This article has an erratum available at: http://dx.doi.org/10.21037/atm-2022-38 the article has been update on 2023-02-08 at here.
Overexpression of miR-651-5p inhibits ultraviolet radiation-induced malignant biological behaviors of sebaceous gland carcinoma cells by targeting ZEB2
Introduction
Sebaceous gland carcinoma (SGC) is a type of metastatic and fatal cutaneous malignancy, and its overall incidence is increasing worldwide (1). Wide excision and selective use of radiotherapy are the main therapies for SGC, but SGC is often misdiagnosed, leading to increased morbidity and mortality. In addition, high invasiveness indicates the poor prognosis of SGC (2). The poor prognosis of SGC may improve with early identification and appropriate treatment. Thus, new insights into its pathogenesis may lead to the development of new effective treatment strategies, along with traditional therapies. As one study reported, exposure to ultraviolet (UV) radiation plays an important role in the etiology of SGC (3). Recently, another study indicated the predominance of a UV-damage signature based on whole-exome sequencing of SGC samples (4). However, the mechanism underlying the effect of UV on the development of SGC still needs further research.
As a mutagen and nonspecific injurious agent, UV (mainly UVA and UVB) radiation is both a tumor inducer and promoter, so it is considered a “complete carcinogen” (5). Many studies have shown that skin photoaging mediated by UV radiation is one of the inducing factors of skin tumors (6,7), especially skin neoplasms of the head and neck (8). It has been reported that UV radiation affects the proliferation, apoptosis (9), migration, and invasion of tumor cells, as well as epithelial-mesenchymal transition (EMT) (10). A previous study showed that UV radiation can induce the apoptosis of melanoma cells (11) and promote the migration and invasion of melanoma cells (12) as well as EMT (10,13), thus promoting the growth and metastasis of tumors.
Increasing evidence indicates that UV exposure regulates the expression of microRNAs (miRNAs). A recent study reported that UV exposure affects the developmental dynamics of miRNAs and phased small interfering RNA (phasiRNA)-producing loci (14). Moreover, abnormal expression of miRNAs is involved in various cancers, including SGC. Research has demonstrated that aberrant expression of miRNAs, such as miR-34a-5p, miR-16-5p, miR-150p, miR-205, and miR-199a, is a potential marker of SGC (15). Another report indicated that miR-939-5p, miR-130a-3p, miR-149-3p, miR-193a-3p, miR-195-5p, and miR-4671-3p are related to functional changes in SGC (16). One study suggested that the expression of miR-651-5p is obviously upregulated in breast cancer, but its specific mechanism has not been studied (17). Previous reports also showed that miR-651 is involved in the progression of non-small cell lung cancer (NSCLC) and nasopharyngeal carcinoma (18,19). Interestingly, miR-651-3p is involved in regulating the sensitivity of hepatocellular carcinoma and cervical cancer to cisplatin (20,21), suggesting that the miR-651-3p has multiple biological function. In addition, miR-651 was reported to be expressed at low levels in SGC, especially in nodular SGC (15). However, whether miR-651-5p expression affects the development and progression of UV-induced SGC remains unclear.
MiRNAs participate in the biological processes of cancer cells by mediating post-transcriptional gene silencing (22). Based on bioinformatics data (StarBase; https://starbase.sysu.edu.cn/agoClipRNA.php?source=mRNA), zinc finger E-box binding homeobox 2 (ZEB2) is one of the target genes of miR-651-5p. ZEB2, an important EMT regulator, has been reported to be highly expressed in SGC and is the best poor prognostic indicator for SGC (23). There is growing evidence that ZEB2 plays a pivotal role in EMT-induced tumorigenesis, differentiation, and malignancy, such as drug resistance, tumor stem cell-like characteristics, apoptosis, survival, cell cycle arrest, tumor recurrence, and metastasis (24). In addition, a previous report revealed that ZEB2 knockdown can inhibit the proliferation, migration, invasion, and EMT of glioma cells and induce glioma cell apoptosis (25). Whether miR-651-5p acts as a regulatory factor in SGC by targeting ZEB2 needs further research.
