Effects of metformin on pregnancy outcome, metabolic profile, and sex hormone levels in women with polycystic ovary syndrome and their offspring: a systematic review and meta-analysis
Introduction
Polycystic ovary syndrome (PCOS), which is often accompanied by insulin resistance (IR), is one of the most common hormonal disorders affecting the ovaries during childbearing years, with an estimated prevalence of 5% to 10% (1). Women with PCOS have difficulties conceiving naturally. Even when pregnancy is successfully achieved through medication, surgery, or in vitro fertilization, women with PCOS still experience many complications, such as gestational diabetes mellitus (GDM), miscarriage, pregnancy-induced hypertension (PIH) and preeclampsia, preterm birth, and intrauterine growth retardation or malformation (2,3).
Metformin can regulate the menstrual cycle and promote ovulation by improving IR and reducing hyperinsulinemia and weight loss, thereby increasing the pregnancy rate for patients with PCOS. At present, most guidelines for PCOS treatment recommend that metformin treatment should be implemented during the early period of pregnancy. However, studies have shown that metformin can enter the placenta through transport channels such as plasma membrane monoamine transporters and organic cation transporters, resulting in possible exposure of the fetus to the drug (4,5). Evidence for the efficacy, safety, and benefits of continual metformin use during the pregnancy period still remains conflicted, for example, some studies reported use of metformin is significantly associated with reduction in gestational diabetes among PCOS women (6-8), however, several other studies showed that metformin have no significant effect on gestational diabetes (1,9-11). Some studies found metformin had no effect on pregnancy induced hypertension (9,12), whereas others showed that metformin significantly reduced hypertension (8,13). Besides, whether metformin is associated with metabolic profile and sex hormone levels also remain a subject of debate. Recent published studies showed controversial results of fasting glucose and SHGB hormone levels (9,14-16). The small sample size of the studies might contribute to the various and inconsistent results, thus it is necessary to develop a meta-analysis to assess the efficacy of metformin.
Given the controversial results, we conducted the present systematic review and meta-analysis to compare the risks and benefits of metformin on pregnancy outcome, metabolic profile, and sex hormone levels of PCOS women and their children, with the expectation to give an evidence-based analysis for clinical practice. We present the following article in accordance with the PRISMA reporting checklist (17) (available at https://atm.amegroups.com/article/view/10.21037/atm-22-909/rc).
Methods
Search strategy and selection criteria
This protocol was registered on the PROSPERO platform (No. CRD42021266145).
We searched the China National Knowledge Infrastructure (CNKI), Wanfang Databases, PubMed, Embase, and Cochrane Library databases to identify human studies using the following keywords: (“metformin”) AND (“polycystic ovary syndrome” OR “PCOS”) AND (“outcomes” OR “complications” OR “effects”). The detailed search strategies were showed in Supplementary file (Table S1). Studies published from inception to July 10, 2021 were included.
Two researchers (DYZ and YC) independently assessed the articles for eligibility. Randomized controlled trials investigating the effects of continual treatment of metformin during pregnancy on maternal or offspring outcomes were included if they met the following inclusion criteria: (I) the study population were women who were pregnant and diagnosed with PCOS according to the Rotterdam 2003 criteria or offspring whose mother had been diagnosed with PCOS; (II) metformin was used as an intervention on participants during pregnancy; (III) the study included a placebo control group who were not treated with metformin during pregnancy; (IV) the study assessed outcomes relating to pregnancy complications, including PIH and preeclampsia, GDM, and preterm birth, or offspring outcomes, including birth weight, birth length, head circumference at birth, and body mass index (BMI) at longer follow-up; (V) the study was a published randomized controlled trial (RCT) study. We excluded conference abstracts, case reports, systematic reviews and meta-analysis, and studies without control groups or without available full text or data were excluded. The detailed information regarding to the specific inclusion and exclusion criteria and treatment intervention of each study were summarized in Table S2 and Table S3. When data appeared in more than 1 publication, only the dataset with the most comprehensive data was included.
