The gut microbiome contributes to splenomegaly and tissue inflammation in a murine model of primary biliary cholangitis
Introduction
Splenomegaly is an abnormal enlargement of the spleen, frequently observed in sepsis, liver cirrhosis, myelofibrosis, and lymphoma (1-5). Splenomegaly is not a mere consequence of several conditions, but may also contribute to the clinical scenario, as represented by the left upper abdominal quadrant derived from the exerted pressure, anemia, and thrombocytopenia related to hypersplenism (6,7), high risk of bleeding from portal hypertension (8), and the possibility of spleen rupture (9). The pathophysiological basis of splenomegaly can be categorized into 3 main groups: increased splenic function, infiltration, and congestion. Immune-mediated disorders, such as rheumatoid arthritis (10) and primary biliary cholangitis (PBC) (11), can lead to hypertrophy of the spleen and then develop to splenomegaly. To date, there is no specific treatment for splenomegaly.
Recently, increasing evidence has suggested that gut microbiota plays a critical role in initiating host inflammatory response, particularly liver inflammation, which is due to the close crosstalk of the gut-liver axis (12). In pathological conditions, the increase of intestinal permeability leads to bacterial translocation and triggers chronic hepatic inflammation and fibrosis. The gut microbiome interacts with the host immune system (13) and also with the spleen, the largest secondary lymphoid organ (14,15), as the microbiome changes following splenectomy (16,17), which in turn ameliorates dysbiosis associated with liver cirrhosis (18). Further, the neonatal gut microbiota is central to spleen development (19), and germ-free mice have defective spleen myeloid cells (20). The gut microbiota also modulates systemic and splenic inflammatory cells induced by sleep deprivation and lipopolysaccharide (LPS) administration (21,22). The spleen-gut axis is further supported by the disappearance of murine intestinal immunoglobulin A (IgA)-secreting plasma cells after splenectomy (23), but additional evidence is needed to better define this interaction.
We have previously reported that p40−/−IL-2Rα−/− mice develop spontaneous autoimmune cholangitis and hepatic fibrosis, which resemble human PBC (24). Furthermore, based on our previous observation that p40−/−IL-2Rα−/− mice develop splenomegaly with enhanced liver T cell-mediated inflammation at 12 weeks of age, we investigated the significant effects of splenomegaly on liver inflammation and gut microbiome that are modulated by antibiotic treatment, ultimately suggesting that the gut microbiota contributes to splenomegaly. We present the following article in accordance with the ARRIVE reporting checklist (available at https://atm.amegroups.com/article/view/10.21037/atm-21-5448/rc).
Methods
Animal models
We obtained IL-2Rα−/− (B6.129S4-Il2ratm1Dw) and p40−/− (B6.129S1-Il12btm1Jm) mice from The Jackson Laboratory (Bar Harbor, ME, USA), and p40−/−IL-2Rα−/− mice were subsequently generated using backcrossing. All mice were housed in a specific pathogen-free (SPF) environment. All mice experiments were approved by the Animal Ethics Committee of the South China University of Technology (No. 2017002), in compliance with the South China University of Technology guidelines for the care and use of animals.
Administration of antibiotics
For antibiotics (ABX) mice models, the p40−/−IL-2Rα−/− mice were administered a cocktail of broad-spectrum ABX in their drinking water from 4 weeks until 12 weeks of age. The cocktail consisted of 1 mg/mL ampicillin, 0.35 mg/mL vancomycin, 1 mg/mL metronidazole, and 1 mg/mL neomycin (Sigma, St. Louis, MO, USA) in drinking water, and fresh antibiotics were administered every 3 days.
Liver histological grading
Hematoxylin and eosin (H&E) staining of liver tissue was conducted as reported previously. The dimension of liver inflammation and bile duct damage was assessed by pathologists in a blind manner. The scores of portal inflammation, lobular inflammation, and bile duct injury were generated from the sum of the score of severity and frequency. First, the extent of portal and lobular inflammation was assessed according to the most serious lesions, and the score was as follows: 0, no change; 1, minimal inflammation; 2, mild inflammation; 3, moderate inflammation; and 4, severe inflammation. Similarly, the dimension of bile duct injury was evaluated according to the severity of the most serious lesions, and scored as follows: 0, no change; 1, epithelial damage (only cytoplasmic changes); 2, epithelial damage with cytoplasmic and nuclear changes; 3, nonsuppurative destructive cholangitis (NSDC); and 4, bile duct loss. Frequency of bile duct injury was determined by the percentage of affected bile duct of each specimen, and scored as follows: 0, none; 1, 1–10%; 2, 11–20%; 3, 21–50%; and 4, over 50%.
