Identification of immune-related biomarkers in embryos with neural tube defects via a bioinformatics analysis
Introduction
Neural tube defects (NTDs) are congenital disorders in which the neural tube does not close during the embryonic period (1). The prevalence of NTDs varies around the world, but with an incidence of about 0.1% in Western countries, NTDs are one of the most common types of birth defects (2,3). The etiology of NTDs is unknown; however, research has confirmed the involvement of genetic and environmental factors (4). Indeed, approximately 70% of the genes variation has been attributed to genetic factors (4). The use of certain medications during pregnancy, obesity, poorly controlled diabetes, and folic acid (FA) deficiency may contribute to the development of NTDs (4). The disturbance of any sequential event during embryonic neurogenesis may also cause NTDs (5). The area of the neural tube that remains open is an important factor in determining the phenotype (e.g., anencephaly or spina bifida). Research has shown that mutations in >200 genes can lead to NTDs in mice, but the pattern of occurrence in humans suggests multifactorial polygenic or oligogenic causes (5). This emphasizes the importance of gene-gene and gene-environment interactions in the origins of these defects.
Epidemiological data suggest that most NTDs occur as a result of complex interactions between the environment and various susceptibility genetic factors (6). The current study indicated that the closure of the mammalian neural tube is intermittently initiated and fused at 4 discrete sites, and the disruption of this process at any 1 of these sites may lead to NTDs. Genes of the folate metabolic pathway and genes involved in folate transport that participate in the neural tube closure are candidate biomarkers (6). Karzbrun et al. found that the width of the nerve tissue determines the shape of the neural tube, and used a chip-based culture system to reconstruct a neural tube in a petri dish that was about 90% similar to that of humans (7). Wolujewicz et al. showed that genetic variation in the genome is an important factor affecting in NTDs (8). Despite the strengthening of FA treatments in the last 20 years, the incidence of NTDs remains unacceptably high. However, we know very little about the mechanism of FA in NTDs. Further, even after surgical repair, the extensive nervous system remains a problem in patients with NTDs. Thus, neglected NTDs remain a significant public health burden (9,10).
Numerous studies have shown that the immune system has the potential to directly affect key morphological regulatory steps in neurogenesis and formation (11-13). For example, Tissir et al. demonstrated that chemokine receptors may have trophic effects on precursor cell proliferation and some neuronal targets (12). While McLin et al. found that the expression of complement components is already extensive during the gastrula/early neurula stage (13). However, as few studies have examined immune genes and their related differences in human NTDs, we conducted this study. First, we compared the differentially expressed genes (DEGs) between NTD and healthy embryos and identified the immune-related genes of NTDs by intersecting these genes with the immune-related genes. We also investigated the differences in immune cell infiltration between NTD and healthy embryos, and the correlations among immune-related gene expression levels and immune cell infiltration in NTDs to identify novel diagnostic and therapeutic biomarkers for NTDs. We present the following article in accordance with the STREGA reporting checklist (available at https://atm.amegroups.com/article/view/10.21037/atm-22-1273/rc).
Methods
Raw data
We downloaded the GSE33111 data set from Gene Expression Omnibus (GEO) database, which contained the messenger ribonucleic acid profiles of 12 NTD-affected fetal samples and 12 healthy control samples in the same period of fetal development. We also downloaded a list of immune-related genes from ImmPort’s shared data (https://www.immport.org/shared/home).
The study was conducted in accordance with the Declaration of Helsinki (as revised in 2013).
The immune-related DEGs of NTDs
We compared the healthy samples to the NTD samples to identify the DEGs. The filtering criteria were as follows: log2 fold change >0.5 and a false discovery rate <0.05. Next, the immune-related DEGs of the NTDs were extracted by identifying the DEGs that intersected with those on the immune-related genes list.
Functional enrichment analysis of DEGs
We used clusterProfiler package in R to conduct the Gene Ontology (GO) and Kyoto Encyclopedia of Genes and Genomes (KEGG) functional enrichment analysis, and used the ggplot2 package to visualize the results.
The protein-protein interaction (PPI) network of DEGs
The PPI network was constructed and visualized using STRING (https://string-db.org/) and Cytoscape software. We extracted the top 10 ranked genes using a number of adjacent nodes as hub biomarkers for the subsequent analysis.
Accuracy of immune-related DEG expression levels in the diagnosis of NTDs according to the ROC curve analysis
We investigated the expression levels of immune-related genes in healthy and NTDs embryos. We drew the receiver operating characteristic (ROC) curves of the expression levels of the immune-related genes in the NTDs samples via the “pROC” package.
