This article has an erratum available at: http://dx.doi.org/10.21037/atm-2022-13 the article has been update on 2022-05-17 at here.
The downregulation of miR-129-5p relieves the inflammatory response in acute respiratory distress syndrome by regulating PPARγ-mediated autophagy
Introduction
Acute respiratory distress syndrome (ARDS) is mainly manifested by the continuous activation of the inflammatory system. ARDS is a life-threatening pulmonary inflammatory reactive disease (1,2). Its clinical manifestations include respiratory distress, refractory hypoxemia, and decreased lung compliance. Epidemiological data indicate that the lives of 2.2 million patients are threatened by ARDS each year worldwide (3,4). As many as 40,070 patients die each year from ARDS; thus, ARDS remains an important public health issue (3,4). Acute lung injury (ALI) is a mild form of ARDS with a complex pathogenesis. Presently, an insufficient understanding of the pathophysiology of ALI affects the evaluation and treatment of ARDS.
The continuous activation of the inflammatory system has a close causal relationship with the progression and outcome of ARDS, and cell autophagy has been confirmed to be closely related to the inflammatory response (5). For example, when Toll-like receptor (TLR)-related pathways induce inflammatory responses, they can simultaneously trigger cellular autophagy, and cellular autophagy has a significant negative regulatory effect on TLR signals and inflammatory responses (6,7). Meng (8) showed that enhancing autophagy reduces inflammation and oxidative stress, and protects lung injury. Peroxisome proliferator-activated receptors (PPARs) are transcription factors. PPARs are mainly expressed in adipose tissue, but are also highly expressed in the kidneys and lungs. The PPARγ is one of the pathways that regulates autophagy and inflammation. In aged human dental pulp cells, the activation of PPARs maintains cell homeostasis by inducing antioxidant responses and autophagy, and inhibits the expression of inflammatory factors (9). Research has shown that the PPARγ activates autophagy (10-12). Further, the activation of the PPARγ has been shown to inhibit the inflammatory response against lung injury (13). In a lipopolysaccharide (LPS)-induced ALI model, PPARγ activation is related to the inhibition of the inflammatory response (14). However, it is still unclear whether the mechanism by which the PPARγ alleviates ALI is related to autophagy.
MicroRNA (miRNA) regulates gene expression at the post-transcriptional level. Studies have shown that the level of the PPARγ is regulated by miRNA, and participates in the process of inflammatory response and autophagy (15,16). Additionally, the intervention of miRNA may regulate autophagy flux by regulating the PPARγ expression (17,18). However, in ALI, the upstream miRNA that regulates the PPARγ is still unclear. MiRNA-129-5p (miR-129-5p) is a newly discovered miRNA that regulates autophagy and inflammation, for example, it is showed that miR-129-5p can alleviate LPS-induced acute kidney injury (19). Further, it has been shown that miR-129-5p participates in adipogenesis by regulating autophagy (20). However, the role of miR-129-5p in ARDS remains unclear. This study sought to examine the role of the PPARγ in the regulation of autophagy in ARDS, and identify the key upstream regulatory miRNA, and its role in autophagy. We present the following article in accordance with the ARRIVE reporting checklist (available at https://atm.amegroups.com/article/view/10.21037/atm-22-979/rc).
Methods
Animals
In this study, 20 C57BL/6 mice [specific-pathogen free (SPF), male, 18–20 g, 8–10 weeks old, Medical Animal Experimental Center of the Third Military Medical University] were randomly divided into the following four groups: (I) the sham group; (II) the ARDS group; (III) the ARDS + pioglitazone group; and (IV) the ARDS + GW9662 group (n=5). The animals were kept in the same environment (SPF Animal Laboratory) to eliminate interference.