Liver and spleen cell isolation
Liver cells were isolated according to a previously reported method (24). In brief, fresh liver tissue was ground with a syringe handle, filtrated through a 200-mesh strainer, and temporarily kept in phosphate-buffered saline (PBS) with 0.2% bovine serum albumin (BSA). Then, the hepatic parenchymal and red blood cells were removed.
Splenomegaly was defined as a spleen weight of over 0.3 g (the mean weight of the spleens of the p40−/−IL-2Rα−/− mice). The spleen was homogenized with 2 glass slides, suspended in PBS with 0.2% BSA, filtrated through a 200-mesh sieve, and erythrocytes were removed. The cell number was counted using a cell counting chamber with a microscope.
Flow cytometry
We incubated 1×106 cells with anti-mouse CD16/CD32 antibody [Becton, Dickinson, and Co. (BD) Biosciences, Franklin Lakes, NJ, USA USA] for 15 min at 4 ℃ to block Fc-receptors and avoid nonspecific binding. Cells were then stained for 20 minutes at 4 ℃ with a mix of fluorescent antibodies, including PacificBlue-CD3 (17A2, BioLegend, San Diego, CA, USA), PE/Cy7-NK1.1 (PK136, BioLegend), V500-CD8α (53-6.7, BioLegend), APC/Cy7-CD4 (RM4-5, BD Biosciences), FITC-CD44 (IM7, eBioscience, San Diego, CA, USA), FITC-Gr-1 (RB6-8C5, BD Biosciences), PerCP/Cy5.5-CD11c (N418, BioLegend), APC-CD11b (M1/70, BioLegend), BV510-B220 (RA3-6B2, BioLegend), and PerCP/Cy5.5-CD62L (MEL-14, BioLegend). Stained cells were detected using a FACSVerse Flow Cytometer (BD Biosciences). Flow data were analyzed using FlowJo software (Tree Star, Ashland, OR, USA).
Statistical analysis
All data were presented as the mean ± standard error of the mean (SEM), and differences were tested for using a 2-tailed unpaired Student’s t-test using GraphPad Prism (GraphPad Software Inc., San Diego, CA, USA). We considered P<0.05 to indicate statistical significance.
Results
Biliary inflammation following splenomegaly
When p40−/−IL-2Rα−/− mice were analyzed according to the presence of splenomegaly, liver mononuclear cells (MNCs) were present in significantly higher numbers in the presence of splenomegaly (Figure 1). Similarly, histological scores of portal inflammation, lobular inflammation, and bile duct damage were significantly higher in mice with splenomegaly (Figure 1), which also manifested as enlarged peripheral lymph nodes (Figure S1), indirectly supporting the link between liver inflammation and splenomegaly.
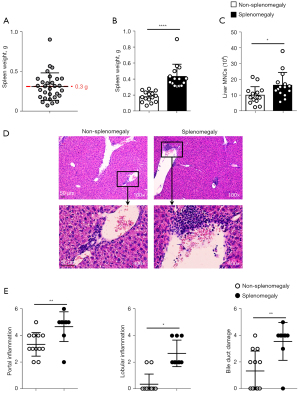
Liver and spleen immune cells
In agreement with our previous data, the absolute number and total proportion of liver CD4+, and CD8+ T cells were significantly higher in the presence of splenomegaly, particularly when activated effector memory CD4+ T (CD4+ Tem) and CD8+ T (CD8+ Tem) cells (CD44+CD62L−) were evaluated (Figure 2). Conversely, there were no differences with splenomegaly in liver central memory CD8+ T (CD8+ Tcm) cells (CD44+CD62L+) (Figure 2).
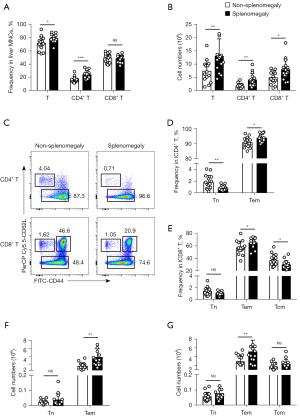
Spleen MNCs showed no significant associations with splenomegaly (Figure 3), while we observed a decrease in granulocytes (Gr-1hiCD11b+) and B cells (B220+CD11c−). CD8+ Tem but not CD4+ Tem cells were increased in the enlarged spleen (Figure 3). The gating strategy image of flow cytometry is shown in Figure S1B.
