3D orientation and kinematic characteristics of zygapophyseal joints while sitting
Introduction
The sitting position is widely chosen as the position for work or rest in daily life (1,2). Unlike common positions, such as supine and standing, the sitting position is characterized by a greater load on the lumbar spine and is often accompanied by lower back pain (LBP) (3,4). Some young LBP patients have no significant changes in imaging (5). In physiological situations, LBP is often associated with abnormal activity of some tissues, such as the facet joint (FJ), intervertebral discs, ligaments, and muscles (6). Previously published studies on the causes of LBP in the sitting position have focused on the intervertebral discs and muscles (7,8). However, as one of the structures that supports and maintains stability in the spine, the FJ has been identified as a potential source of pain, and its degeneration can also accelerate disc degeneration and lumbar spondylolisthesis. The orientation and kinematic characteristics of FJ are important to understand sitting-related LBP (9). In previous studies of other functional activities, facet tropism (FT) and potential abnormal activity under prolonged loading have been widely recognized as causes of LBP (10-12).
FT refers to differences in orientation between the right and left FJs of the lumbosacral spine (13). Previously published studies have shown that FT increases the shear force on the intervertebral disc, resulting in abnormal strain distribution and motion patterns in the lumbar spine, which in turn induces intervertebral disc degeneration (14-16). Several methods (such as computer tomography and direct measurement on cadaver specimens) have been used to describe the FT and to verify the relationship between joint asymmetry and degenerative diseases of the lumbar spine (17,18). Due to the limitations of experimental techniques, most previous studies have been based on the measurement in the transverse plane, lacking 3D measurements, such as sagittal and coronal facet angles.
In addition, the in vivo kinematic parameters of the FJ in the physiological state can be used as a baseline for assessing traumatic and degenerative changes, and as a reference for judging the effectiveness of posterior element replacement surgical techniques (17). Previous kinematic assessments of the FJ of the lumbar spine have been performed in the standing position; however, the kinematic characteristics of the FJ in the sitting position are not known (19).
To investigate the 3D orientation and in vivo motion characteristics of the FJ in the sitting position, we used the dual fluoroscopic image system (DFIS) and computed tomography (CT) to measure the 3D facet angle and kinematic characteristics of the L3-L4, L4-L5, and L5-S1 segments in healthy participants in the sitting position. In addition, we explored the relationship between lumbar FJ motion and its 3D orientation, hoping to analyze the kinematics parameters in sitting position from the perspective of 3D orientation of lumbar FJs in different planes. We present the following article in accordance with the MDAR reporting checklist (available at https://atm.amegroups.com/article/view/10.21037/atm-22-969/rc).
Methods
Participant recruitment
Ten asymptomatic participants (5 male and 5 female), aged 25–35 years with a body mass index of 22.4±1.8, were recruited in the present study. Study of asymptomatic participants is representative of lumbar spine kinematic changes in a healthy population. Exclusion criteria included scoliosis, history of spinal surgery, symptoms or history of LBP, functional spinal problems, and musculoskeletal lesions of the lower extremities or neurological disorders in the past 12 months. The study was conducted in accordance with the Declaration of Helsinki (as revised in 2013). The study was approved by the Research Ethics Committee of Tianjin Hospital of Tianjin University and informed consent was taken from all the patients.
Reconstruction of 3D models of the lumbar spine
The L3-S1 segments (thickness of 0.625 mm with no gap) of all participants were scanned in the supine position with CT with a resolution of 512×512 pixels. The obtained lumbar scans were then imported into MIMICS version 19.0 (Materialise, Leuven, Belgium) for reconstruction. We chose specific bony thresholds to better separate the surrounding soft tissues from the lumbar spine and obtained the reconstructed lumbar spine with FJ (L3, L4, L5, S1) (Figure 1).
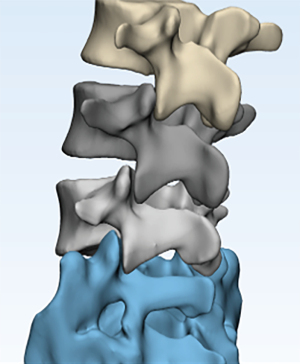
Instantaneous motion acquisition of the lumbar spine
The participant was seated on a height-adjustable seat with feet shoulder width apart, lower legs perpendicular to the ground, thighs parallel to the ground, arms hanging in front of the chest, and hands clenched in fists on either side of the neck. This posture was maintained throughout the experiment. The 2 fluoroscopes (Phillips, Berlin, Germany) were positioned crosswise, but at a distance to avoid image interference due to diffraction of X-rays. Height-adjustable seats were placed near the receivers. Participants were asked to perform maximum flexion to maximum extension movements in the sitting position to simulate daily life, and fluoroscopic images were collected in the neutral sitting position, maximum forward flexion sitting, and maximum extension sitting (Figure 2). The range of motion was at the individual’s maximum tolerance and did not exceed the fluoroscopes range. Two transient lumbar spine fluoroscopic images were obtained separately in each position.