LPS (10 mg) was dissolved in 5 mL of saline and stored at 4 ℃. The mice were intraperitoneally injected with LPS (15 mg/kg) to establish an ARDS model. The mice in the ARDS + pioglitazone group also received pioglitazone (10 mg/kg, agonists of PPARγ, Selleck, China) for 4 consecutive days before LPS. At 6 h before treatment with LPS, the mice in the ARDS + GW9662 group were injected intraperitoneally with GW9662 [1 mg/kg, 10% (v/v), dissolved in DMSO, antagonists of PPARγ, Selleck, China]. The mice were sacrificed by cervical dislocation after 1% pentobarbital (50 mg/kg) anesthesia at 24 h after LPS injection, and blood and lung tissues were collected for the experiments. The animal research was approved by the Animal Experiment Committee of The First Affiliated Hospital of the Army Medical University (AMUWEC20182012). The experiment process complied with the institutional guidelines for the care and use of laboratory animals. Subsequent indicator testers did not know the specific groupings of the samples.
Wet-to-dry ratio of the lung
At 24 h after treatment with LPS, the right lungs were removed and the wet weight was determined. The lungs were incubated at 60 ℃ for 5 days to dry, and the weight was measured. The wet-to-dry weight ratio was then calculated.
Hematoxylin and eosin (H&E)
The lung tissue was fixed with 4% (v/v) paraformaldehyde and embedded in paraffin, sectioned at 4 µm thickness, and stained with H&E solution (Sigma-Aldrich, St. Louis, MO, USA).
Enzyme-linked immunosorbent assay (ELISA)
Tumor necrosis factor-α (TNF-α), interleukin (IL)-1β, and IL-6 in cell cultures were detected using an ELISA kit (Beyotime, Beijing, China). The antibody and reagent were added according to the manufacturer’s instructions. The optical density (OD) at 450 nm was measured by a microplate reader.
Cell cultures and transfections
Human lung epithelial cells BEAS-2B (ATCC, USA) were cultured in bronchial epithelial cell culture medium BEpiCM (#3221, SclenCell, USA). The culture environment was 37 ℃ and 5% carbon dioxide (CO2). Next, 0, 0.5, 1, or 2 µg/mL of LPS (Sigma-Aldrich) was added to the culture medium, and the cells were cultured for 24 h. PBS (Sigma-Aldrich) was added to the 0 µg/mL group as a control.
The miR-129-5p and PPARγ in the cells were downregulated by plasmid transfection. In brief, 2 µL of LipofectamineTM 3000 (Invitrogen, Waltham, USA), 40 pmol of miR-129-5p inhibitor, short interfering RNA PPARγ (si-PPARγ, F-5'-CTCCAATGTTCTCAAACTTAC-3'; R-5'-GATGAGTCATGTAAGTTGACC-3') or negative control (NC) (GenePharma, Suzhou, China) were mixed in 50 µL serum-free medium at room temperature for 15 min. The lipid compounds were diluted in 300 µL of serum-free medium, and incubated with the cells at 37 ℃ with 5% CO2 for subsequent experiments.
Dual-luciferase reporter assay
The wild-type (WT)/mutated (MUT) PPARγ and miR-129-5p mimic/NC were both cloned into pMIR-REPORT Luciferase vectors (GenePharma, Shanghai, China). The BEAS-2B cells were transfected with both vectors using Lipofectamine 3000 for 24 h. The Dual-Luciferase Reporter 1000 Assay System (Promega, Madison, WI, USA) was used to evaluate luciferase activity.
Quantitative polymerase chain reaction (qPCR)
The total RNA was acquired, and the purity was detected. The RNA was reverse transcribed using a reverse transcription complementary DNA (cDNA) kit (TaKaRa, Dalian, China) to synthesize the cDNA. SYBR Green PCR Master Mix (TaKaRa, Dalian, China) and the PCR detection system (ABI 7500, Life Technology, Carlsbad, CA, USA) were applied to conduct the qPCR experiment. The PCR cycle was as follows: pretreatment at 95 ℃ for 10 min; followed by 40 cycles of 94 ℃ for 15 s, 60 ℃ for 1 min, and 4 ℃ for preservation. The comparative cycle threshold (ΔΔCt) was employed to analyze the expression of the RNAs. GAPDH and U6 expression was used for normalization. The primers are listed in Table 1.