Targeted therapy is an important strategy for improving the clinical treatment of tumors, especially late-stage solid tumors, and improving the quality of life of patients. In recent years, there has been great interest in the development of intelligent drug delivery systems (drug-loaded particles) using passive targeting, active targeting, and/or stimulation response strategies (26). Therefore, research on the molecular mechanisms of tumorigenesis and development is helpful for exploring potential cellular or molecular targets for tumor-targeted therapy (27,28). In this study, the effect of UV exposure on the expression of miR-651-5p in SGC was studied, and the relationship between miR-651-5p and ZEB2 was verified. In addition, the apoptosis, invasion, migration, and EMT of SGC cells after UV treatment were measured. Furthermore, the mechanism by which miR-651-5p affects UV-induced SGC was studied to provide new insights into the pathogenesis of SGC and to develop a new effective therapeutic target. We present the following article in accordance with the ARRIVE reporting checklist (available at https://atm.amegroups.com/article/view/10.21037/atm-21-3897/rc).
Methods
Tissue samples and isolation of primary SGC cells
SGC tumor tissues were obtained by surgical excision from five patients with eyelid SGC. Control samples were obtained from five adjacent normal epidermis tissues resected approximately 5 cm from the main tumor (29). None of the patients received radiotherapy or chemotherapy before surgery. The expression of miRNAs in these samples was measured by real-time quantitative polymerase chain reaction (RT-qPCR). SGC tissues were used to isolate primary SGC cells. The study was conducted in accordance with the Declaration of Helsinki (as revised in 2013). This study was approved by the Medical Ethics Committee of Kunming University of Science and Technology (No. KMUST-MEC-040), and written informed consent was obtained from each participant before sample collection.
Primary SGC cells were isolated from SGC tissues as described in a previous report (30). Fresh SGC tissues were placed in a culture dish containing phosphate-buffered saline (PBS; #10010023; Gibco; Thermo Fisher Scientific, Inc., USA). Blood clots, fat, necrotic tissue, and connective tissue were removed with ophthalmic scissors, and the cells were washed twice with PBS. The SGC tissues were placed into a new culture dish, and a small amount of Dulbecco’s modified Eagle medium (DMEM; #11965092; Gibco; Thermo Fisher Scientific, Inc., USA) was added. The tissues were cut into pieces with ophthalmic scissors. Then, the cells were transferred to centrifuge tubes and washed with PBS several times, and the PBS was discarded after the tissue block sank. The tissue pieces were transferred into culture flasks, and collagenase (#10269638001; Merck; Sigma-Aldrich, China) was added. The tissues were digested on a constant temperature shaking table at 37 ℃ and observed under a microscope every 30 min. When the tissue pieces had good light transmittance and aggregated under the microscope, they were transferred into centrifuge tubes and centrifuged at 1,300 r/min for 5 min, and the supernatant was discarded. PBS was added to wash the cells several times, then they were centrifuged at 1,300 r/min for 5 min and the supernatant was discarded. Complete DMEM was used to resuspend the cells, and the suspension was transferred to a culture dish. Primary SGC cells were obtained when single cells or cell clumps were observed under the microscope.
Cell culture and transfection
The immortalized human sebaceous gland cell line SZ95 (31) was purchased from BeNa Culture Collection (BNCC338262, BNCC, China) and cultured in Sebomed® Basal Medium (#F8025; Biochrom, Berlin, Germany) containing 10% fetal bovine serum (FBS, #16140071; Gibco; Thermo Fisher Scientific, Inc., USA) and 5 ng/mL recombinant human epidermal growth factor (#PHG0313; Gibco; Thermo Fisher Scientific, Inc., USA) at 37 ℃ and 5% CO2 according to a previous report (32).
MiR-651-5p mimics and pcDNA-ZEB2 were constructed by Guangzhou RiboBio Biotechnology Co., Ltd. (Guangzhou, China) and were transfected with Lipofectamine® 2000 transfection reagent (#11668027; Invitrogen; Thermo Fisher Scientific, Inc., USA) following the instructions.
Application of UV treatment to SGC cells
UV treatment was applied to SGC cells with lamps (UVP) emitting a spectral output in the 290–400 nm range (60% UVB, 40% UVA, <1% UVC) (Upland, CA, USA). To assess the influence of UV radiation on SGC cells, primary SGC cells were cultured in 24-well plates and received UV radiation at a single dose of 0, 10, 30, or 50 mJ/cm2 according to a previous study (33). Then, the apoptosis, migration, invasion, and EMT of SGC cells were measured 48 h after UV treatment. A UV dose of 50 mJ/cm2 was used for further in vitro studies.