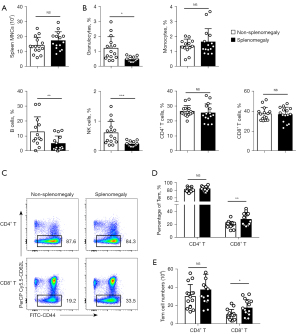
Effects of antibiotic treatment on histology and immune cells
We used combined antibiotics treatment, a standard and accepted method (25), in p40−/−IL-2Rα−/− mice to explore the effect of gut microbiota on splenomegaly. The spleen weight and the number of spleen and liver MNCs were reduced following the antibiotics combination treatment, ultimately preventing splenomegaly, while the spleen weight was similar in p40−/−IL-2Rα−/− mice treated with antibiotics and WT C57BL/6 mice (Figure 4). The scores of portal inflammation, lobular inflammation, and bile duct damage in mice treated with antibiotics also decreased (Figure 4). These results were not observed when single antibiotics were used (Figure S2).
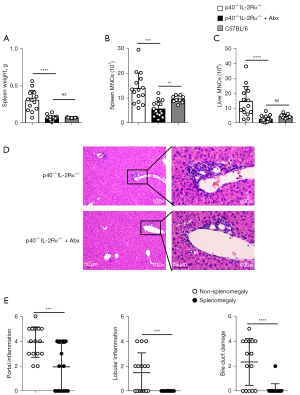
We observed a significant reduction of liver T cells and CD4+ T and CD8+ T cell subsets both in terms of frequency and number following treatment with four antibiotics (Figure 5). The changes of CD4+ T cell paralleled the decrease of CD4+ Tem subsets and the increase of naïve CD4+ T cell subsets, and similar changes were demonstrated in CD8+ T cells (Figure 5).
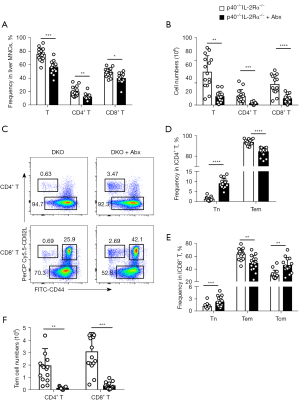
The frequency of spleen NK and B cells was increased, while CD4+ T and CD8+ T cells were reduced in p40−/−IL-2Rα−/− mice treated with antibiotics (Figure 6) with the resulting proportions being similar to wild-type mice.
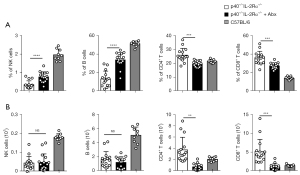
Discussion
Our p40−/−IL-2Rα−/− mouse is a PBC model, which spontaneously develops portal lymphocytic infiltrates, liver inflammation, biliary ductular damage, and other autoimmune phenotypes modelling human disease, often with splenomegaly (26), enhanced liver inflammation, and less severe colitis compared to the IL-2Rα−/− model (24). Here, we reported for the first time that splenomegaly is associated with more severe liver inflammation in our PBC murine model, and that this effect is reversed by a quadruple antibiotic treatment.
The spleen serves as the largest secondary lymphoid organ in the body and plays an important immunological role to modulate inflammation (27). Splenomegaly is associated with multiple conditions (28,29) through different mechanisms (30), in some cases resulting in spleen hyperactivity (31). Infections such as malaria, measles, typhoid fever, and viral hepatitis, as well as immune-mediated disorders, such as immune thrombocytic purpura, rheumatoid arthritis, and PBC (32-36), lead to follicular hyperplasia of the spleen, and it is thus not counterintuitive to hypothesize that splenomegaly may play a role in modulating chronic inflammation. Nonetheless, while the infiltration with inflammatory cells contributes to splenomegaly, the number of spleen MNCs showed no difference between p40−/−IL-2Rα−/− mice according to spleen weight.