Table 1
Gene | Primer sequence |
---|---|
MiR-129-5p-F | 5'-GGGGGCTTTTTGCGGTCTGG-3' |
MiR-129-5p-R | 5'-AGTGCGTGTCGTGGAGTC-3' |
U6-F | 5'-CTCGCTTCGGCAGCACATATACT-3' |
U6-R | 5'-ACGCTTCACGAATTTGCGTGTC-3' |
PPARγ-R | 5'-CGAAGAGCACCTGACGAACA-3' |
PPARγ-F | 5'-CTCGTCATTCCCAAAACGCC-3' |
GAPDH-F | 5'-GCATCTTCTTGTGCAGTGCC-3' |
GAPDH-R | 5'-ACTGTGCCGTTGAATTTGCC-3' |
Western blot
The protein was extracted by protein lysate and detected using a BCA kit. Next, the protein was separated using SDS-PAGE (110 V, 100 min). The proteins were transferred to PVDF membranes (90 V, 90 min). The PVDF membranes were blocked in 5% non-fat milk for 1 h at room temperature. The anti-PPARγ (Abcam, San Francisco, CA, USA; ab45036, 57 kD), anti-Beclin1 (ab207612, 52 kD), LC3B (ab51520, 16/18 kD), and GAPDH antibody (ab8245, 36 kD) diluted at 1:500 were added and incubated overnight at 4 ℃. Next, the secondary antibodies (sc-516102/sc-2357; Santa Cruz Biotechnology, Inc., Dallas, TX, USA) were diluted at 1:5,000, and added and incubated at room temperature for 2 h. Protein blot bands were detected by the PierceTM ECL plus western blotting substrate (Thermo Fisher Scientific) in ChemiDoc MP (Bio-Rad, Hercules, CA, USA).
Cell counting kit-8 (CCK-8) assays
In this study, 2×104 cells (100 µL) were seeded in96-well plates, 10 µL of CCK-8 (Beyotime Institute of Biotechnology, Beijing, China) medium was added, and the cells were cultured at 37 ℃ for 2 h. The OD at 450 nm was measured using a microplate reader (Tecan Infinite M200 Micro Plate Reader; LabX, Männedorf, Switzerland).
Flow cytometry
The apoptosis rates were tested using flow cytometry (BD FACSCalibur, Becton Dickinson, USA) and the annexin V-FITC/PI kit (Millipore, USA). The reagents were added according to the manufacturers’ instructions. Q2 + Q3 was the apoptotic rate.
Statistical analysis
The experiments were repeated 3 times. All the statistical analyses were performed using GraphPad Prism 7. All the experimental data are presented as the mean ± standard deviation. The t-test was used for comparisons between groups, and the Tukey test was used for comparisons among multiple groups. A P value <0.05 was considered statistically significant.
Results
PPARγ alleviates lung injury in LPS-induced ARDS mice by promoting autophagy and suppressing inflammatory
To analyze whether the mechanism of the PPARγ alleviating the inflammatory response of ALI is related to autophagy, we simulated the ARDS process in vivo by injecting LPS intraperitoneally into a mouse model of ALI. GW9662 and pioglitazone were used to inhibit and activate the PPARγ, respectively. By observing the pathological changes of lung tissue, we found that antagonizing the PPARγ with GW9662 further aggravated lung tissue damage, while activating the PPARγ with pioglitazone alleviated LPS-induced lung injury to a certain extent (see Figure 1A). GW9662 further increased of TNF-α, IL-1β, and IL-6, and the wet-to-dry ratio caused by LPS. The activation of the PPARγ by pioglitazone reduced the inflammatory reaction and pulmonary edema caused by LPS (see Figure 1B-1E). Additionally, the GW9662 antagonism of the PPARγ caused the levels of autophagy-related proteins Beclin1 and LC3II/LC3I to reduce further. Pioglitazone not only upregulated the PPARγ, but also partially reversed the autophagy inhibition caused by LPS (see Figure 1F). This suggested that in the LPS-induced ARDS model, the level of the PPARγ and autophagy was reduced. Antagonizing the PPARγ with GW9662 further inhibited autophagy and exacerbated pulmonary edema and inflammatory damage. Conversely, the use of pioglitazone promoted autophagy levels and effectively relieved the lung injury caused by LPS. This suggested that the anti-inflammatory effects of the PPARγ might be related to autophagy.