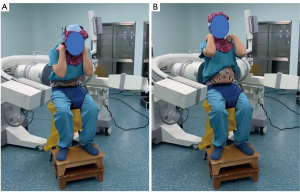
Reproduction of in vivo lumbar spine motion in virtual space
According to the method of Li et al. (20), a set of orthogonal virtual camera sources and receivers were created in Rhinoceros (Robert McNeel & Associates, Seattle, WA, USA) software to simulate the location of the fluoroscopic image intensifier in a real situation. Lumbar spine images were acquired by the fluoroscopes, and a 3D reconstructed lumbar spine model was imported simultaneously. Lumbar spine edges were then depicted by tracing the difference in pixel values of the lumbar spine in the 2 perspective images. The reconstructed 3D model of the lumbar spine is translated and rotated so that its contour coincides with the outline of the bony feature drawn on the fluoroscopic images (Figure 3). With this method, the actual position of the in vivo FJ could be reproduced.
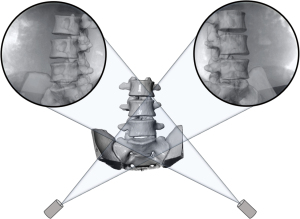
Establishment of a coordinate system of the FJ
Based on Chowdhury et al.’s method (12), a Cartesian coordinate system was established on the articular surface of each zygapophyseal joint, and the motion of the coordinate system was used to estimate the kinematic of the joint. Four points were selected at the uppermost, lowermost, innermost, and outermost sides of the surfaces. The origin of the coordinate system is the center of mass of the geometry formed by the 4 points. The vector connecting the innermost and outermost points was defined as the Y-axis; similarly, the vector passing through the line connecting the lowermost and uppermost points was defined as the Z-axis; the vector orthogonal to the Y–Z plane was defined as the X-axis; the coordinate system was established following the right-hand rule (Figure 4).
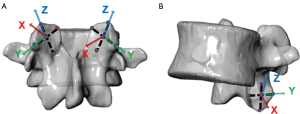
Definition and measurement of FJ orientation
The 3D facet angles were defined and measured in the sagittal, coronal, and transverse planes, respectively. The sagittal facet angle (α) was defined as the angle between the projection of the line connecting the uppermost and lowermost points of the FJ in the sagittal plane and the transverse axis (Figure 5A). The coronal facet angle (β) was defined as the angle between the projection of the line connecting the uppermost and lowermost points of the FJ on the coronal plane and the transverse axis (Figure 5B). The transverse facet angle (γ) was defined as the angle between the projection of the line connecting the medial-most and lateral-most points of the FJ on the transverse plane and the sagittal axis (Figure 5C).
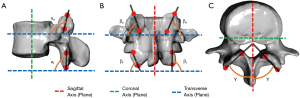
Measurement of FJ motion
The range of rotation within the FJ was calculated by measuring the motion of the inferior joint surface of the upper segment relative to the superior joint surface of the lower segment, with the superior articular surface as a reference (Figure 6). The following kinematic parameters could be obtained: (I) the range of rotation during the forward-flexion-backward extension motion in the sitting position; (II) the decomposition of the forward-flexion-backward extension motion of the lumbar spine into the following 2 submotion processes: neutral-anterior flexion and neutral-backward extension, to obtain the range of rotation of the FJ, respectively, and to compare the difference in the mobility of the 2 submotion processes.
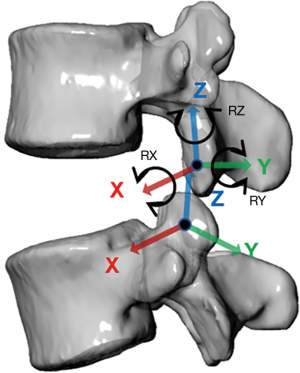
Statistical analysis
Statistical analysis was performed using SPSS version 26.0 (IBM, Armonk, NY, USA). The facet angles of the FJ in the sagittal, coronal, and transverse planes were described as mean ± standard deviation. The results of all kinematic parameters were expressed as P50 (P25, P75). The differences in the mobility of the left and right FJ in flexion and extension movements were compared using Kruskal-Wallis test. Pearson correlation analysis was performed between the 3D facet angle and the mobility of the joint. Statistical significance was set at P<0.05.