The liver and gut communicate directly through the biliary tract and portal circulation (37), and this provides an anatomical link to explain how intestinal bacteria may translocate to the liver via increased intestinal permeability (38). Pathogen-associated molecular patterns (PAMPs) are recognized by immune receptors on liver Kupffer cells and stellate cells (39), which activate T cells and initiate inflammatory cascade that ultimately leads to chronic inflammation and liver damage (40). We observed that splenomegaly is associated with more activated T cells infiltrating the liver, and that these effects were reversed by antibiotics, which also ameliorated splenomegaly, thus supporting the hypothesis that gut microbiota changes contribute to splenomegaly and possibly vice versa.
The spleen is a site where innate and adaptive immune responses against pathogens are initiated (41) and this process is disturbed in splenomegaly, particularly affecting T cells (42). Macrophages are the main innate immune cells in the spleen and play a pivotal role in splenomegaly due to their responsibility for the removal of old erythrocytes and apoptotic cells. Increased numbers of macrophages have been detected in the enlarged spleen caused by Schistosoma infection (43); however, we did not observe a significant change of monocytes in enlarged spleens of p40−/−IL-2Rα−/− mice. In our experimental setting, we observed a change of several immune cells in the enlarged spleen, including granulocytes, B, NK, and CD8+ Tem cells, while quadruple antibiotics caused a significant reduction of total spleen CD4+ T and CD8+ T cells, which may impair the immune response. However, the gut microbiota consisted of probiotic microbiota and pathogenic microbiota. Thus, identifying and specifically eliminating the pathogenic gut microbiota should be investigated in future work.
In conclusion, we demonstrated that the gut microbiota is associated with splenomegaly in a PBC murine model by inducing liver inflammation, and that antibiotics treatment alleviates both splenomegaly and immune cell changes. The gathered evidence suggests that novel approaches to splenomegaly and hypersplenism may result from microbiome modulation in an individualized fashion.
Acknowledgments
We would like to thank Carlo Selmi and Eric Gershwin for their help in polishing our paper.
Funding: This work was supported by the Guangdong Introducing Innovative and Entrepreneurial Teams (2017ZT07S054), the National Natural Science Foundation of China (82001730, 81873877, and 81671600), the Natural Science Foundation of Shandong Grants (ZR2016HM13), and the Fundamental Research Funds for the Central Universities in the South China University of Technology (2020ZYGXZR045).
Footnote
Reporting Checklist: The authors have completed the ARRIVE reporting checklist. Available at https://atm.amegroups.com/article/view/10.21037/atm-21-5448/rc
Data Sharing Statement: Available at https://atm.amegroups.com/article/view/10.21037/atm-21-5448/dss
Conflicts of Interest: All authors have completed the ICMJE uniform disclosure form (available at https://atm.amegroups.com/article/view/10.21037/atm-21-5448/coif). The authors have no conflicts of interest to declare.
Ethical Statement: The authors are accountable for all aspects of the work in ensuring that questions related to the accuracy or integrity of any part of the work are appropriately investigated and resolved. All mice experiments were approved by the Animal Ethics Committee of the South China University of Technology (No. 2017002), in compliance with the South China University of Technology guidelines for the care and use of animals.
Open Access Statement: This is an Open Access article distributed in accordance with the Creative Commons Attribution-NonCommercial-NoDerivs 4.0 International License (CC BY-NC-ND 4.0), which permits the non-commercial replication and distribution of the article with the strict proviso that no changes or edits are made and the original work is properly cited (including links to both the formal publication through the relevant DOI and the license). See: https://creativecommons.org/licenses/by-nc-nd/4.0/.