Immune cell infiltration analysis comparing the healthy and NTDs samples
We compared the immune infiltration of the healthy and NTD samples via a single-sample gene set enrichment analysis (ssGSEA) method. Further, we analyzed the relationship between the top 10 ranked PPI immune-related genes and immune cell infiltration.
Statistical analysis
R software was used for all data analysis in present study. Differential analysis of healthy and NTD embryos was performed using the limma package. Wilcoxon non-parametric test and Spearman’s method were used to compare the differences and correlation between the two groups. P<0.05 was considered statistically significant.
Results
Immune-related DEGs in NTDs
Compared to the healthy samples, 403 genes were upregulated and 208 genes were downregulated in the NTD samples (see Figure 1A,1B). A total of 95 immune-related DEGs in the NTD samples were extracted from the intersection of the DEGs and immune-related genes list (see Figure 1C).
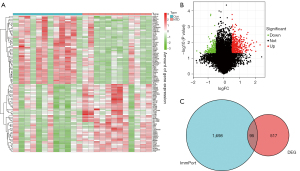
The GO and KEGG analysis of the NTDs related to DEGs
The results of the GO function analysis of the NTDs were as follows: the top 5 biological processes were the response to interferon-gamma, the cellular response to interferon-gamma, the interferon-gamma-mediated signaling pathway, antigen processing and the presentation of exogenous antigens, antigen processing and presentation; the top 5 cellular components were the major histocompatibility complex (MHC) protein complex, the integral component of the lumenal side of the endoplasmic reticulum membrane, the lumenal side of the endoplasmic reticulum membrane, the lumenal side of the membrane, and the MHC class II protein complex; and the top 5 molecular functions were the receptor ligand activity, signaling receptor activator activity, cytokine receptor binding, immune receptor activity, and peptide antigen binding (see Figure 2A,2B).
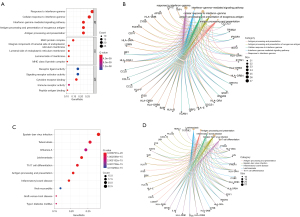
The results of the KEGG analysis showed that the top 10 enrichment KEGG pathways were Epstein-Barr virus infection, tuberculosis, influenza A, leishmaniasis, Th17 cell differentiation, antigen processing and presentation, inflammatory bowel disease, viral myocarditis, graft-versus-host disease, and type I diabetes mellitus (see Figure 2C,2D).
Top 10 ranked proteins of the PPI network of the NTD immune-related DEGs
The PPI network analysis of the NTD immune-related DEGs indicated that the top 10 ranked proteins were beta-2-microglobulin (B2M), C-C motif chemokine ligand 2 (CCL2), colony stimulating factor 1 receptor (CSF1R), C-X-C motif chemokine ligand 10 (CXCL10), interleukin 1 beta (IL1B), interferon regulatory factor (IRF1), Jun proto-oncogene, AP-1 transcription factor subunit (JUN), signal transducer and activator of transcription 1 (STAT1), STAT3, and transmembrane immune signaling adaptor transmembrane immune signaling adaptor (TYROBP) (see Figure 3). These 10 biomarkers were used as the hub genes for the subsequent analysis.
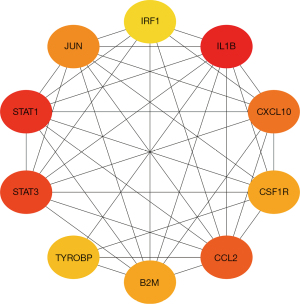
Expression level of hub immune-related DEGs in healthy and NTD samples
B2M, CCL2, CSF1R, CXCL10, IL1B, IRF1, JUN, STAT1, STAT3, and TYROBP were more highly expressed in the NTD samples than the healthy samples, (see Figure 4A-4J).
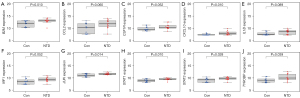
Accuracy of the single-gene expression levels in the diagnosis of NTDs (ROC curves)
At the single-gene expression level, the area under the curve (AUC) values of the ROC curves for the diagnosis of NTDs were as follows: B2M =0.812, CCL2 =0.729, CSF1R =0.736, CXCL10 =0.806, IL1B =0.708, IRF1 =0.736, JUN =0.792, STAT1 =0.806, STAT3 =0.764, and TYROBP =0.750 (see Figure 5A-5J).