Results
3D measurement of FJ orientation
In the sagittal plane, the left superior facet angle of the L5 was 1.9° smaller than that of the right, and the remaining segments were greater on the left than on the right, with differences ranging from 0.5° to 1.8°, with no statistically significant differences (Table 1). In the coronal plane, the left side of the L5 vertebra had a significantly smaller facet angle of 6.4° than the right side (P=0.01), and the other 2 sides had close angles, with differences ranging from 0 to 0.7°, with no statistically significant differences (Table 2). In the transverse plane, the difference between the 2 sides of the inferior facet angles was greater in L4 and L5, with the left side being 1.8° smaller than the right side in the L4; the left side was 7.1° greater than the right side in the L5, with no statistically significant difference. The other segments had a slightly larger facet angle on the left side than the right side in the transverse plane, with a difference ranging from 0° to 0.7° (Table 3).
Table 1
Segment | Left | Right | P value |
---|---|---|---|
Inferior | |||
L3 | 79.3±6.0 | 77.5±5.1 | 0.710 |
L4 | 78.7±5.7 | 78.2±3.8 | 1 |
L5 | 68.4±4.4 | 66.6±4.9 | 0.710 |
Superior | |||
L4 | 98.4±2.3 | 96.6±4.5 | 0.710 |
L5 | 95.5±4.8 | 97.4±5.7 | 0.318 |
S | 104.2±8.3 | 103.1±6.8 | 0.805 |
Table 2
Segment | Left | Right | P value |
---|---|---|---|
Inferior | |||
L3 | 88.5±7.3 | 88.5±3.9 | 0.805 |
L4 | 90.4±5 | 87.7±6.2 | 0.535 |
L5 | 77.7±6.7 | 77.2±8.8 | 0.902 |
Superior | |||
L4 | 78.8±2.1 | 79.1±4.4 | 0.620 |
L5 | 74.9±3.3 | 81.3±4.3 | 0.010* |
S | 82.7±2.2 | 82.7±4.5 | 0.902 |
*, statistically significant differences at P<0.05.
Table 3
Segment | Left | Right | P value |
---|---|---|---|
Inferior | |||
L3 | 40.9±11.3 | 40.8±10 | 0.805 |
L4 | 48.1±13.3 | 49.9±13.1 | 1.000 |
L5 | 52.2±9.1 | 45.1±13.7 | 0.383 |
Superior | |||
L4 | 41.9±7.7 | 41.8±6.3 | 0.805 |
L5 | 48.7±8.8 | 48±11.5 | 1.000 |
S | 55.4±7.6 | 54.4±10.9 | 0.805 |
Lumbar FJ range of rotation
The range of motion of the FJ on the right side of L5-S1 was 8.8° and 14.9° in the sagittal and coronal planes during forward flexion and backward extension movements in the seated position, which were 5.5° (P=0.004) and 11.7° (P=0.026) greater than those on the left side, respectively (Figure 7A-7F). In the transverse plane, the range of rotation of the right side was slightly greater than that of the left in L5-S1, with no statistically significant differences (Figure 7G-7I). In the FJ of L3-L4 and L4-L5, the range of motion was slightly greater on the left side than on the right side in both L3-L4 and L4-L5. The range of rotation was 1.7° and 1.2° greater on the left side than on the right side in the sagittal plane, 0.8° and 0.9° greater in the coronal plane, and 0.4° and 1.8° greater in the transverse plane, respectively.
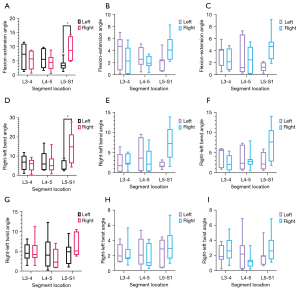
The sitting flexion and extension motions were divided into neutral sitting to forward flexion sitting and neutral sitting to posterior extension sitting to compare the mobility of the FJ during sitting forward flexion and posterior extension, respectively. During both submovements, the range of rotation of the FJ on the left side of L5-S1 was smaller than that on the right side in all 3 planes. In the sagittal plane, the range of rotation of the left FJ of L3-L4, L4-L5, and L5-S1 during anterior flexion was greater than that during posterior extension, increasing by 0.7°, 1.4°, and 1.1°, respectively; the range of rotation of the right FJ of L4-L5 and L5-S1 during anterior flexion was less than that during posterior extension, decreasing by 0.6°, while L3-L4 tended to be opposite to that of the caudal segment, increasing by 0.2° (Figure 7B,7C). In the coronal plane, the range of rotation of the left FJ of L3-L4 differed more in submotion, with the range of motion during forward flexion being 3.8° smaller than that during posterior extension (Figure 7E,7F). In the transverse plane, the range of rotation of the left FJ in L5-S1 was 1° greater during forward flexion than during posterior extension, and the right FJ in L3-L4 was 0.9° smaller during forward flexion than during posterior extension (Figure 7H, 7I). The range of rotation of the FJ on both sides of the remaining lumbar segments was less variable in the submovements, with a range of rotation between 0° and 0.3°.