References
- Pozo AL, Godfrey EM, Bowles KM. Splenomegaly: investigation, diagnosis and management. Blood Rev 2009;23:105-11. [Crossref] [PubMed]
- Valdés-Ferrer SI, Rosas-Ballina M, Olofsson PS, et al. HMGB1 mediates splenomegaly and expansion of splenic CD11b+ Ly-6C(high) inflammatory monocytes in murine sepsis survivors. J Intern Med 2013;274:381-90. [Crossref] [PubMed]
- Xu R, Zhang Z, Wang FS. Liver fibrosis: mechanisms of immune-mediated liver injury. Cell Mol Immunol 2012;9:296-301. [Crossref] [PubMed]
- Barosi G, Bergamaschi G, Marchetti M, et al. JAK2 V617F mutational status predicts progression to large splenomegaly and leukemic transformation in primary myelofibrosis. Blood 2007;110:4030-6. [Crossref] [PubMed]
- Ingle SB, Ingle CR. Splenic lymphoma with massive splenomegaly: Case report with review of literature. World J Clin Cases 2014;2:478-81. [Crossref] [PubMed]
- Wagner KU, Claudio E, Rucker EB 3rd, et al. Conditional deletion of the Bcl-x gene from erythroid cells results in hemolytic anemia and profound splenomegaly. Development 2000;127:4949-58. [Crossref] [PubMed]
- Motta I, Filocamo M, Poggiali E, et al. A multicentre observational study for early diagnosis of Gaucher disease in patients with Splenomegaly and/or Thrombocytopenia. Eur J Haematol 2016;96:352-9. [Crossref] [PubMed]
- Sharma P, Kirnake V, Tyagi P, et al. Spleen stiffness in patients with cirrhosis in predicting esophageal varices. Am J Gastroenterol 2013;108:1101-7. [Crossref] [PubMed]
- Tremblay D, Schwartz M, Bakst R, et al. Modern management of splenomegaly in patients with myelofibrosis. Ann Hematol 2020;99:1441-51. [Crossref] [PubMed]
- Gorodetskiy V, Probatova N, Sidorova Y, et al. The non-leukemic T cell large granular lymphocytic leukemia variant with marked splenomegaly and neutropenia in the setting of rheumatoid arthritis - Felty syndrome and hepatosplenic T cell lymphoma mask. Am J Blood Res 2021;11:227-37. [PubMed]
- Idilman IS, Venkatesh SH, Eaton JE, et al. Magnetic resonance imaging features in 283 patients with primary biliary cholangitis. Eur Radiol 2020;30:5139-48. [Crossref] [PubMed]
- Ma HD, Zhao ZB, Ma WT, et al. Gut microbiota translocation promotes autoimmune cholangitis. J Autoimmun 2018;95:47-57. [Crossref] [PubMed]
- Rosser EC, Mauri C. A clinical update on the significance of the gut microbiota in systemic autoimmunity. J Autoimmun 2016;74:85-93. [Crossref] [PubMed]
- Yang W, Cong Y. Gut microbiota-derived metabolites in the regulation of host immune responses and immune-related inflammatory diseases. Cell Mol Immunol 2021;18:866-77. [Crossref] [PubMed]
- Barrea L, Di Somma C, Muscogiuri G, et al. Nutrition, inflammation and liver-spleen axis. Crit Rev Food Sci Nutr 2018;58:3141-58. [Crossref] [PubMed]
- Zhu H, Liu Y, Li S, et al. Altered gut microbiota after traumatic splenectomy is associated with endotoxemia. Emerg Microbes Infect 2018;7:197. [Crossref] [PubMed]
- Wei Y, Chang L, Ishima T, et al. Abnormalities of the composition of the gut microbiota and short-chain fatty acids in mice after splenectomy. Brain Behav Immun Health 2021;11:100198. [Crossref] [PubMed]
- Liu Y, Li J, Jin Y, et al. Splenectomy Leads to Amelioration of Altered Gut Microbiota and Metabolome in Liver Cirrhosis Patients. Front Microbiol 2018;9:963. [Crossref] [PubMed]
- Rosado MM, Aranburu A, Scarsella M, et al. Spleen development is modulated by neonatal gut microbiota. Immunol Lett 2018;199:1-15. [Crossref] [PubMed]
- Khosravi A, Yáñez A, Price JG, et al. Gut microbiota promote hematopoiesis to control bacterial infection. Cell Host Microbe 2014;15:374-81. [Crossref] [PubMed]
- Wang G, Wu X, Zhu G, et al. Dexmedetomidine alleviates sleep-restriction-mediated exaggeration of postoperative immunosuppression via splenic TFF2 in aged mice. Aging (Albany NY) 2020;12:5318-35. [Crossref] [PubMed]