Data extraction
Working independently, DYZ and YC used detailed information forms to extract the data and conduct the quality assessment. A third researcher (LZX) was responsible for addressing any unresolved disagreements between DYZ and YC concerning the extracted data or its quality. The data extraction included the following information: first author, year of publication, country where the study was conducted, study design, total sample size, survey time, mean or median age with standard deviation (SD) of pregnant women, mean or median BMI with SD of pregnant women, follow-up time, number of events or total participants in the exposure group and control group, mean or median of offspring weight, length, and BMI with SD.
We used the Cochrane Reviewer’s Handbook 5.0 to assess the risk of bias for all of the eligible articles. We evaluated the selection, performance, detection, attribution, reporting and other bias of RCT studies and clarified the risk of bias as “low”, “high” and “unclear”. We defined the articles with 7 low-risk items as “high quality”, the articles with 5 or more than 5 high-risk or unclear risk items as “low quality”, and the rest as “medium quality”.
Statistical analysis
We calculated the pooled risk ratio (RR) or mean difference, each accompanied by the 95% CI. To estimate risks for pregnancy outcomes, we divided the data into two comparative groups: participants treated with metformin versus participants without metformin treatment. We used an I2 statistic of ≥50% as an indicator of large statistical heterogeneity. If substantial heterogeneity was detected, we used a random-effects model to calculate pooled effect size and 95% CIs.
Subgroup analyses and meta-regression analyses were also performed to explore potential sources of heterogeneity. The subgroup analyses were categorized by the following variables: regional income level based on the World Bank standard (18), and quality of the study. Evidence for differences between the subgroups was quantified by the RRs or mean difference comparing associations in the subgroups and the corresponding P values for interaction. Studies that clearly provided essential information were included in corresponding subgroup analyses. A meta-regression analysis of the relationship between metformin treatment and pregnancy outcomes was conducted for each outcome with high heterogeneity.
We used funnel plots, Egger’s test, and Begger’s test to assess the possibility of publication bias and small-study effects in each result. In addition, sensitivity analyses were performed to determine the impact of individual studies on the pooled estimates by excluding each of the studies from the pooled estimate. All analyses used the “Meta-Analysis” statistical package in Stata 12 software (StataCorp LLC, College Station, TX, USA).
Results
We conducted the search on July 10, 2021. A total of 3,149 records were included in the initial search, and 2,837 remained after duplicates were removed. After excluding articles that did not meet the inclusion criteria, 48 studies were full-text screened, and finally 18 articles were determined to be eligible and included in this analysis (Figure 1) (1,9-12,14-16,19-28).
The characteristics of the included studies and their corresponding quality scores are reported in Table 1. These studies covered 6 countries (13 in Norway, and each in China, Egypt, Bangladesh, Finland and a multi-national study). Among the 18 eligible studies, a total of 2,461 women and 1,291 offspring were included in this meta-analysis. Regarding pregnancy complications, 11 studies were included for maternal prenatal outcomes, 4 studies for maternal metabolic profile, and 2 studies for maternal hormone levels. Regarding complications among offspring, 10 studies were included for prenatal outcomes, 2 studies for metabolic profile, and 2 studies for sex hormone levels. One study involved psychiatric and neurological complications among pregnant women and offspring.