Relationship between facet angle and motion
The magnitude of the left-side facet angles in the 3 planes correlated more with FJ motion. The range of rotation of the L3-L4 left joint in the coronal plane showed a positive correlation with the coronal inferior facet angle in L3 (P=0.016) and a negative correlation with the transverse facet angles of the L3 joints (Table 4). The range of rotation of the L4-L5 left joint in the coronal plane showed a negative correlation with the sagittal inferior facet angle of the L4 left joint (P=0.029) (Table 4). The range of rotation of the L5-S1 left joint in the sagittal and coronal planes showed a positive correlation (P=0.03) with the sagittal superior facet angle and a negative correlation (P=0.03) with the transverse superior facet angle in the S1 left joint (Table 4).
Table 4
Projection angles | Articular surface angle | Statistics | Left | Right | |||||
---|---|---|---|---|---|---|---|---|---|
L3-L4 | L4-L5 | L5-S1 | L3-L4 | L4-L5 | L5-S1 | ||||
Flexion-extension ROM | |||||||||
α | IFA | r | –0.01 | –0.6 | –0.1 | 0.1 | –0.02 | 0.4 | |
P | 0.986 | 0.137 | 0.875 | 0.894 | 0.962 | 0.315 | |||
SFA | r | 0.2 | –0.4 | 0.8 | 0.1 | 0.6 | 0.2 | ||
P | 0.538 | 0.334 | 0.033* | 0.841 | 0.136 | 0.734 | |||
β | IFA | r | 0.7 | –0.2 | 0.7 | 0.6 | 0.2 | 0.4 | |
P | 0.08 | 0.636 | 0.066 | 0.133 | 0.614 | 0.34 | |||
SFA | r | 0.6 | 0.4 | 0.3 | –0.1 | –0.2 | 0.5 | ||
P | 0.193 | 0.326 | 0.459 | 0.895 | 0.747 | 0.204 | |||
γ | IFA | r | –0.5 | 0.2 | –0.4 | –0.7 | 0.4 | 0.05 | |
P | 0.284 | 0.746 | 0.39 | 0.054 | 0.414 | 0.919 | |||
SFA | r | –0.6 | 0.2 | –0.6 | –0.5 | 0.4 | –0.1 | ||
P | 0.191 | 0.734 | 0.18 | 0.208 | 0.416 | 0.784 | |||
Right-left bending ROM | |||||||||
α | IFA | r | –0.1 | –0.8 | 0.1 | 0.02 | 0.3 | 0.3 | |
P | 0.762 | 0.029* | 0.864 | 0.965 | 0.55 | 0.558 | |||
SFA | r | 0.5 | –0.2 | 0.8 | 0.1 | 0.7 | –0.05 | ||
P | 0.224 | 0.66 | 0.029* | 0.835 | 0.07 | 0.923 | |||
β | IFA | r | 0.8 | –0.4 | 0.9 | 0.6 | 0.2 | 0.6 | |
P | 0.016* | 0.422 | 0.001* | 0.129 | 0.621 | 0.144 | |||
SFA | r | 0.6 | 0.5 | 0.6 | –0.2 | 0.1 | 0.3 | ||
P | 0.178 | 0.251 | 0.126 | 0.741 | 0.77 | 0.494 | |||
γ | IFA | r | –0.8 | –0.1 | –0.5 | –0.7 | 0.1 | –0.04 | |
P | 0.048* | 0.802 | 0.296 | 0.058 | 0.82 | 0.934 | |||
SFA | r | –0.8 | –0.03 | –0.8 | –0.5 | 0.1 | –0.2 | ||
P | 0.016* | 0.946 | 0.029* | 0.306 | 0.759 | 0.722 | |||
Right-left twisting ROM | |||||||||
α | IFA | r | –0.5 | 0.1 | –0.4 | 0.3 | 0.4 | 0.3 | |
P | 0.205 | 0.83 | 0.318 | 0.5 | 0.403 | 0.462 | |||
SFA | r | –0.1 | –0.3 | 0.5 | 0.3 | 0.3 | 0.4 | ||
P | 0.879 | 0.583 | 0.299 | 0.512 | 0.496 | 0.36 | |||
β | IFA | r | –0.04 | 0.6 | 0.4 | 0.3 | –0.1 | 0.3 | |
P | 0.929 | 0.2 | 0.329 | 0.474 | 0.762 | 0.527 | |||
SFA | r | –0.5 | –0.1 | –0.3 | 0.1 | 0.5 | 0.5 | ||
P | 0.267 | 0.822 | 0.537 | 0.776 | 0.301 | 0.226 | |||
γ | IFA | r | –0.1 | –0.1 | –0.5 | –0.5 | –0.2 | 0.1 | |
