher than the baseline within the first 7 days, and/or there is a urine volume of <0.5 mL/kg/h for 6–12 h (28). The main histological feature of AKI is tubular cell death (33). Our study showed that the proportion of Cr 1.5 times higher than the baseline level at peak 12 h was only 37.50% in the Saline + HE group, while the proportion of Cr in the Glycerol + HE group was 100.00%. Additionally, the renal pathology results of the Glycerol + HE group also showed typical AKI manifestations, such as tubular epithelial cell necrosis, tubular dilatation, and cast formation, with scores of 0.79, 1.29, 1.58, 0.85, and 0.77 at each time point, respectively. As a result, the Glycerol + HE group mice successfully formed significant AKI lesions with Cr peaked at 12 h and pathological damage was the worst at 1 day. In conclusion, we believe that this model can be used as a stable EHS-AKI mouse model. This EHS-AKI mice model was established by a combination of intramuscular glycerol injection and heat exercise, which was different from other etiology related AKI model establishment. For example, sepsis related AKI model was induced by cecal ligation and punctured or single intraperitoneal injection dose of 10–15 mg/kg lipopolysaccharide. Cisplatin related AKI model was induced by single does 6–20 mg/kg cisplatin. And ischemia-reperfusion related AKI was usually induced by ischemia 30–45 min and reperfusion 24–48 h (33).
Rhabdomyolysis is common in EHS. Both heat and exercise may contribute to rhabdomyolysis during EHS. CK is still the most effective indicator for identifying and measuring rhabdomyolysis (34). A study examined patients with CK >1,000 U/L in emergency rooms from 2012 to 2017, and found that 2.1% had exertional rhabdomyolysis, with CK averaging 16,884.4±41,645.6 U/L (35). Another study on the changes of clinical biomarkers in patients with EHS over 16 days showed that CK reached its peak on the 3rd day of onset, and was 4.65 times that of the normal upper limit (7). However, the muscle mass of mice was small and the half-life of CK was very short at 1.5 h, which is 1/10 of that of humans, and the changes in vivo were relatively rapid (18). Compared to the baseline level, the CK levels of the Saline + HE group and Glycerol + HE group increased at 6 and 12 h, and the CK level of the Glycerol + HE group was still higher at 1 day than the baseline. The CK levels of the Glycerol + HE group were higher than those of the Saline + HE group at 6 h and 1 day, and higher than those of the Glycerol + SHE group at 6 h, 12 h, and 1 day.
MYO is known to damage the kidney in various ways, including via renal vasoconstriction, cast formation, and direct toxicity to renal tubular cells, and is a relatively effective indicator for predicting AKI (34,36). The MYO level of the Glycerol + HE group was higher than that of the Saline + HE group at 6 h, but the difference was not statistically significant. Preexisting muscle injury caused by glycerol may have exacerbated the follow-up muscle injury caused by heat exercise, and prolonged the rise time of CK in the Glycerol + HE group.
As a typical member of the IL-6 cytokine family, IL-6 is a pleiotropic pro-inflammatory cytokine, which is released by the skeletal muscle during exercise, and is also promoted by infection, stress, and injury (37). IL-6 promotes the production of multiple pro-inflammatory cytokines (including IL-6 itself) and chemokines through the activation of NF-κB and STAT3, thereby promoting systemic inflammation (38). The results showed that in the Glycerol Control group, glycerol damaged muscle tissue and promoted IL-6 production, and the IL-6 level was higher than that of the Saline Control group at 0 h. This further promoted the increase of IL-6 in the Glycerol + HE group during the subsequent heat exercise. The IL-6 levels of the Glycerol + HE group were higher than those of the Saline + HE group and Glycerol + SHE group at 6 and 12 h. The significantly increased IL-6 levels of the Glycerol + HE group suggested a more severe inflammatory response, which is also an important pathophysiological process of EHS. At the same time, as a pyrogen, the increased expression level of IL-6 may also promote the further increase of Tc in mice (39). The results also showed that the Tc at the endpoint of the Glycerol + HE group was 44.28 ℃ higher than that of the Saline + HE group. Additionally, the mice had more severe symptoms, such as severe convulsions at the end point. The higher Tc and more severe neurological symptoms of the Glycerol + HE group indicated that the heat damage of the mice was aggravated. The increased muscle injury, inflammatory response, and heat injury of the Glycerol + HE group may partly explain the occurrence of AKI in this model.
Our study had some limitations. First, the functional damage of other organs in mice was not evaluated, but some studies on EHS have confirmed damage to liver, intestine, and heart in mice (18,20,30). Second, this is a relatively mild EHS-AKI mouse model. Indeed, renal function had almost recovered at 48 h post-AKI. Thus, the model may not be able to be used to evaluate the therapeutic effects of drugs, and for therapeutic experiments, a more severe AKI model may be needed to observe the therapeutic effect of the relevant drugs.