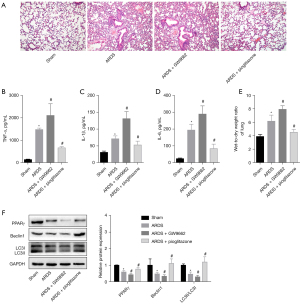
MiR-129-5p directly targets PPARγ
The prediction results showed that there were targeted binding sites between miR-129-5p and the 3'-untranslated region (3'-UTR) of the PPARγ messenger RNA (mRNA) (see Figure 2A). The dual-luciferase report verified that miR-129-5p directly targeted the PPARγ mRNA 3'-UTR (see Figure 2B,2C).
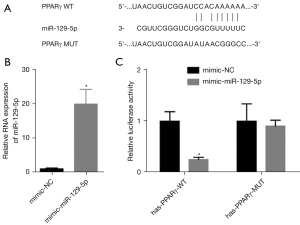
LPS induces miR-129-5p and inhibits PPARγ
The BEAS-2B cells and LPS were used to establish an in vitro ALI model to simulate ARDS. The results showed that LPS inhibited the growth and promoted the apoptosis of the BEAS-2B cells in a dose-dependent manner in the concentration range of 0–2 µg/mL (see Figure 3A,3B). The results also showed that LPS promoted the levels of miR-129-5p, such that the levels of miR-129-5p increased 2-, 5-, and 6-fold at 0.5, 1 and 2 µg/mL of LPS, respectively (see Figure 3C). Additionally, in a concentration range of 0–2 µg/mL, LPS promoted the secretion of TNF-α, IL-1β, and IL-6 by BEAS-2B cells in a dose-dependent manner (see Figure 3D-3F).
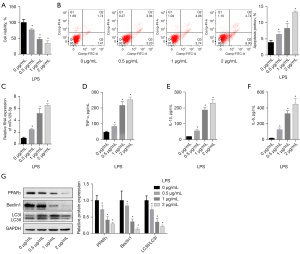
To analyze the changes of the PPARγ-related pathways and autophagy levels in ARDS simulated by LPS, the PPARγ and the autophagy-related proteins Beclin1 and LC3 were detected. We found that the levels of the PPARγ protein decreased to about 0.7, 0.4, and 0.3 for 0, 0.5, 1, and 2 µg/mL of LPS, respectively. The changing trend of autophagy-related proteins were also reflected in the range of 0–2 µg/mL, such that LPS inhibited autophagy in a quantitative manner (see Figure 3G). Thus, in the LPS-induced in vitro ARDS model, the miR-129-5p and inflammatory factor levels were elevated, while the PPARγ and autophagy fluxes were decreased.
PPARγ-induced autophagy alleviates the inflammatory response and apoptosis of lung epithelial cells caused by LPS
The BEAS-2B cells were cultured at 1 µg/mL of LPS to induce acute injury, and simulate the ARDS environment in vitro. Before adding LPS, the cells were transfected with the miR-129-5p inhibitor and/or the si-PPARγ plasmid. We found that LPS induced the upregulation of miR-129-5p and the downregulation of the PPARγ mRNA and PPARγ protein. The addition of the miR-129-5p inhibitor promoted the expression of the PPARγ mRNA and PPARγ. Additionally, si-PPARγ blocked the promotion of the miR-129-5p inhibitor on the PPAR and PPARγ. The PPARγ mRNA and PPARγ had no significant effects on the level of miR-129-5p (see Figure 4A-4C). In the presence of 1 µg/mL of LPS, downregulating the expression of miR-129-5p promoted cell survival and reduced the apoptosis rate. Additionally, the downregulation of the PPARγ further increased the damage of LPS to cells, and the co-transfection of the si-PPARγ blocked the protective effect of the miR-129-5p inhibitor on the cells (see Figure 4D,4E). In the presence of 1 µg/mL of LPS, the transfection of the miR-129-5p inhibitor restored the levels of Beclin1 protein and LC3II/LC3I to promote autophagy, and inhibited the cells from secreting TNF-α, IL-1β, and IL-6 inflammatory factors into the culture medium. Inhibiting the expression of the PPARγ protein further reduced the levels of Beclin1 and LC3II/LC3I to inhibit autophagy, and blocked the promotion effect of the miR-129-5p inhibitor on autophagy and inhibition of inflammatory factors (see Figure 4F-4I). This suggested that the transfection of the miR-129-5p inhibitor increased the level of the PPARγ protein, promoted autophagy, inhibited the secretion of inflammatory factors, and alleviated the apoptosis induced by LPS. Additionally, this effect was blocked by the si-PPARγ plasmid.