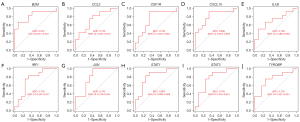
SsGSEA analysis of immune infiltration in NTDs
Of the 28 immune cell types, 20 were more highly infiltrated in the NTD samples than the healthy samples, including activated CD8 T cells, central memory CD8 T cells, effector memory CD8 T cells, activated CD4 T cells, and central memory CD4 T cells (see Figure 6A). The correlation analysis indicated that in the 10 hub biomarkers, the expression levels of CXCL10, TYROBP, IRF1, and CCL2 were positively correlated with the infiltration levels of most immune cells in NTDs (see Figure 6B).
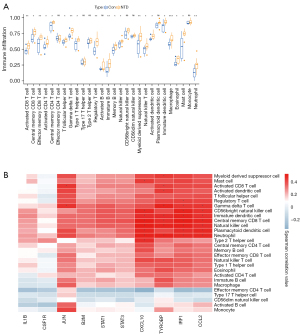
Discussion
NTDs are multi-factor diseases caused by a complex combination of and interactions between genes and the environment. The pathogenesis of NTDs remains unclear, but there have been 2 major advances in the prevention and treatment of NTDs (9,14,15). First, research has shown that taking FA at a childbearing age significantly reduces the risk of NTDs (16). Second, research has shown that the surgical repair of spina bifida in uterus can improve the prognosis of NTDs (17). However, not all NTDs can be prevented by taking FA, and as the incidence of NTDs remains about 0.1% in Western countries, consideration needs to be given to alternative therapies to treat the NTDs that do not respond to FA (2,18). Additionally, there is growing evidence that components of the immune system may directly affect key morpho-regulatory steps in neurogenesis (11). As for the possible pathogenesis of NTDs, Mallela et al. showed that taking sodium valproate in the first trimester of pregnancy may alter a variety of biological processes including apoptosis, thus inducing fetal malformation (19). Dong et al. experimentally verified five complement proteins (C1QA, C1S, C1R, C9 and C3), and concluded that the disease prediction model combining complement factor and AFP data could be a more accurate method for noninvasive prenatal NTD diagnosis (20).Thus, we conducted this study to analyze the correlations among immune genes and NTDs and to investigate the feasibility of diagnosing NTDs by detecting the expression levels of the hub immune genes to identify novel prevention strategies and reduce NTDs rates at birth.
In the present study, 611 DEGs were identified in the NTDs samples, and a total of 95 immune-related DEGs were extracted from the intersection of the DEGs and genes in the immune-related gene list A functional enrichment analysis of the immune-related DEGs showed that the NTDs were correlated with diabetes, inflammatory bowel disease, tuberculosis, influenza A, and certain signaling pathways. Our findings are similar to those of previous research; for example, Wani et al. showed that FA status was damaged in many pathophysiological conditions, including inflammatory bowel disease, cancer, alcoholism, pregnancy, and neonatal growth, and via the use of certain drugs, which led to the occurrence of NTDs (21). Additionally, Zabihi et al. showed that pregnant women with gestational diabetes (types 1 or 2) are at increased risk of a wide range of birth defects, including NTDs (22). In addition to interferon signaling, immune-related genes may have individual or multiple effects on NTDs (22). Further, Jensen et al. showed that maternal polymorphism in the CCL2 promoter region is associated with an increased risk of spina bifida in humans (23). CCL2 is a member of the CC chemokine subfamily that that induces the chemotaxis of monocytes and basophils by interacting with C-C Motif Chemokine Receptor 2 and C-C Motif Chemokine Receptor 4 receptors (24).
Other studies have shown that a FA deficiency can induce the synthesis of CCL2 in vitro and in vivo (25-27). The results of the present study indicated that CCL2 was more highly expressed in the NTDs samples than healthy samples, which suggested that CCL2 may be related to the occurrence and development of NTDs. Additionally, in the present study, the hub immune-related genes were highly expressed in the NTDs samples. Notably, most of the genes examined in this study have not been studied before. Our results also indicated that NTDs can be diagnosed with high accuracy based on the expression levels of certain hub immune-related genes (AUC range, 0.708–0.812). Thus, we can be measured by measuring the study the expression of key genes to predict the possibility of NTDs happen. A number of genes (e.g., B2M, CXCL10, IL1B, and STAT1/3) have great research prospects in the diagnosis and treatment of human NTDs. However, there is still a lack of corresponding methods and measures in the treatment and prevention of NTDs through these genes.