RT-qPCR
RT-qPCR was used to measure expression levels in this study. Total RNA was extracted from human SGC tissues and SGC cells from each group with TRIzol reagent (#15596018; Invitrogen; Thermo Fisher Scientific, Inc., USA). Then, RT-qPCR was performed in triplicate with SYBR Green Real-Time PCR Master Mix (#4309155; Applied Biosystems; Thermo Fisher Scientific, Inc., USA) on an ABI 7500 Real-Time PCR System (Applied Biosystems; Thermo Fisher Scientific, Inc., USA). The thermocycling conditions were as follows: initial denaturation at 95 ℃ for 10 sec followed by 40 cycles of 95 ℃ for 10 sec and 60 ℃ for 30 sec. The relative expression levels of mRNAs and miRNAs were presented as fold changes, which were calculated by the 2−ΔΔCT method. GAPDH and U6 were used for normalization of mRNA levels and miRNA levels, respectively. The primer sequences used were as follows: human GAPDH, forward: 5'-TTCCGTGTTCCTACCC-3', and reverse: 5'-GTCGCAGGAGACAACC-3'; human U6, forward: 5'-CTCGCTTCGGCAGCACATATACT-3', and reverse: 5'-ACGCTTCACGAATTTGCGTGTC-3'; human ZEB2, forward: 5'-ACCAGCGGAAACAAGGAT-3', and reverse: 3'-ACCAGCGGAAACAAGGAT-5'; human miR-651-5p, forward: 5'-CGCAGTTTAGGATAAGCTTG-3', and reverse: 3'-TCCAGTTTTTTTTTTTTTTTCAAAAGTC-5'. In addition, RT-qPCR for miRNAs was carried out using a TaqManTM MicroRNA Reverse Transcription Kit (#4366597; Applied Biosystems; Thermo Fisher Scientific, Inc., USA) and TaqMan Universal PCR Master Mix (#4304437; Applied Biosystems; Thermo Fisher Scientific, Inc., USA).
Western blotting
Western blotting was used to measure the expression levels of proteins in this study. SGC cells from each group were lysed with radioimmunoprecipitation assay (RIPA) protein lysis buffer (#89900; Invitrogen; Thermo Fisher Scientific, Inc., USA), and the protein content was determined with a BCA kit (#23252; Pierce; Thermo Fisher Scientific, Inc., USA). Equivalent amounts of protein (40 µg) were separated by SDS-PAGE then transferred to polyvinylidene difluoride (PVDF) membranes and blocked with skimmed milk at room temperature for 1 h. After incubation with primary and secondary antibodies, the protein bands were visualized by enhanced chemiluminescence (#32106; Pierce; Thermo Fisher Scientific, Inc., USA). The following primary antibodies were used at a dilution of 1:1,000: anti-p63 (#39692; 1:1,000; CST, USA), anti-EMA (#14161; 1:1,000; CST, USA), anti-CKpan (#ab7753; 1:500; Abcam, USA), anti-ZEB2 (#97885, 1:1,000; CST, USA), anti-E-cadherin (#3195; 1:1,000; CST, USA), anti-N-cadherin (#13116; 1:1,000; CST, USA), anti-vimentin (#5741; 1:1,000; CST, USA), and anti-GAPDH (#5174; 1:1,000; CST, USA). Grayscale value analysis was performed using ImageJ software (version 1.52a; National Institutes of Health), and GAPDH was used as the loading control.
Flow cytometry
Flow cytometry was used to measure the cell apoptosis rate. SGC cells in each group were harvested and resuspended. Apoptotic cells were double-labeled with annexin V-FITC and propidium iodide (PI) using an annexin V-FITC/PI apoptosis detection kit (#40302ES20; Yeasen; Shanghai, China) for 30min in the dark. Then, the fluorescence intensity of the cells was quantified by flow cytometry.
Transwell assay
Transwell chambers coated with or without Matrigel (#356234; Corning, Wuhan, China) were used to assess cell invasion and migration ability. SGC cells from each group were plated in the upper chamber (5×104 cells per chamber, 150 µL per chamber) without FBS, and medium with 10% FBS was added to the lower chamber. After incubation at 37 ℃ for 24 h, the cells in the upper chamber were removed, and the invaded cells on the lower surface were stained with crystal violet (#E607309; Sandon Biotech; Shanghai, China) and photographed under a microscope. The cell number was determined by Image-Pro Plus IPWIN32.