P | 0.827 | 0.893 | 0.205 | 0.31 | 0.637 | 0.875 | |||
SFA | r | –0.3 | –0.3 | –0.4 | –0.7 | –0.1 | 0.1 | ||
P | 0.526 | 0.507 | 0.316 | 0.099 | 0.864 | 0.912 |
*, statistically significant differences at P<0.05. α, β, and γ are the projection angles of the small joints in the sagittal, coronal, and transverse planes, respectively. r is the correlation coefficient between the facet angle and joint mobility in different planes. IFA, inferior articular surface angle; SFA superior articular surface angle; ROM, range of motion.
Discussion
Facet orientation and range of motion have been a topic of interest for scholars in studies of the FJ of the lumbar spine. FT is a potential anatomical factor for degenerative changes in the lumbar spine, which could increase the incidence of LBP and disc degeneration (21). In the past, FT and motion characteristics have been investigated in physiological and pathological conditions through in vitro and in vivo experiments. In their in vitro experiment, Masharawi et al. measured the facet orientation of cadaveric specimens, T1-L5 FJs, in the transverse and sagittal planes (22). In their in vivo experiment, Zhou et al. used CT to measure the transverse facet angle on the L1-S1 in 53 patients with lumbar disc herniation and 129 normal young adults (18). Ko et al. used CT to measure the transverse facet angle of L3-L5 in 462 patients with LBP (23). Most previously published studies on FT measurement have been limited to the transverse plane because of the limitation of experimental methods, which cannot fully reflect the orientation of FJ. Chowdhury et al. first quantified the 3D facet angles of L2-S1 in 14 healthy volunteers using a dynamic stereo-radiography system (12). However, 3D anatomical measurements of the FJ orientation have not been reported in the Chinese population. Furthermore, kinematic characteristics in the different activities of the FJ of the lumbar spine have been less frequently reported, and past studies have focused on the different movements in the standing position (12,19,24). Sitting is as common a physiological position as standing in daily life; however, the kinematic changes of FJ in the sitting position have not been studied extensively. The DFIS used in our study is capable of acquiring position information in 3D for each lumbar segment in the transient state. Moreover, compared to other radiographs, it can quantify the 6 degrees of freedom of movement in the bony structures of the joints and accurately measure kinematic changes with a rotational error of only 0.7°, which can better reflect the anatomical and kinematic characteristics of FJ (18). Therefore, we applied this method to study the facet orientation in the physiological situation and the motion characteristics of the FJ during anterior flexion and posterior extension movements in the sitting position.