Table 1
Author [publication year] | Nation | Sample size | Study design | Mean age (SD) in metformin group | Mean age (SD) in control group | Mean BMI (SD) in metformin group |
Mean BMI (SD) in control group | Follow-up duration | Main outcomes |
---|---|---|---|---|---|---|---|---|---|
Vanky E [2004] | Norway | 40 | RCT | 28.6±4.2 | – | 30.6±7.3 | – | During pregnancy | Severe pregnancy or post partum complications, preterm delivery |
Vanky E [2010] | Norway | 274 | RCT | 29.6±4.4 | 29.2±4.4 | 29.5±7.0 | 28.5±7.2 | During pregnancy | Preeclampsia, preterm delivery, gestational diabetes mellitus |
Underdal MO [2018] | Norway | 274 | Follow-up study of an RCT | 37.5±4.2 | – | 29.6±6.9 | – | 7.7 years after pregnancy | Type 2 diabetes mellitus, depression |
Salvesen KA [2007] | Norway | 40 | RCT | 28.9 (4.8) | 28.3 (3.7) | 32.1 (6.1) | 29.3 (8.0) | From 6–12 (mean: 8) gestational weeks to birth | Pregnancy complications, cesarean section, persistent nausea and gastrointestinal discomfort, preterm delivery, preeclampsia, postpartum streptococcus sepsis |
Løvvik TS [2019] | Norway, Sweden, and Iceland | 468 | RCT | 29 (24–34) | 30 (24–36) | 27.5 (18.2–36.5) | 26.7 (18.8–34.6) | During pregnancy | Miscarriage, GDM, preterm delivery, PIH |
Hjorth-Hansen A [2018] | Norway | 258 | RCT | 29.6 (4.3) | 29.3 (4.4) | 29.6 (7.3) | 28.2 (6.4) | During pregnancy | Birth weight, birth length |
Greger HK [2020] | Norway | 93 | Follow-up of 2 randomized, placebo-controlled trials | 29.3 (3.4) | 29.0 (4.3) | 30.2 (7.0) | 27.9 (6.3) | 7.7 years | GDM, preeclampsia, preterm delivery |
Fougne KJ [2008] | Norway | 40 | RCT | 28.9 (26.5–31.4) | 28.3 (26.6–30.0) | 29.3 (25.8–32.9) | 32.1 (29.1–35.2) | During pregnancy | GDM |
Carlsen SM [2012] | Norway | 199 | Follow-up study of RCT | 29.7 (4.4) | 29.4 (4.3) | 29.5 (7.1) | 27.6 (6.1) | 1 year after birth | 1-year postpartum BMI |
Carlsen SM [2010] | Norway | 40 | Follow-up study of RCT | – | – | – | – | During pregnancy | SHBG levels, dihydrotestosterone |
Begum MR [2009] | Bangladesh | 59 | RCT | 28.14 (2.92) | 26.13 (3.62) | 28.21 (2.37) | 27.97 (2.49) | During pregnancy | GDM, miscarriage, preterm labor |
Abdalmagee OS [2019] | Egypt | 102 | RCT | 31.1±3.7 | 31.89±3.6 | 33.8±4.4 | 32.9±6.7 | During pregnancy | Miscarriage |
Wang Aiping [2018] | China | 72 | RCT | 30.8±3.0 | 30.0±2.7 | – | – | During pregnancy | Fasting glucose, insulin, HbA1c (%) |
Rø TB [2012] | Norway | 25 | Follow-up study of an RCT | 8.2 (0.6) | 8.1 (0.8) | 1.09 (0.91) | 1.05 (1.06) | 8 years after birth | Birth weight, BMI (SDS) at 8 years old |
Morin-Papunen L [2012] | Finland | 135 | RCT | 28.4 (3.9) | 27.9 (4.1) | 27.1 (6.3) | 26.9 (6.2) | During pregnancy | Live birth |
Hanem LGE [2019] | Norway | 139 | Follow-up of randomized, placebo-controlled trials | 29.5±3.9 | 30.2±4.2 | 29.0±6.9 | 28.4±6.3 | 4 years after birth | BMI at 4 years, waist circumference, body fat |
Hanem LGE [2018] | Norway | 141 | Follow-up of randomized, placebo-controlled trials | – | – | 17.8 (17.0 to 18.5) | 16.8 (16.2 to 17.3) | 5–10 years after birth | Birth length, birth weight |
Hanem LGE [2021] | Norway | 62 | Follow-up of randomized, placebo-controlled trials | 7.40 ± 1.21 | 7.46 ± 1.18 | – | – | 5–10 years after birth | Androstenedione, 11-deoxycortisol |
BMI, body mass index; GDM, gestational diabetes mellitus, RCT, randomized controlled trial; BMI SDS, standardized BMI.