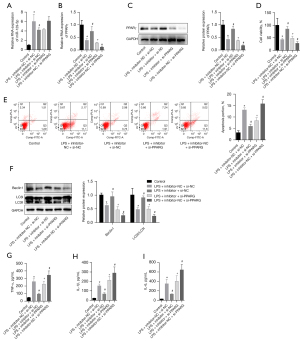
Discussion
In this study, we found that in ARDS, miR-129-5p is upregulated and autophagy is suppressed. In in vitro and in vivo ARDS models, the downregulation of miR-129-5p may promote PPARγ expression and play a role in the promotion of autophagy. This may cause the promotion of autophagy and the inhibition of inflammatory response, thus preventing lung injury.
Autophagy is the degradation of proteins and organelles mediated by lysosomes in eukaryotes. It is a physiological process in which the material is recycled and maintained in the internal environment homeostasis (21). Autophagy not only occurs during the normal physiological metabolism of cells, but is also a defense mechanism that enables cells to cope with adverse external environmental factors (22). The disturbance of cell autophagy or autophagy-related genes is a key factor causing the inflammatory response. Research has shown that in a mouse model of ALI induced by LPS, autophagy inhibits the inflammatory response and relieves lung injury by regulating the NF-κB pathway (23). In an LPS-induced ARDS mice model, Parkin-dependent mitophagy autophagy was shown to inhibit apoptosis and relieve lung injury (24). However, the mechanism by which ARDS-related autophagy is regulated is unknown.
The PPARs are activated after binding to their ligands, and they then interact with PPAR response elements upstream of the target gene promoter, ultimately regulating the transcription of the target gene and the metabolic process in the cell. In a LPS-induced acute inflammatory injury model of RAW264.7 cells in vitro, the expression levels of TNF-α and other inflammatory factors were upregulated, and the level of the PPARγ protein was inhibited. The activation of the PPARγ inhibits the inflammatory response by inhibiting the activation of NF-κBp65 (25). Decreasing the methylation level of the PPARγ gene promoter inhibits the expression level of inflammatory genes (26). The PPARγ is also an important protein that regulates the autophagy pathway (27,28). Based on this, we speculated that the PPARγ may inhibit the inflammatory response by promoting autophagy, and alleviate the lung injury caused by ARDS. LPS-induced ALI was used to simulate an ARDS mice model, and pioglitazone and GW9662 were applied to activate and antagonize the PPARγ, respectively. The results showed that the activation of the PPARγ significantly relieved lung injury and pulmonary edema, inhibited the expression of inflammatory factors, and promoted autophagy. GW9662 aggravated inflammatory damage and inhibited autophagy. Research has shown that pioglitazone relieves ARDS (29). Defects in autophagy activate inflammasomes, and promote the release of cytokines, such as IL-1 and IL-18 (30). Thus, the anti-inflammatory effects of the PPARγ may be related to autophagy.
MiRNA-related targeted regulatory mechanisms have recently been found to be related to ARDS (31,32). MiRNAs regulate the inflammatory response by regulating the target gene expression, and participate in ARDS and lung injury (31,32). MiR-129-5p promotes the inflammatory response and regulates autophagy. During the inflammatory response of cardiomyocytes, the miR-129-5p level is significantly upregulated and involved in promoting the apoptosis of cardiomyocytes (33). In adipose-derived stem cells, inhibiting the level of miR-129-5p has also been shown to regulate the immunosuppressive response and reduce the release of inflammatory factors (34). However, miR-129-5p has been shown to have anti-inflammatory effects (35,36). Recent research has shown that miR-129-5p has anti-autophagy effects (37-39). During hydrogen peroxide, infection, and tumor-related autophagy, the increased expression of miR-129-5p is related to the inhibition of autophagy (37-39). Through in vitro experiments, this study showed that the targeting of miR-129-5p inhibits the expression of the PPARγ. Further, downregulating the level of miR-129-5p promotes the PPARγ expression, promotes autophagy, and inhibits inflammatory response and apoptosis. Additionally, silencing the PPARγ blocks the protective effects of miR-129-5p. This indicates that in the LPS-induced ARDS model, the decrease of the PPARγ caused by miR-129-5p may promote the inflammatory response, and inhibit autophagy, thereby leading to the inflammatory response.