Results from a previous study have found some parameters of the anatomical characteristics of the FJ. Masharawi et al. found that the articular facet angles of L3-L5 in the cross-section ranged from 40.4° to 56.3°, with good symmetry of the left and right lateral articular facet angles (22). Mohanty et al. found that the transverse facet angle in the physiological situation gradually increases from cephalad to caudal, and the L4-L5 and L5-S1 segments were more likely to show FJ asymmetry (17). Shin et al. found that the incidence of facet disease after total disc replacement increased when the difference between the right and left side facet angles was greater than 5° (13). In our study, we found that the range of articular facet angles of L3-S1 in the transverse plane was between 40.8° and 55.4° and showed a gradual increase from the cephalad to caudal side. Among the L5-S1 FJs, the inferior articular facet angle of L5 increased by 7.1° on the left side compared with the right side, while the difference in other articular facet angles was within 0.7°. The facet angles measured in our study were compared with those from Chowdhury et al.’s study (Figure 8) (12). We found that in the sagittal plane, the inferior facet angles on both sides of L4 and L5 were smaller in Chinese participants, and were specifically more distant from the coronal plane and biased toward the transverse plane, especially in the inferior facet surface of L5. In the coronal plane, the left superior articular surface angles of L4 and L5 in Chinese participants were smaller than those of participants in Chowdhury et al.’s study (12); that is, they were more distant from the sagittal plane and biased toward the transverse plane. The rest of the results were not significant and were all within 1 standard deviation. Thus, the 3D orientation of zygapophyseal joints in the L4 and L5 vertebrae differs in Chinese from other ethnic groups, especially in the sagittal and coronal planes, with the articular surface orientation of L5 being more transverse. Analyzed from the perspective of FT, Chowdhury et al. found that FT was more significant at L3-L4 and L5-S1 (12). Nevertheless, our data suggest that there is 6.4° difference between the left and right lateral superior facet angles in the coronal plane for L5, and a 7.1° difference between the inferior articular surface angles in the transverse plane. The rest of the lumbar segments have similar facet angles on both sides. There could be some differences in 3D articular surface angles in different ethnic groups, and FT is more common in L4-L5 and L5-S1 segments. This could also explain the epidemiological investigation of degenerative spondylolisthesis prone to L4-L5 and disc and degeneration prone to the L5-S1 segment.
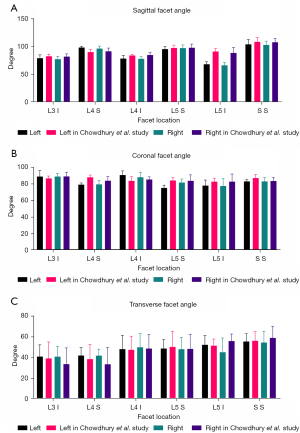
FJ kinematics can reflect the motion of joints in daily activities and help us to analyze the causes of joint degenerative diseases; therefore, it is a topic of interest to scholars. Kozanek et al. found that the range of rotation in the sagittal plane of the L2-L5 in forward flexion and extension in the standing position ranged from 2° to 6°, and the range of rotation in the sagittal plane of L4-L5 was significantly smaller than that of L2–L3 and L3-L4, while there were no significant differences in the 3 segments in the coronal and transverse planes (19). In seated maximal forward flexion and back extension movements, our study found that in the sagittal plane the range of rotation of the L3-S1 was between 3.3° and 8.8°, and the range of rotation of the L4-L5 was less than that of L3-L4, supporting the findings of Kozanek et al. (19). In addition, differences in the mobility of the left and right FJs during forward flexion and backward extension movements were found in our study. The right FJ of L5-S1 was significantly more mobile in the sagittal and coronal planes than the left. However, the range of rotation of the left and right lateral joints of L3-L4 and L4-L5 was less different on the 3 planes. Movement from neutral position to forward flexion and posterior extension showed a similar pattern to the overall forward flexion and posterior extension movement, and the differences in left and right lateral joint mobility were mainly present in L5-S1. There was no significant difference in the range of motion of the FJ in each segment when comparing the 2 submovement processes.
The change in the 3D orientation of the FJ of the lumbar spine could be related to mobility. There have been few previously published studies on this due to the limitation of research conditions. In our study, we characterized the correlation between the 3D orientation of the FJs and their mobility. There was a correlation between the 3D orientation of the left small joint and its mobility. The L3-L4 and L5-S1 facet angles in the transverse plane were negatively correlated with the range of rotation of the joints in the coronal plane. The smaller the angle of the FJ on the transverse plane, that is, the closer the joint surface is to the sagittal plane, the greater its range of motion in the left-right side bending. In the sagittal plane, the L4 inferior facet angle was negatively correlated with the range of rotation of FJ in the coronal plane, and the S1 superior facet angle was positively correlated with it. The angle in the sagittal plane deviated from the sagittal plane and was close to the transverse plane; the corresponding FJ had greater mobility of the lateral bending. The change of facet angle in the transverse plane influenced the axial torsional resistance, especially when the facet angle was biased toward the sagittal plane, decreasing axial torsional resistance (25). Reduced torsional resistance increases the rotational mobility of the FJ, which in turn increases the rotational strain on the disc, making lumbar disc degeneration more likely to occur unilaterally (17). Similar to the change in facet angle in the transverse plane, the facet angle in the sagittal plane was close to the transverse plane, and the corresponding articular surface with which the FJs are formed has a reduced resistance to rotation and a corresponding increase in mobility.