The effect of continual use of metformin on pregnancy outcomes
Using a random-effects model, the meta-analysis showed that continual use of metformin during pregnancy can significantly reduce the occurrence of PIH and preeclampsia (RR =0.45, 95% CI: 0.24–0.83, I2=12%, P=0.33); preterm delivery (RR =0.37, 95% CI: 0.23–0.61, I2=0%, P=0.52); and macrosomia (RR =0.26, 95% CI: 0.11–0.64, I2=0%, P=0.83; Figure 2). However, it has no significant efficacy on improving the live birth rate (RR =1.20, 95% CI: 0.98–1.47, I2=2%, P=0.36); or decrease the risk of GDM or miscarriage compared with a placebo, with a pooled RR of 0.86 (95% CI: 0.67–1.12, I2=35%, P=0.19) and (RR =0.53, 95% CI: 0.24–1.21, I2=0%, P=0.74; Figure 2), respectively. With respect to other complications, such as persistent nausea and gastrointestinal discomfort, a study conducted by Salvesen (12) reported no difference between the metformin and placebo groups.
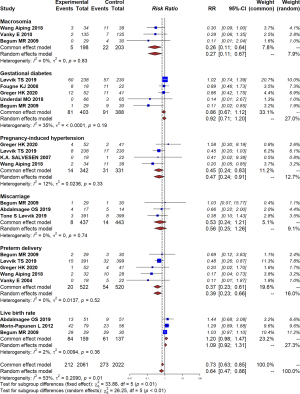
With respect to offspring, we found that intrauterine exposure of metformin was significantly associated with increased risk of larger head circumference at birth (MD =0.29, 95% CI: 0.13–0.45, I2=56%, P=0.11) and higher BMI measures in the long term (MD =0.37, 95% CI: 0.17–0.56, I2=0%, P=0.82). Despite these differences, no difference was detected in other offspring outcomes, including birth weight (MD =−0.08, 95% CI: −0.27 to 0.12, I2=51%, P=0.04) or birth length (MD =0.08, 95% CI: −0.09 to 0.24, I2=0%, P=0.87; Figure 3). Additionally, no significant difference was found in birth asphyxia (RR =0.62, 95% CI: 0.28–1.36, I2=54%, P=0.14), hypoglycemia (RR =0.59, 95% CI: 0.20–1.75, I2=71%, P=0.06), or malformation (RR =1.57, 95% CI: 0.26–9.61, I2=26%, P=0.25; Figure 4).
Regarding long term cognitive function, a follow-up study of 7.7 years by Greger (10) found no statistically significant difference in IQ scores between metformin and placebo. Moreover, no significant difference was detected in verbal comprehension, working memory, perceptual organization, or processing speed.
The effect of metformin on metabolic profile and sex hormone levels
Our study found that metformin could significantly decrease homeostatic model assessment for insulin resistance (HOMA-IR) scores (MD =−0.32, 95% CI: −0.63 to −0.02, I2=0%, P=0.62) in maternal women, although no effect on fasting glucose (MD =0.10, 95% CI: −0.14 to 0.35, I2=63%, P=0.06) or glycated hemoglobin (HbA1c; MD =−0.20, 95% CI: −0.47 to 0.07, I2=71%, P=0.06) was detected (Figure 5). In addition, levels of androstenedione, dehydroepiandrosterone sulfate (DHEAS), sex hormone binding globulin (SHBG), and testosterone were similar in the maternal blood of the metformin group and the placebo group (Figure 6).
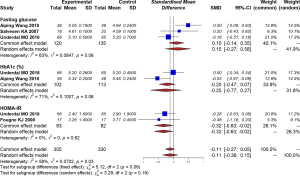
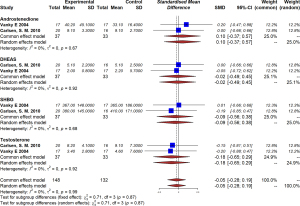
In the metformin group, offspring of mothers with PCOS had significantly higher SHGB levels in their blood (MD =0.33, 95% CI: 0.01–0.65, I2=69%, P=0.07). There was a tendency towards higher androstenedione (MD =0.02, 95% CI: −0.30 to 0.34), testosterone (MD =0.13, −0.19 to 0.45), and 11-deoxycortisol (MD =0.27, 95% CI: −0.10 to 0.63), but the difference was not statistically significant (Figure 7).