In conclusion, activating the PPARγ might promote autophagy, inhibit the inflammatory response, and alleviate lung injury induced by LPS. The PPARγ is under the targeted regulation of miR-129-5p. MiR-129-5p may induce autophagy and inhibit the inflammatory response by promoting the expression of the PPARγ. The mechanism by which miR-129-5p regulates autophagy, and its role in ARDS still need to be confirmed. Additionally, the clinical significance of miR-129-5p in ARDS and its relationship with prognosis require further exploration. The mechanism by which miR-129-5p regulates autophagy and its role in ARDS still needs to be confirmed by in vivo studies.
Acknowledgments
Funding: This study received funding from the General project of National Natural Science Foundation of China (project No. 81670070), and the Chongqing Research Program of Basic Research and Frontier Technology (project No. cstc2017jcyjAX0338).
Footnote
Reporting Checklist: The authors have completed the ARRIVE reporting checklist. Available at https://atm.amegroups.com/article/view/10.21037/atm-22-979/rc
Data Sharing Statement: Available at https://atm.amegroups.com/article/view/10.21037/atm-22-979/dss
Conflicts of Interest: All authors have completed the ICMJE uniform disclosure form (available at https://atm.amegroups.com/article/view/10.21037/atm-22-979/coif). All authors report that this study received funding from the General project of National Natural Science Foundation of China (project No. 81670070), and the Chongqing Research Program of Basic Research and Frontier Technology (project No. cstc2017jcyjAX0338). The authors have no other conflicts of interest to declare.
Ethical Statement: The authors are accountable for all aspects of the work in ensuring that questions related to the accuracy or integrity of any part of the work are appropriately investigated and resolved. The animal research was approved by the Animal Experiment Committee of The First Affiliated Hospital of the Army Medical University (AMUWEC20182012). The experiment process complied with the institutional guidelines for the care and use of laboratory animals.
Open Access Statement: This is an Open Access article distributed in accordance with the Creative Commons Attribution-NonCommercial-NoDerivs 4.0 International License (CC BY-NC-ND 4.0), which permits the non-commercial replication and distribution of the article with the strict proviso that no changes or edits are made and the original work is properly cited (including links to both the formal publication through the relevant DOI and the license). See: https://creativecommons.org/licenses/by-nc-nd/4.0/.
References
- Kato K, Fujimoto S, Inukai S, et al. Late-onset acute respiratory distress syndrome induced by a gadolinium-based contrast agent. Respir Med Case Rep 2020;29:100990. [Crossref] [PubMed]
- Laffey JG, Misak C, Kavanagh BP. Acute respiratory distress syndrome. BMJ 2017;359:j5055. [Crossref] [PubMed]
- Monahan LJ. Acute respiratory distress syndrome. Curr Probl Pediatr Adolesc Health Care 2013;43:278-84. [Crossref] [PubMed]
- Muthu V, Dhooria S, Aggarwal AN, et al. Acute Respiratory Distress Syndrome Due To Tuberculosis in a Respiratory ICU Over a 16-Year Period. Crit Care Med 2017;45:e1087-90. [Crossref] [PubMed]