The angles of the adjacent upper and lower articular surfaces of the FJ do not match perfectly on all 3 planes. The balance between mobility and stability of the lumbar segment is more in favor of the former than with the cervical and thoracic spine, and the imperfect matching of the articular surfaces helps to increase the range of motion of the FJ (22). In our study, we found that the range of motion of FJ was only highly correlated with independent articular surfaces, and not significantly correlated with the adjacent articular surfaces that comprise them.
In the present study, using DFIS and CT, we described the 3D orientation and asymmetry of the FJ and analyzed the data on the in vivo motion of FJ during sitting flexion and extension movements. From our experiments, it can be concluded that FT is more prevalent in the L4-L5 and L5-S1 segments than in L3-L4. The mobility of L5-S1 in the sagittal and coronal planes during seated flexion and extension was greater on the right side than on the left side. In the correlation analysis, the 3D orientation of the left FJ correlated highly with mobility. The more transverse facet angles in the L3-L4 and L5-S1 segments were biased toward the sagittal plane, the greater the range of mobility of the corresponding FJ in the coronal plane. The data analysis helps us to understand the physiological characteristics of FJ in the sitting position and the potential mechanism of lumbar spine disease occurrence, which provides a theoretical basis to guide clinical practice. The correlation between the 3D orientation and the spatial mobility of FJ also provides a new perspective for the study of joint kinematics. The orientation of the FJ in the sagittal and coronal planes could have a greater kinematic significance. The FJ mobility and the possibility of LBP can be better analyzed in sitting motion from the perspective of the 3D orientation of zygapophyseal joints. In addition, when there is a difference in the joints orientation between the two sides, appropriate adjustment of posture and motion during sitting may reduce the occurrence of FJ degeneration. Our study has several limitations. First, the sample size was small. Second, data were collected only for seated flexion and extension movements, lacking analysis of other positions in the seated position, such as lateral flexion and rotation. Third, only asymptomatic young participants were selected. Further analyses of 3D orientation and kinematic parameters of FJ in different age groups and with different diseases should be performed in the future.
Acknowledgments
Funding: None.
Footnote
Reporting Checklist: The authors have completed the MDAR reporting checklist. Available at https://atm.amegroups.com/article/view/10.21037/atm-22-969/rc
Data Sharing Statement: Available at https://atm.amegroups.com/article/view/10.21037/atm-22-969/dss
Conflicts of Interest: All authors have completed the ICMJE uniform disclosure form (available at https://atm.amegroups.com/article/view/10.21037/atm-22-969/coif). The authors have no conflicts of interest to declare.
Ethical Statement: The authors are accountable for all aspects of the work in ensuring that questions related to the accuracy or integrity of any part of the work are appropriately investigated and resolved. The study was conducted in accordance with the Declaration of Helsinki (as revised in 2013). The study was approved by the Research Ethics Committee of Tianjin Hospital of Tianjin University and informed consent was taken from all the patients.
Open Access Statement: This is an Open Access article distributed in accordance with the Creative Commons Attribution-NonCommercial-NoDerivs 4.0 International License (CC BY-NC-ND 4.0), which permits the non-commercial replication and distribution of the article with the strict proviso that no changes or edits are made and the original work is properly cited (including links to both the formal publication through the relevant DOI and the license). See: https://creativecommons.org/licenses/by-nc-nd/4.0/.