A study by Rø et al. (25) reported higher systolic blood pressure in the metformin group at a follow-up of 8 years (106 vs. 101 mmHg), with a borderline significance of P=0.05. Our study found no significant differences in the metabolic health profile of offspring, including fasting glucose, insulin, c-peptide, or HOMA2-IR (Figure 8).
Methodological assessment
The majority of the studies were in medium quality, while 4 of the studies were in low quality. Sixteen (88.9%) studies were in low risk in randomization sequence generation, while 2 studies were in high risk because they didn’t provide appropriate methods of randomization. Fourteen (77.8%) studies reported appropriate methods of allocation concealment, while the rest 4 studies didn’t report it. Thirteen (72.2%) studies provided the method of blinding for the participants, while no studies reported blinding of outcome assessment. Eight (45.4%) studies reported incomplete outcome data and in a high risk, the remaining studies were in low risk. There were only 3 (16.7%) studies in low risk of selective reporting of outcomes and none of the studies reported information of other bias. The assessment of risk of bias for the included trials was showed in Figures S1,S2.
Subgroup analysis and meta-regression
To further investigate influential factors with results, we conducted subgroup analyses according to study location and quality level. The results showed that the effect of metformin on GDM could be influenced by the study region. Metformin significantly reduced the risk of GDM in Middle or low income country compared with high income country, with RRs of 0.11 (95% CI: 0.02–0.85) and 0.94 (95% CI: 0.72–1.23, I2=0%, P=0.59), respectively (P=0.04 for subgroup differences). Birth weight was found to be influenced by both study region and study quality. We found that metformin had a significant efficacy on studies conducted in Middle or low income country and studies with low levels of quality (P<0.01 for both subgroup differences). In addition, no difference was found in the regional or quality subgroup analysis concerning preterm delivery (Figures S3-S8). The meta-regression analysis found no effect of either age or BMI on the effect of metformin (P=0.2949 and P=0.2431).
Publication bias and quality control
The leave-one-out sensitivity analysis showed consistency on all outcomes. Either Egger’s test or Begger’s test showed no publication bias for either pregnancy or offspring outcomes (P>0.05) (Figures S9-S19; Table S4).
Discussion
To our knowledge, this study is the largest and most wide-ranging systematic review and meta-analysis comprehensively summarizing current evidence on the possible effect of metformin treatment on maternal and offspring pregnancy outcome, metabolic health, and sex hormone levels in women with PCOS and their offspring. Our results showed that metformin treatment during pregnancy could reduce the risk of pregnancy complications, including PIH, macrosomia and preterm delivery. In addition, we found that metformin ameliorated IR in women with PCOS and increased SHBG levels in offspring. Regarding negative impacts, our results showed that metformin might increase the risk of larger head circumference at birth and lead to higher BMI in the long-term.
Individuals with PCOS have an increased risk of developing maternal complications involving GDM, PIH, and miscarriage (29,30). Previous studies have reported that PCOS is associated with severe pregnancy complications, including a threefold increased risk of GDM, a threefold to fourfold increased risk of PIH and preeclampsia, and a twofold increased risk of preterm delivery (28,29,31). In addition, approximately 30% to 50% of patients experience miscarriage (32). Our study demonstrated that metformin treatment decreased the risk of PIH and preeclampsia, and preterm delivery by 55% and 63%, respectively, when compared with the placebo group. Controversy remains regarding the effect of metformin on pregnancy outcome. In 2012, Morin-Papunen et al. (24) reported an insignificant difference between the metformin and the placebo group. In 2010, a randomized, placebo-controlled, double-blind, multicenter trial conducted by Vanky et al. investigating 257 women with PCOS found that metformin treatment from first trimester to delivery did not reduce pregnancy complications (including preeclampsia and preterm delivery) in patients with PCOS (28). However, our findings are consistent with a number of high-quality studies. In 2019, a multicenter, randomized, controlled trial performed at 14 hospitals revealed that metformin treatment from the late first trimester until delivery reduced the risk of late miscarriage and preterm birth but did not prevent GDM (11). Additionally, our findings are consistent with another meta-analysis which reported that metformin treatment throughout pregnancy can increase the success possibility of full-term delivery and decrease the risk of preterm labor and pregnancy complications, such as PIH, with no serious side effects.