Given that immune cells play an important role in inflammatory processes, we were interested in studying immune cell infiltration in NTDs. Our results suggest that immune-related genes are significantly correlated with the infiltration of multiple immune cells. Cassius et al. observed T cell dysregulation in patients with active diabetes (28). Zhang et al. showed that spinal cord tissues of NTDs patients had different genome-wide methylation patterns. Abnormal methylation pattern of TRIM4 in immune pathway may be involved in the pathogenesis of NTD (29). Additionally, B cells, macrophages, and natural killer cells have also been shown to significantly affect the progression of diabetes, and to lead indirectly to the occurrence of NTDs (30-32). The present study showed that the T cells, B cells, and natural killer cells associated with diabetes were also more highly expressed in the NTDs samples than the healthy samples. Thus, these results suggest that the deregulation of multiple immune-related genes in embryos may lead to alterations in immune cell infiltration, which may lead to the occurrence and development of NTDs. A limitation of this study is that no real-world experiments have been conducted to verify the differences in the expression of hub immune-related genes between healthy and NTDs embryos.
Conclusions
Immune-related genes may act as critical regulators of NTDs, but the specific mechanism by which this occurs remains unclear. Our findings may pave the way for future research in the immune-related diagnosis and treatment of NTDs.
Acknowledgments
Funding: This work was supported by Scientific Research and Technology Development Project of Baise City (No. 20213731) and Guangxi Zhuang Autonomous Region Health and Family Planning Commission project (No. 220180220).
Footnote
Reporting Checklist: The authors have completed the STREGA reporting checklist. Available at https://atm.amegroups.com/article/view/10.21037/atm-22-1273/rc
Conflicts of Interest: All authors have completed the ICMJE uniform disclosure form (available at https://atm.amegroups.com/article/view/10.21037/atm-22-1273/coif). The authors have no conflicts of interest to declare.
Ethical Statement: The authors are accountable for all aspects of the work in ensuring that questions related to the accuracy or integrity of any part of the work are appropriately investigated and resolved. The study was conducted in accordance with the Declaration of Helsinki (as revised in 2013).
Open Access Statement: This is an Open Access article distributed in accordance with the Creative Commons Attribution-NonCommercial-NoDerivs 4.0 International License (CC BY-NC-ND 4.0), which permits the non-commercial replication and distribution of the article with the strict proviso that no changes or edits are made and the original work is properly cited (including links to both the formal publication through the relevant DOI and the license). See: https://creativecommons.org/licenses/by-nc-nd/4.0/.
References
- Shamsuddin H, Raudenbush CL, Sciba BL, et al. Evaluation of Neural Tube Defects (NTDs) After Exposure to Raltegravir During Pregnancy. J Acquir Immune Defic Syndr 2019;81:247-50. [Crossref] [PubMed]
- Centers for Disease Control and Prevention, National Center on Birth Defects and Developmental Disabilities. [database on the Internet]. Available online: https://www.cdc.gov/ncbddd/birthdefectscount/index.html
- Joosten PH, van Zoelen EJ, Murre C. Pax1/E2a double-mutant mice develop non-lethal neural tube defects that resemble human malformations. Transgenic Res 2005;14:983-7. [Crossref] [PubMed]
- Copp AJ, Stanier P, Greene ND. Neural tube defects: recent advances, unsolved questions, and controversies. Lancet Neurol 2013;12:799-810. [Crossref] [PubMed]
- Copp AJ, Greene ND. Genetics and development of neural tube defects. J Pathol 2010;220:217-30. [Crossref] [PubMed]
- Finnell RH, Gould A, Spiegelstein O. Pathobiology and genetics of neural tube defects. Epilepsia 2003;44:14-23. [Crossref] [PubMed]
- Karzbrun E, Khankhel AH, Megale HC, et al. Human neural tube morphogenesis in vitro by geometric constraints. Nature 2021;599:268-72. [Crossref] [PubMed]
- Wolujewicz P, Steele JW, Kaltschmidt JA, et al. Unraveling the complex genetics of neural tube defects: From biological models to human genomics and back. Genesis 2021;59:e23459. [Crossref] [PubMed]