In conclusion, in this study, we successfully constructed an EHS-AKI mouse model, in which the mice presented with increased Cr, BUN, and tubular necrosis. This model may be an effective tool for further studying the underlying mechanism of EHS-AKI and exploring potential therapeutic targets.
Acknowledgments
Funding: This study was funded by grants from the National Natural Science Foundation of China (grant No. 81871581 to Prof. Dr. Feihu Zhou).
Footnote
Reporting Checklist: The authors have completed the ARRIVE reporting checklist. Available at https://atm.amegroups.com/article/view/10.21037/atm-22-715/rc
Data Sharing Statement: Available at https://atm.amegroups.com/article/view/10.21037/atm-22-715/dss
Conflicts of Interest: All authors have completed the ICMJE uniform disclosure form (available at https://atm.amegroups.com/article/view/10.21037/atm-22-715/coif). The authors have no conflicts of interest to declare.
Ethical Statement: The authors are accountable for all aspects of the work in ensuring that questions related to the accuracy or integrity of any part of the work are appropriately investigated and resolved. All experimental protocols involving animals were approved by the Animal Care and Use Committee of the Chinese People’s Liberation Army General Hospital, in compliance with the National Institutes of Health Guide for the Care and Use of Laboratory Animals.
Open Access Statement: This is an Open Access article distributed in accordance with the Creative Commons Attribution-NonCommercial-NoDerivs 4.0 International License (CC BY-NC-ND 4.0), which permits the non-commercial replication and distribution of the article with the strict proviso that no changes or edits are made and the original work is properly cited (including links to both the formal publication through the relevant DOI and the license). See: https://creativecommons.org/licenses/by-nc-nd/4.0/.
References
- Hifumi T, Kondo Y, Shimizu K, et al. Heat stroke. J Intensive Care 2018;6:30. [Crossref] [PubMed]
- Peiris AN, Jaroudi S, Noor R. Heat Stroke. JAMA 2017;318:2503. [Crossref] [PubMed]
- Casa DJ, Stearns RL. Preventing Sudden Death in Sports & Physical Activity. Burlington, MA: Jones & Bartlett Publishers; 2017.
- Thongprayoon C, Qureshi F, Petnak T, et al. Impact of Acute Kidney Injury on Outcomes of Hospitalizations for Heat Stroke in the United States. Diseases 2020;8:28. [Crossref] [PubMed]
- Zhao JJ, Zhou JJ, Hu J, et al. Analysis of risk factors affecting prognosis of exertional heat stroke. Zhonghua Wei Zhong Bing Ji Jiu Yi Xue 2013;25:515-8. [PubMed]
- Satirapoj B, Kongthaworn S, Choovichian P, et al. Electrolyte disturbances and risk factors of acute kidney injury patients receiving dialysis in exertional heat stroke. BMC Nephrol 2016;17:55. [Crossref] [PubMed]
- Ward MD, King MA, Gabrial C, et al. Biochemical recovery from exertional heat stroke follows a 16-day time course. PLoS One 2020;15:e0229616. [Crossref] [PubMed]
- Johnson RJ, Sánchez-Lozada LG, Newman LS, et al. Climate Change and the Kidney. Ann Nutr Metab 2019;74:38-44. [Crossref] [PubMed]
- Wan Y, Sun SS, Fu HY, et al. Adjuvant rhubarb alleviates organs dysfunction and inhibits inflammation in heat stroke. Exp Ther Med 2018;16:1493-8. [Crossref] [PubMed]
- Chen F, Li H, Zhu G, et al. Sodium tanshinone IIA sulfonate improves inflammation, aortic endothelial cell apoptosis, disseminated intravascular coagulation and multiple organ damage in a rat heat stroke model. Mol Med Rep 2017;16:87-94. [Crossref] [PubMed]
- Thongprayoon C, Petnak T, Kanduri SR, et al. Impact of rhabdomyolysis on outcomes of hospitalizations for heat stroke in the United States. Hosp Pract (1995) 2020;48:276-81. [Crossref] [PubMed]
- Li Z, McKenna ZJ, Kuennen MR, et al. The Potential Role of Exercise-Induced Muscle Damage in Exertional Heat Stroke. Sports Med 2021;51:863-72. [Crossref] [PubMed]
- Bruchim Y, Segev G, Kelmer E, et al. Hospitalized dogs recovery from naturally occurring heatstroke; does serum heat shock protein 72 can provide prognostic biomarker? Cell Stress Chaperones 2016;21:123-30. [Crossref] [PubMed]
- Lin Y, Zhang Y. Renoprotective effect of oral rehydration solution III in exertional heatstroke rats. Ren Fail 2019;41:190-6. [Crossref] [PubMed]