In terms of offspring, the effect of metformin treatment throughout the pregnancy period remains unknown due to the limited number of studies addressing the issue. Our meta-analysis found that metformin significantly decreased the risk of macrosomia but was associated with significantly increased fetal head circumference. However, there was no evidence that fetal birth weight or length were associated with metformin treatment. Our results showed that metformin had a significant impact on children’s long-term growth as measured by BMI, which indicated that metformin-exposed children tended to be larger than placebo-exposed children. This finding is consistent with the 5–10 years follow-up of the PregMet RCT conducted by Hanem et al., which found that metformin treatment in PCOS pregnancies led to children with a higher mean BMI accompanied by central adiposity and an increased prevalence of obesity at 5–10 years of age (9). Another study following the offspring of women with GDM found that metformin-exposed offspring were larger by measures of weight, arm and waist circumference, and BMI at 9 years compared with offspring exposed to insulin treatment during pregnancy (29). Although our study found no difference in the birth weight of offspring, children exposed to metformin appeared to have a higher risk of obesity in childhood.
With respect to metabolic profile, our study found that metformin could help modulate IR in pregnant women with PCOS. A previous study revealed that metformin could reduce plasma insulin levels by promoting weight loss and improving the metabolic health of women (30). Although animal models have suggested that metformin might contribute to improvements in IR in female offspring, the effect of metformin on the offspring of patients with PCOS remains uncertain. A follow-up study of children from the PregMet study found no difference in HOMA2IR scores in offspring between the groups (9). Our results are consistent with these studies; although metformin appeared to improve the HOMA2IR scores in offspring, no significant difference was detected.
We also investigated the effect of metformin on sex hormone levels. Our study found that SHGB levels were significantly increased in offspring of women with PCOS; however, no similar trend was found in mothers. The underlying mechanism might be related to the improvement of the metabolic health of mothers. A previous study has shown that IR can cause decreased SHBG, so taking metformin during pregnancy might impact SHBG by ameliorating HOMA2IR levels (33). Regarding androgen and testosterone levels, we found that neither was affected by metformin treatment, which is consistent with previous study (14). Although no significant difference in maternal hormone levels was found in this study, the correlation between maternal and offspring hormone levels should be investigated in the future.
This meta-analysis has several limitations. First, there are a limited number of studies addressing the relevant outcomes, especially regarding long-term metabolic profile and sex hormone levels, and so our results should be interpreted cautiously. Second, the qualities of eligible studies were not high. Therefore, a higher quality study with a larger sample size should be established to further investigate the inconsistent situation. Third, although we found no significant difference in maternal sex hormone levels between groups, the impact of maternal sex hormones on the sex hormones of offspring should be further studied in the future.
In conclusion, although the relative low quality of RCTs and limited results made it difficult to draw a definite conclusion, our study showed that metformin treatment during pregnancy can reduce the risk of pregnancy complications but may have impacts on SHBG levels and long-term BMI in offspring.
Acknowledgments
Funding: This study was supported by a grant from the National Natural Science Foundation of China (No. 81971354).
Footnote
Reporting Checklist: The authors have completed the PRISMA reporting checklist. Available at https://atm.amegroups.com/article/view/10.21037/atm-22-909/rc
Conflicts of Interest: All authors have completed the ICMJE uniform disclosure form (available at https://atm.amegroups.com/article/view/10.21037/atm-22-909/coif). The authors have no conflicts of interest to declare.
Ethical Statement: The authors are accountable for all aspects of the work in ensuring that questions related to the accuracy or integrity of any part of the work are appropriately investigated and resolved.
Open Access Statement: This is an Open Access article distributed in accordance with the Creative Commons Attribution-NonCommercial-NoDerivs 4.0 International License (CC BY-NC-ND 4.0), which permits the non-commercial replication and distribution of the article with the strict proviso that no changes or edits are made and the original work is properly cited (including links to both the formal publication through the relevant DOI and the license). See: https://creativecommons.org/licenses/by-nc-nd/4.0/.