- Wallingford JB, Niswander LA, Shaw GM, et al. The continuing challenge of understanding, preventing, and treating neural tube defects. Science 2013;339:1222002. [Crossref] [PubMed]
- Arnal A, Waleckx E, Rico-Chávez O, et al. Estimating the current burden of Chagas disease in Mexico: A systematic review and meta-analysis of epidemiological surveys from 2006 to 2017. PLoS Negl Trop Dis 2019;13:e0006859. [Crossref] [PubMed]
- Denny KJ, Jeanes A, Fathe K, et al. Neural tube defects, folate, and immune modulation. Birth Defects Res A Clin Mol Teratol 2013;97:602-9. [Crossref] [PubMed]
- Tissir F, Wang CE, Goffinet AM. Expression of the chemokine receptor Cxcr4 mRNA during mouse brain development. Brain Res Dev Brain Res 2004;149:63-71. [Crossref] [PubMed]
- McLin VA, Hu CH, Shah R, et al. Expression of complement components coincides with early patterning and organogenesis in Xenopus laevis. Int J Dev Biol 2008;52:1123-33. [Crossref] [PubMed]
- Greene ND, Copp AJ. Neural tube defects. Annu Rev Neurosci 2014;37:221-42. [Crossref] [PubMed]
- Mousa A, Naqash A, Lim S. Macronutrient and Micronutrient Intake during Pregnancy: An Overview of Recent Evidence. Nutrients 2019;11:443. [Crossref] [PubMed]
- Blom HJ, Shaw GM, den Heijer M, et al. Neural tube defects and folate: case far from closed. Nat Rev Neurosci 2006;7:724-31. [Crossref] [PubMed]
- Adzick NS, Thom EA, Spong CY, et al. A randomized trial of prenatal versus postnatal repair of myelomeningocele. N Engl J Med 2011;364:993-1004. [Crossref] [PubMed]
- Mosley BS, Cleves MA, Siega-Riz AM, et al. Neural tube defects and maternal folate intake among pregnancies conceived after folic acid fortification in the United States. Am J Epidemiol 2009;169:9-17. [Crossref] [PubMed]
- Mallela M, Hrubec T. Reduction in valproic acid-induced neural tube defects by maternal immune stimulation: role of apoptosis. Birth Defects Res B Dev Reprod Toxicol 2012;95:296-303. [Crossref] [PubMed]
- Dong N, Gu H, Liu D, et al. Complement factors and alpha-fetoprotein as biomarkers for noninvasive prenatal diagnosis of neural tube defects. Ann N Y Acad Sci 2020;1478:75-91. [Crossref] [PubMed]
- Wani NA, Hamid A, Kaur J. Folate status in various pathophysiological conditions. IUBMB Life 2008;60:834-42. [Crossref] [PubMed]
- Zabihi S, Loeken MR. Understanding diabetic teratogenesis: where are we now and where are we going? Birth Defects Res A Clin Mol Teratol 2010;88:779-90. [Crossref] [PubMed]
- Jensen LE, Etheredge AJ, Brown KS, et al. Maternal genotype for the monocyte chemoattractant protein 1 A(-2518)G promoter polymorphism is associated with the risk of spina bifida in offspring. Am J Med Genet A 2006;140:1114-8. [Crossref] [PubMed]
- Yadav A, Saini V, Arora S. MCP-1: chemoattractant with a role beyond immunity: a review. Clin Chim Acta 2010;411:1570-9. [Crossref] [PubMed]
- Li M, Chen J, Li YS, et al. Folic acid reduces chemokine MCP-1 release and expression in rats with hyperhomocystinemia. Cardiovasc Pathol 2007;16:305-9. [Crossref] [PubMed]
- Brown KS, Huang Y, Lu ZY, et al. Mild folate deficiency induces a proatherosclerotic phenotype in endothelial cells. Atherosclerosis 2006;189:133-41. [Crossref] [PubMed]
- Lu ZY, Jensen LE, Huang Y, et al. The up-regulation of monocyte chemoattractant protein-1 (MCP-1) in Ea.hy 926 endothelial cells under long-term low folate stress is mediated by the p38 MAPK pathway. Atherosclerosis 2009;205:48-54. [Crossref] [PubMed]
- Cassius C, Branchtein M, Battistella M, et al. Persistent deficiency of mucosal-associated invariant T cells during dermatomyositis. Rheumatology (Oxford) 2020;59:2282-6. [Crossref] [PubMed]
- Zhang H, Guo Y, Gu H, et al. TRIM4 is associated with neural tube defects based on genome-wide DNA methylation analysis. Clin Epigenetics 2019;11:17. [Crossref] [PubMed]
- Gonzalez-Amaro R, Alcocer-Varela J, Alarcón-Segovia D. Natural killer cell activity in dermatomyositis-polymyositis. J Rheumatol 1987;14:307-10. [PubMed]
- Piper CJM, Wilkinson MGL, Deakin CT, et al. CD19+CD24hiCD38hi B Cells Are Expanded in Juvenile Dermatomyositis and Exhibit a Pro-Inflammatory Phenotype After Activation Through Toll-Like Receptor 7 and Interferon-α. Front Immunol 2018;9:1372. [Crossref] [PubMed]
- Jiang T, Huang Y, Liu H, et al. Reduced miR-146a Promotes REG3A Expression and Macrophage Migration in Polymyositis and Dermatomyositis. Front Immunol 2020;11:37. [Crossref] [PubMed]
(English Language Editor: L. Huleatt)