- Zhang Y, Xie B, Chen X, et al. A key role of gut microbiota-vagus nerve/spleen axis in sleep deprivation-mediated aggravation of systemic inflammation after LPS administration. Life Sci 2021;265:118736. [Crossref] [PubMed]
- Carsetti R, Di Sabatino A, Rosado MM, et al. Lack of Gut Secretory Immunoglobulin A in Memory B-Cell Dysfunction-Associated Disorders: A Possible Gut-Spleen Axis. Front Immunol 2019;10:2937. [Crossref] [PubMed]
- Yao Y, Yang W, Yang YQ, et al. Distinct from its canonical effects, deletion of IL-12p40 induces cholangitis and fibrosis in interleukin-2Rα(-/-) mice. J Autoimmun 2014;51:99-108. [Crossref] [PubMed]
- Iida N, Dzutsev A, Stewart CA, et al. Commensal bacteria control cancer response to therapy by modulating the tumor microenvironment. Science 2013;342:967-70. [Crossref] [PubMed]
- Terziroli Beretta-Piccoli B, Mieli-Vergani G, Vergani D, et al. The challenges of primary biliary cholangitis: What is new and what needs to be done. J Autoimmun 2019;105:102328. [Crossref] [PubMed]
- Mebius RE, Kraal G. Structure and function of the spleen. Nat Rev Immunol 2005;5:606-16. [Crossref] [PubMed]
- Curovic Rotbain E, Lund Hansen D, Schaffalitzky de Muckadell O, et al. Splenomegaly - Diagnostic validity, work-up, and underlying causes. PLoS One 2017;12:e0186674. [Crossref] [PubMed]
- Sjoberg BP, Menias CO, Lubner MG, et al. Splenomegaly: A Combined Clinical and Radiologic Approach to the Differential Diagnosis. Gastroenterol Clin North Am 2018;47:643-66. [Crossref] [PubMed]
- Bedu-Addo G, Bates I. Causes of massive tropical splenomegaly in Ghana. Lancet 2002;360:449-54. [Crossref] [PubMed]
- McKenzie CV, Colonne CK, Yeo JH, et al. Splenomegaly: Pathophysiological bases and therapeutic options. Int J Biochem Cell Biol 2018;94:40-3. [Crossref] [PubMed]
- Swaroop J, O'Reilly RA. Splenomegaly at a university hospital compared to a nearby county hospital in 317 patients. Acta Haematol 1999;102:83-8. [Crossref] [PubMed]
- Leoni S, Buonfrate D, Angheben A, et al. The hyper-reactive malarial splenomegaly: a systematic review of the literature. Malar J 2015;14:185. [Crossref] [PubMed]
- Haqshenas G, Shivaprasad HL, Woolcock PR, et al. Genetic identification and characterization of a novel virus related to human hepatitis E virus from chickens with hepatitis-splenomegaly syndrome in the United States. J Gen Virol 2001;82:2449-62. [Crossref] [PubMed]
- Scherer HU, Häupl T, Burmester GR. The etiology of rheumatoid arthritis. J Autoimmun 2020;110:102400. [Crossref] [PubMed]
- Sun C, Xiao X, Yan L, et al. Histologically proven AMA positive primary biliary cholangitis but normal serum alkaline phosphatase: Is alkaline phosphatase truly a surrogate marker? J Autoimmun 2019;99:33-8. [Crossref] [PubMed]
- Wang R, Tang R, Li B, et al. Gut microbiome, liver immunology, and liver diseases. Cell Mol Immunol 2021;18:4-17. [Crossref] [PubMed]
- Ju C, Tacke F. Hepatic macrophages in homeostasis and liver diseases: from pathogenesis to novel therapeutic strategies. Cell Mol Immunol 2016;13:316-27. [Crossref] [PubMed]
- Acharya C, Sahingur SE, Bajaj JS. Microbiota, cirrhosis, and the emerging oral-gut-liver axis. JCI Insight 2017;2:94416. [Crossref] [PubMed]
- Tilg H, Cani PD, Mayer EA. Gut microbiome and liver diseases. Gut 2016;65:2035-44. [Crossref] [PubMed]
- Bronte V, Pittet MJ. The spleen in local and systemic regulation of immunity. Immunity 2013;39:806-18. [Crossref] [PubMed]
- Li Y, Yue H, Yang S, et al. Splenomegaly induced by anemia impairs T cell movement in the spleen partially via EPO. Mol Immunol 2019;112:399-405. [Crossref] [PubMed]
- Kong D, Zhou C, Guo H, et al. Praziquantel Targets M1 Macrophages and Ameliorates Splenomegaly in Chronic Schistosomiasis. Antimicrob Agents Chemother 2018;62:e00005-17. [Crossref] [PubMed]
(English Language Editors: B. Meiser and J. Jones)