References
- Fougner KJ, Vanky E, Carlsen SM. Metformin has no major effects on glucose homeostasis in pregnant women with PCOS: results of a randomized double-blind study. Scand J Clin Lab Invest 2008;68:771-6. [Crossref] [PubMed]
- Roos N, Kieler H, Sahlin L, et al. Risk of adverse pregnancy outcomes in women with polycystic ovary syndrome: population based cohort study. BMJ 2011;343:d6309. [Crossref] [PubMed]
- Gadalla MA, Norman RJ, Tay CT, et al. Medical and Surgical Treatment of Reproductive Outcomes in Polycystic Ovary Syndrome: An Overview of Systematic Reviews. Int J Fertil Steril 2020;13:257-70. [PubMed]
- Rojas J, Chávez-Castillo M, Bermúdez V. The Role of Metformin in Metabolic Disturbances during Pregnancy: Polycystic Ovary Syndrome and Gestational Diabetes Mellitus. Int J Reprod Med 2014;2014:797681. [Crossref] [PubMed]
- Lindsay RS, Loeken MR. Metformin use in pregnancy: promises and uncertainties. Diabetologia 2017;60:1612-9. [Crossref] [PubMed]
- Glueck CJ, Wang P, Kobayashi S, et al. Metformin therapy throughout pregnancy reduces the development of gestational diabetes in women with polycystic ovary syndrome. Fertil Steril 2002;77:520-5. [Crossref] [PubMed]
- Khattab S, Mohsen IA, Foutouh IA, et al. Metformin reduces abortion in pregnant women with polycystic ovary syndrome. Gynecol Endocrinol 2006;22:680-4. [Crossref] [PubMed]
- Nawaz FH, Rizvi J. Continuation of metformin reduces early pregnancy loss in obese Pakistani women with polycystic ovarian syndrome. Gynecol Obstet Invest 2010;69:184-9. [Crossref] [PubMed]
- Hanem LGE, Salvesen Ø, Juliusson PB, et al. Intrauterine metformin exposure and offspring cardiometabolic risk factors (PedMet study): a 5-10 year follow-up of the PregMet randomised controlled trial. Lancet Child Adolesc Health 2019;3:166-74. [Crossref] [PubMed]
- Greger HK, Hanem LGE, Østgård HF, et al. Cognitive function in metformin exposed children, born to mothers with PCOS - follow-up of an RCT. BMC Pediatr 2020;20:60. [Crossref] [PubMed]
- Løvvik TS, Carlsen SM, Salvesen Ø, et al. Use of metformin to treat pregnant women with polycystic ovary syndrome (PregMet2): a randomised, double-blind, placebo-controlled trial. Lancet Diabetes Endocrinol 2019;7:256-66. [Crossref] [PubMed]
- Salvesen KA, Vanky E, Carlsen SM. Metformin treatment in pregnant women with polycystic ovary syndrome--is reduced complication rate mediated by changes in the uteroplacental circulation? Ultrasound Obstet Gynecol 2007;29:433-7. [Crossref] [PubMed]
- Khattab S, Mohsen IA, Aboul Foutouh I, et al. Can metformin reduce the incidence of gestational diabetes mellitus in pregnant women with polycystic ovary syndrome? Prospective cohort study. Gynecol Endocrinol 2011;27:789-93. [Crossref] [PubMed]
- Carlsen SM, Vanky E. Metformin influence on hormone levels at birth, in PCOS mothers and their newborns. Hum Reprod 2010;25:786-90. [Crossref] [PubMed]
- Hanem LGE, Salvesen Ø, Madsen A, et al. Maternal PCOS status and metformin in pregnancy: Steroid hormones in 5-10 years old children from the PregMet randomized controlled study. PLoS One 2021;16:e0257186. [Crossref] [PubMed]
- Aiping. Wang. The effect of metformin on mother and child in pregnancy with polycycstic ovary syndrome combined with type 2 diabetes mellitus. Jilin University, MA thesis. 2018.
- Moher D, Liberati A, Tetzlaff J, et al. Preferred reporting items for systematic reviews and meta-analyses: the PRISMA statement. PLoS Med 2009;6:e1000097. [Crossref] [PubMed]
- The world bank.World bank country and lending groups. January 30, 2021.; available from: https://datahelpdesk.worldbank.org/knowledgebase/articles/906519-world-bank-country-and-lending-groups.