- Netea-Maier RT, Plantinga TS, van de Veerdonk FL, et al. Modulation of inflammation by autophagy: Consequences for human disease. Autophagy 2016;12:245-60. [Crossref] [PubMed]
- Into T, Inomata M, Takayama E, et al. Autophagy in regulation of Toll-like receptor signaling. Cell Signal 2012;24:1150-62. [Crossref] [PubMed]
- Inomata M, Niida S, Shibata K, et al. Regulation of Toll-like receptor signaling by NDP52-mediated selective autophagy is normally inactivated by A20. Cell Mol Life Sci 2012;69:963-79. [Crossref] [PubMed]
- Meng L, Zhao X, Zhang H. HIPK1 Interference Attenuates Inflammation and Oxidative Stress of Acute Lung Injury via Autophagy. Med Sci Monit 2019;25:827-35. [Crossref] [PubMed]
- Lee YH, Lee HY, Kim TG, et al. PPARγ Maintains Homeostasis through Autophagy Regulation in Dental Pulp. J Dent Res 2015;94:729-37. [Crossref] [PubMed]
- Ji J, Xue TF, Guo XD, et al. Antagonizing peroxisome proliferator-activated receptor γ facilitates M1-to-M2 shift of microglia by enhancing autophagy via the LKB1-AMPK signaling pathway. Aging Cell 2018;17:e12774. [Crossref] [PubMed]
- Jiang C, Liu S, Cao Y, et al. High Glucose Induces Autophagy through PPARγ-Dependent Pathway in Human Nucleus Pulposus Cells. PPAR Res 2018;2018:8512745. [Crossref] [PubMed]
- Liu J, Yao Q, Xiao L, et al. PPARγ induces NEDD4 gene expression to promote autophagy and insulin action. FEBS J 2020;287:529-45. [Crossref] [PubMed]
- Wang YL, Guo XY, He W, et al. Effects of alliin on LPS-induced acute lung injury by activating PPARγ. Microb Pathog 2017;110:375-9. [Crossref] [PubMed]
- Hu X, Qin H, Li Y, et al. Biochanin A protect against lipopolysaccharide-induced acute lung injury in mice by regulating TLR4/NF-κB and PPAR-γ pathway. Microb Pathog 2020;138:103846. [Crossref] [PubMed]
- Wang D, Shi L, Xin W, et al. Activation of PPARγ inhibits pro-inflammatory cytokines production by upregulation of miR-124 in vitro and in vivo. Biochem Biophys Res Commun 2017;486:726-31. [Crossref] [PubMed]
- Qi H, Ren J, Ba L, et al. MSTN Attenuates Cardiac Hypertrophy through Inhibition of Excessive Cardiac Autophagy by Blocking AMPK /mTOR and miR-128/PPARγ/NF-κB. Mol Ther Nucleic Acids 2020;19:507-22. [Crossref] [PubMed]
- Bae IS, Park PJ, Lee JH, et al. PPARγ-mediated G-protein coupled receptor 120 signaling pathway promotes transcriptional activation of miR-143 in adipocytes. Gene 2017;626:64-9. [Crossref] [PubMed]
- Yu Y, Du H, Wei S, et al. Adipocyte-Derived Exosomal MiR-27a Induces Insulin Resistance in Skeletal Muscle Through Repression of PPARγ. Theranostics 2018;8:2171-88. [Crossref] [PubMed]
- Huang X, Hou X, Chuan L, et al. miR-129-5p alleviates LPS-induced acute kidney injury via targeting HMGB1/TLRs/NF-kappaB pathway. Int Immunopharmacol 2020;89:107016. [Crossref] [PubMed]
- Fu X, Jin L, Han L, et al. miR-129-5p Inhibits Adipogenesis through Autophagy and May Be a Potential Biomarker for Obesity. Int J Endocrinol 2019;2019:5069578. [Crossref] [PubMed]
- Ryter SW, Choi AM. Autophagy in lung disease pathogenesis and therapeutics. Redox Biol 2015;4:215-25. [Crossref] [PubMed]
- Liao G, Gao B, Gao Y, et al. Phycocyanin Inhibits Tumorigenic Potential of Pancreatic Cancer Cells: Role of Apoptosis and Autophagy. Sci Rep 2016;6:34564. [Crossref] [PubMed]
- Lei M, Wang CJ, Yu F, et al. Different intensity of autophagy regulate interleukin-33 to control the uncontrolled inflammation of acute lung injury. Inflamm Res 2019;68:665-75. [Crossref] [PubMed]