- Garcia CK, Mattingly AJ, Robinson GP, et al. Sex-dependent responses to exertional heat stroke in mice. J Appl Physiol (1985) 2018;125:841-9. [Crossref] [PubMed]
- Ly JP, Onay T, Quaggin SE. Mouse models to study kidney development, function and disease. Curr Opin Nephrol Hypertens 2011;20:382-90. [Crossref] [PubMed]
- Rabe M, Schaefer F. Non-Transgenic Mouse Models of Kidney Disease. Nephron 2016;133:53-61. [Crossref] [PubMed]
- King MA, Leon LR, Mustico DL, et al. Biomarkers of multiorgan injury in a preclinical model of exertional heat stroke. J Appl Physiol (1985) 2015;118:1207-20. [Crossref] [PubMed]
- King MA, Leon LR, Morse DA, et al. Unique cytokine and chemokine responses to exertional heat stroke in mice. J Appl Physiol (1985) 2017;122:296-306. [Crossref] [PubMed]
- He SX, Li R, Yang HH, et al. Optimization of a Rhabdomyolysis Model in Mice With Exertional Heat Stroke Mouse Model of EHS-Rhabdomyolysis. Front Physiol 2020;11:642. [Crossref] [PubMed]
- Scott RP, Quaggin SE. Review series: The cell biology of renal filtration. J Cell Biol 2015;209:199-210. [Crossref] [PubMed]
- Susztak K, Bitzer M, Meyer TW, et al. Animal models of renal disease. Kidney Int 2008;73:526-8. [Crossref] [PubMed]
- Song SJ, Kim SM, Lee SH, et al. Rhabdomyolysis-Induced AKI Was Ameliorated in NLRP3 KO Mice via Alleviation of Mitochondrial Lipid Peroxidation in Renal Tubular Cells. Int J Mol Sci 2020;21:8564. [Crossref] [PubMed]
- Liu J, Cui X, Guo F, et al. 2-methylquinazoline derivative F7 as a potent and selective HDAC6 inhibitor protected against rhabdomyolysis-induced acute kidney injury. PLoS One 2019;14:e0224158. [Crossref] [PubMed]
- Fortes MB, Di Felice U, Dolci A, et al. Muscle-damaging exercise increases heat strain during subsequent exercise heat stress. Med Sci Sports Exerc 2013;45:1915-24. [Crossref] [PubMed]
- Montain SJ, Latzka WA, Sawka MN. Impact of muscle injury and accompanying inflammatory response on thermoregulation during exercise in the heat. J Appl Physiol (1985) 2000;89:1123-30. [Crossref] [PubMed]
- Tseng WC, Lee PY, Tsai MT, et al. Hypoxic mesenchymal stem cells ameliorate acute kidney ischemia-reperfusion injury via enhancing renal tubular autophagy. Stem Cell Res Ther 2021;12:367. [Crossref] [PubMed]
- Ricci Z, Romagnoli S. Acute Kidney Injury: Diagnosis and Classification in Adults and Children. Contrib Nephrol 2018;193:1-12. [Crossref] [PubMed]
- Garcia CK, Sheikh LH, Iwaniec JD, et al. Effects of Ibuprofen during Exertional Heat Stroke in Mice. Med Sci Sports Exerc 2020;52:1870-8. [Crossref] [PubMed]
- Laitano O, Garcia CK, Mattingly AJ, et al. Delayed metabolic dysfunction in myocardium following exertional heat stroke in mice. J Physiol 2020;598:967-85. [Crossref] [PubMed]
- Bugyei-Twum A. Sex differences in long-term effects of exertional heat stroke on myocardial metabolism. J Physiol 2020;598:3829-30. [Crossref] [PubMed]
- Li D, Wang X, Liu B, et al. Exercises in hot and humid environment caused liver injury in a rat model. PLoS One 2014;9:e111741. [Crossref] [PubMed]
- Bao YW, Yuan Y, Chen JH, et al. Kidney disease models: tools to identify mechanisms and potential therapeutic targets. Zool Res 2018;39:72-86. [PubMed]
- Lippi G, Schena F, Ceriotti F. Diagnostic biomarkers of muscle injury and exertional rhabdomyolysis. Clin Chem Lab Med 2018;57:175-82. [Crossref] [PubMed]
- Bäcker HC, Busko M, Krause FG, et al. Exertional rhabdomyolysis and causes of elevation of creatine kinase. Phys Sportsmed 2020;48:179-85. [Crossref] [PubMed]
- Panizo N, Rubio-Navarro A, Amaro-Villalobos JM, et al. Molecular Mechanisms and Novel Therapeutic Approaches to Rhabdomyolysis-Induced Acute Kidney Injury. Kidney Blood Press Res 2015;40:520-32. [Crossref] [PubMed]
- Hennigar SR, McClung JP, Pasiakos SM. Nutritional interventions and the IL-6 response to exercise. FASEB J 2017;31:3719-28. [Crossref] [PubMed]
- Hirano T. IL-6 in inflammation, autoimmunity and cancer. Int Immunol 2021;33:127-48. [Crossref] [PubMed]
- Mosili P, Maikoo S, Mabandla MV, et al. The Pathogenesis of Fever-Induced Febrile Seizures and Its Current State. Neurosci Insights 2020;15:2633105520956973. [Crossref] [PubMed]
(English Language Editor: L. Huleatt)