- Abdalmageed OS, Farghaly TA, Abdelaleem AA, et al. Impact of Metformin on IVF Outcomes in Overweight and Obese Women With Polycystic Ovary Syndrome: A Randomized Double-Blind Controlled Trial. Reprod Sci 2019;26:1336-42. [Crossref] [PubMed]
- Begum MR, Khanam NN, Quadir E, et al. Prevention of gestational diabetes mellitus by continuing metformin therapy throughout pregnancy in women with polycystic ovary syndrome. J Obstet Gynaecol Res 2009;35:282-6. [Crossref] [PubMed]
- Carlsen SM, Martinussen MP, Vanky E. Metformin’s effect on first-year weight gain: a follow-up study. Pediatrics. 2012;130:e1222-6. [Crossref] [PubMed]
- Hanem LGE, Stridsklev S, Júlíusson PB, et al. Metformin Use in PCOS Pregnancies Increases the Risk of Offspring Overweight at 4 Years of Age: Follow-Up of Two RCTs. J Clin Endocrinol Metab 2018;103:1612-21. [Crossref] [PubMed]
- Hjorth-Hansen A, Salvesen Ø, Engen Hanem LG, et al. Fetal Growth and Birth Anthropometrics in Metformin-Exposed Offspring Born to Mothers With PCOS. J Clin Endocrinol Metab 2018;103:740-7. [Crossref] [PubMed]
- Morin-Papunen L, Rantala AS, Unkila-Kallio L, et al. Metformin improves pregnancy and live-birth rates in women with polycystic ovary syndrome (PCOS): a multicenter, double-blind, placebo-controlled randomized trial. J Clin Endocrinol Metab 2012;97:1492-500. [Crossref] [PubMed]
- Rø TB, Ludvigsen HV, Carlsen SM, et al. Growth, body composition and metabolic profile of 8-year-old children exposed to metformin in utero. Scand J Clin Lab Invest 2012;72:570-5. [Crossref] [PubMed]
- Underdal MO, Stridsklev S, Oppen IH, et al. Does Metformin Treatment During Pregnancy Modify the Future Metabolic Profile in Women With PCOS? J Clin Endocrinol Metab 2018;103:2408-13. [Crossref] [PubMed]
- Vanky E, Salvesen KA, Heimstad R, et al. Metformin reduces pregnancy complications without affecting androgen levels in pregnant polycystic ovary syndrome women: results of a randomized study. Hum Reprod 2004;19:1734-40. [Crossref] [PubMed]
- Vanky E, Stridsklev S, Heimstad R, et al. Metformin versus placebo from first trimester to delivery in polycystic ovary syndrome: a randomized, controlled multicenter study. J Clin Endocrinol Metab 2010;95:E448-55. [Crossref] [PubMed]
- Rowan JA, Rush EC, Plank LD, et al. Metformin in gestational diabetes: the offspring follow-up (MiG TOFU): body composition and metabolic outcomes at 7-9 years of age. BMJ Open Diabetes Res Care 2018;6:e000456. [Crossref] [PubMed]
- Jorquera G, Echiburú B, Crisosto N, et al. Metformin during Pregnancy: Effects on Offspring Development and Metabolic Function. Front Pharmacol 2020;11:653. [Crossref] [PubMed]
- Sha T, Wang X, Cheng W, et al. A meta-analysis of pregnancy-related outcomes and complications in women with polycystic ovary syndrome undergoing IVF. Reprod Biomed Online 2019;39:281-93. [Crossref] [PubMed]
- Kamalanathan S, Sahoo JP, Sathyapalan T. Pregnancy in polycystic ovary syndrome. Indian J Endocrinol Metab 2013;17:37-43. [Crossref] [PubMed]
- Kajaia N, Binder H, Dittrich R, et al. Low sex hormone-binding globulin as a predictive marker for insulin resistance in women with hyperandrogenic syndrome. Eur J Endocrinol 2007;157:499-507. [Crossref] [PubMed]
(English Language Editor: C. Gourlay)