- Li T, Liu Y, Xu W, et al. Polydatin mediates Parkin-dependent mitophagy and protects against mitochondria-dependent apoptosis in acute respiratory distress syndrome. Lab Invest 2019;99:819-29. [Crossref] [PubMed]
- Zhu T, Zhang W, Feng SJ, et al. Emodin suppresses LPS-induced inflammation in RAW264.7 cells through a PPARγ-dependent pathway. Int Immunopharmacol 2016;34:16-24. [Crossref] [PubMed]
- Lei C, Jiao Y, He B, et al. RIP140 down-regulation alleviates acute lung injury via the inhibition of LPS-induced PPARγ promoter methylation. Pulm Pharmacol Ther 2016;37:57-64. [Crossref] [PubMed]
- Faghfouri AH, Khajebishak Y, Payahoo L, et al. PPAR-gamma agonists: Potential modulators of autophagy in obesity. Eur J Pharmacol 2021;912:174562. [Crossref] [PubMed]
- Xi X, Zou C, Ye Z, et al. Pioglitazone protects tubular cells against hypoxia/reoxygenation injury through enhancing autophagy via AMPK-mTOR signaling pathway. Eur J Pharmacol 2019;863:172695. [Crossref] [PubMed]
- He J, Qi D, Tang XM, et al. Rosiglitazone promotes ENaC-mediated alveolar fluid clearance in acute lung injury through the PPARγ/SGK1 signaling pathway. Cell Mol Biol Lett 2019;24:35. [Crossref] [PubMed]
- Brickle A, Tran HT, Lim R, et al. Autophagy, which is decreased in labouring fetal membranes, regulates IL-1β production via the inflammasome. Placenta 2015;36:1393-404. [Crossref] [PubMed]
- Jiang J, Song Z, Zhang L. miR-155-5p Promotes Progression of Acute Respiratory Distress Syndrome by Inhibiting Differentiation of Bone Marrow Mesenchymal Stem Cells to Alveolar Type II Epithelial Cells. Med Sci Monit 2018;24:4330-8. [Crossref] [PubMed]
- Jiang X, Yu M, Zhu T, et al. Kcnq1ot1/miR-381-3p/ETS2 Axis Regulates Inflammation in Mouse Models of Acute Respiratory Distress Syndrome. Mol Ther Nucleic Acids 2020;19:179-89. [Crossref] [PubMed]
- Zou Y, Kong M. Tetrahydroxy stilbene glucoside alleviates palmitic acid-induced inflammation and apoptosis in cardiomyocytes by regulating miR-129-3p/Smad3 signaling. Cell Mol Biol Lett 2019;24:5. [Crossref] [PubMed]
- Zhang HY, Wang YH, Wang Y, et al. miR-129-5p Regulates the Immunomodulatory Functions of Adipose-Derived Stem Cells via Targeting Stat1 Signaling. Stem Cells Int 2019;2019:2631024. [Crossref] [PubMed]
- Zeng Z, Liu Y, Zheng W, et al. MicroRNA-129-5p alleviates nerve injury and inflammatory response of Alzheimer's disease via downregulating SOX6. Cell Cycle 2019;18:3095-110. [Crossref] [PubMed]
- Tian J, Song T, Wang W, et al. miR-129-5p Alleviates Neuropathic Pain Through Regulating HMGB1 Expression in CCI Rat Models. J Mol Neurosci 2020;70:84-93. [Crossref] [PubMed]
- Zhang H, Zhang X, Zhang J. MiR-129-5p inhibits autophagy and apoptosis of H9c2 cells induced by hydrogen peroxide via the PI3K/AKT/mTOR signaling pathway by targeting ATG14. Biochem Biophys Res Commun 2018;506:272-7. [Crossref] [PubMed]
- Qu Y, Ding S, Ma Z, et al. MiR-129-3p favors intracellular BCG survival in RAW264.7 cells by inhibiting autophagy via Atg4b. Cell Immunol 2019;337:22-32. [Crossref] [PubMed]
- Shi Y, Gong W, Lu L, et al. Upregulation of miR-129-5p increases the sensitivity to Taxol through inhibiting HMGB1-mediated cell autophagy in breast cancer MCF-7 cells. Braz J Med Biol Res 2019;52:e8657. [Crossref] [PubMed]
(English Language Editor: L. Huleatt)