References
- Imai T, Nishiike S, Okumura T, et al. Effect of Sitting Position vs. Supine Position With the Head Turned to the Affected Side on Benign Paroxysmal Positional Vertigo Fatigue. Front Neurol 2021;12:705034. [Crossref] [PubMed]
- Czaprowski D, Gwiazdowska-Czubak K, Tyrakowski M, et al. Sagittal body alignment in a sitting position in children is not affected by the generalized joint hypermobility. Sci Rep 2021;11:13748. [Crossref] [PubMed]
- Ledet EH, Tymeson MP, DiRisio DJ, et al. Direct real-time measurement of in vivo forces in the lumbar spine. Spine J 2005;5:85-94. [Crossref] [PubMed]
- Spyropoulos P, Papathanasiou G, Georgoudis G, et al. Prevalence of low back pain in greek public office workers. Pain Physician 2007;10:651-9. [Crossref] [PubMed]
- Edwards J, Hayden J, Asbridge M, et al. The prevalence of low back pain in the emergency department: a descriptive study set in the Charles V. Keating Emergency and Trauma Centre, Halifax, Nova Scotia, Canada. BMC Musculoskelet Disord 2018;19:306. [Crossref] [PubMed]
- Ianuzzi A, Little JS, Chiu JB, et al. Human lumbar facet joint capsule strains: I. During physiological motions. Spine J 2004;4:141-52. [Crossref] [PubMed]
- Berry DB, Hernandez A, Onodera K, et al. Lumbar spine angles and intervertebral disc characteristics with end-range positions in three planes of motion in healthy people using upright MRI. J Biomech 2019;89:95-104. [Crossref] [PubMed]
- Shaikh N, Zhang H, Brown SHM, et al. The effect of posture on lumbar muscle morphometry from upright MRI. Eur Spine J 2020;29:2306-18. [Crossref] [PubMed]
- Crawford NR, Duggal N, Chamberlain RH, et al. Unilateral cervical facet dislocation: injury mechanism and biomechanical consequences. Spine (Phila Pa 1976) 2002;27:1858-64; discussion 1864. [Crossref] [PubMed]
- Zehr JD, Barrett JM, Fewster KM, et al. Strain of the facet joint capsule during rotation and translation range-of-motion tests: an in vitro porcine model as a human surrogate. Spine J 2020;20:475-87. [Crossref] [PubMed]
- Kong MH, He W, Tsai YD, et al. Relationship of facet tropism with degeneration and stability of functional spinal unit. Yonsei Med J 2009;50:624-9. [Crossref] [PubMed]
- Chowdhury SK, Byrne RM, Zhou Y, et al. Lumbar Facet Joint Kinematics and Load Effects During Dynamic Lifting. Hum Factors 2018;60:1130-45. [Crossref] [PubMed]
- Shin MH, Ryu KS, Hur JW, et al. Association of facet tropism and progressive facet arthrosis after lumbar total disc replacement using ProDisc-L. Eur Spine J 2013;22:1717-22. [Crossref] [PubMed]
- Karacan I, Aydin T, Sahin Z, et al. Facet angles in lumbar disc herniation: their relation to anthropometric features. Spine (Phila Pa 1976) 2004;29:1132-6. [Crossref] [PubMed]
- Rong X, Wang B, Ding C, et al. The biomechanical impact of facet tropism on the intervertebral disc and facet joints in the cervical spine. Spine J 2017;17:1926-31. [Crossref] [PubMed]
- Do DH, Taghavi CE, Fong W, et al. The relationship between degree of facet tropism and amount of dynamic disc bulge in lumbar spine of patients symptomatic for low back pain. Eur Spine J 2011;20:71-8. [Crossref] [PubMed]
- Mohanty SP, Pai Kanhangad M, Kamath S, et al. Morphometric study of the orientation of lumbar zygapophyseal joints in a South Indian population. J Orthop Surg (Hong Kong) 2017;25:2309499017739483. [Crossref] [PubMed]
- Zhou Q, Teng D, Zhang T, et al. Association of facet tropism and orientation with lumbar disc herniation in young patients. Neurol Sci 2018;39:841-6. [Crossref] [PubMed]
- Kozanek M, Wang S, Passias PG, et al. Range of motion and orientation of the lumbar facet joints in vivo. Spine (Phila Pa 1976) 2009;34:E689-96. [Crossref] [PubMed]
- Li G, Wang S, Passias P, et al. Segmental in vivo vertebral motion during functional human lumbar spine activities. Eur Spine J 2009;18:1013-21. [Crossref] [PubMed]
- Kim HJ, Chun HJ, Lee HM, et al. The biomechanical influence of the facet joint orientation and the facet tropism in the lumbar spine. Spine J 2013;13:1301-8. [Crossref] [PubMed]
- Masharawi Y, Rothschild B, Dar G, et al. Facet orientation in the thoracolumbar spine: three-dimensional anatomic and biomechanical analysis. Spine (Phila Pa 1976) 2004;29:1755-63. [Crossref] [PubMed]
- Ko S, Chae S, Choi W, et al. The Prevalence of Facet Tropism and Its Correlation with Low Back Pain in Selected Community-Based Populations. Clin Orthop Surg 2019;11:176-82. [Crossref] [PubMed]
- Song Y, Wen WQ, Xu J, et al. Kinematic Characteristics and Biomechanical Changes of Lower Lumbar Facet Joints Under Different Loads. Orthop Surg 2021;13:1047-54. [Crossref] [PubMed]
- Ahmed AM, Duncan NA, Burke DL. The effect of facet geometry on the axial torque-rotation response of lumbar motion segments. Spine (Phila Pa 1976) 1990;15:391-401. [Crossref] [PubMed]