Dual-luciferase reporter assay
The binding sites between miR-651-5p and ZEB2 were predicted with StarBase (https://starbase.sysu.edu.cn/agoClipRNA.php?source=mRNA). Luciferase vectors containing the 3'UTR of human ZEB2 with miR-651-5p binding sites and mutant miR-651-5p binding sites were purchased from Shanghai GenePharma Co., Ltd. Then, the vectors were cotransfected with miR-651-5p mimics into 293T cells by Lipofectamine® 2000 transfection reagent (#11668027; Invitrogen; Thermo Fisher Scientific, Inc., USA). After 48 h, luciferase reporter activity was determined using a Dual-Luciferase® Reporter Assay System (Promega Corporation).
In vivo tumor growth assay
An in vivo tumor growth assay was used to measure tumor growth according to previously described methods (34,35). A protocol was prepared before the study without registration. Animal experiments were approved by the Institutional Animal Care and Use Committee of Kunming University of Science and Technology, in compliance with the Kunming University of Science and Technology guidelines for the care and use of animals. A total of 107 cultured human SGC cells [normal control (NC)-, miR-651-5p mimics-, and miR-651-5p mimics + pcDNA-ZEB2-transfected cells] suspended in 0.2 mL of PBS were subcutaneously injected into the right armpits of 8-week-old female BALB/c nude mice (n=6 per group, day 0) to establish a nude mouse xenograft tumor model. Subsequently, the mice were placed in the supine position, and only the right axillary skin was exposed to UV radiation (1,000 J/m2/d) daily according to previously described methods with minor modification (36). The length (L) and width (W) of the tumor were assessed 4, 8, 12, 16, 20, 24, and 28 days after injection. The tumor volumes were calculated as V = L × W2 × 0.5. The mice were sacrificed on day 28, and the tumors were weighed.
Terminal deoxynucleotidyl transferase dUTP nick-end labeling (TUNEL) assay
SGC cells were treated or transfected and then cultured for 48 hours with a 2 PBS wash. After this, SGC cells underwent 4% PFA fixation for 15 minutes at 37 ℃. 2×104 cells were prepared in 96-well plates and then subjected to Click-iTTM Plus TUNEL Assay Kit (ThermoFisher Scientific, Waltham, MA, USA) following the manufacturer’s instructions. Following DAPI staining, the apoptotic cells were measured by using a Nikon Eclipse 80i microscope (Nikon Corporation, Japan).
Statistical analyses
All data are presented as the mean ± standard deviation (SD), and all experiments were repeated independently at least 3 times. Two-tailed Student’s t-tests were carried out for two-group comparisons. One-way analysis of variance (ANOVA) or two-way ANOVA followed by the Bonferroni post hoc test was used to assess differences between more than two groups. Statistical analyses were performed with GraphPad Prism 5.0 software (GraphPad Software, Inc., USA). P<0.05 indicated a statistically significant difference.
Results
Expression of miR-651-5p and ZEB2 in SGC tissues and cells
Aberrantly expressed miRNAs are related to various cancers (37), and research has also indicated that UV regulates the expression of miRNAs (38). In this study, we examined the expression of miR-651-5p and ZEB2 mRNA in SGC tissues and adjacent tissues by RT-qPCR. The results showed that miR-651-5p was expressed at low levels in tumor tissues, while ZEB2 mRNA was highly expressed in tumor tissues (Figure 1A,1B). Subsequently, primary SGC cells were isolated from SGC tissues, and the expression of SGC markers (p63, EMA, and CKpan) was verified by western blotting (39,40). The results showed that these markers were strongly expressed in isolated SGC cells (P<0.05 compared with human normal sebaceous gland cell line SZ95 cells, Figure 1C,1D). The results also showed that the expression of miR-651-5p was lower in SGC cells than in the SZ95 (Figure 1E). Moreover, as shown in Figure 1F, the expression of ZEB2 in SGC cells was higher than that in SZ95 cells. Hence, the abnormal expression of miR-651-5p and ZEB2 in SGC tissues and SGC cells might be related to the development and progression of SGC.
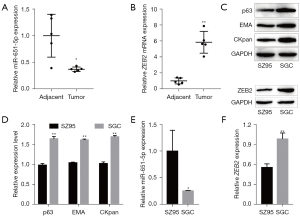
The effect of UV exposure on SGC cells
A recent study found that UV radiation regulated the expression of miRNAs, which affected the apoptosis of immortalized human epidermal cells (41). The effect of UV exposure on the apoptosis, invasion, migration, and EMT of SGC cells was assessed. The expression of miR-651-5p in UV-induced SGC cells was measured by RT-qPCR, and the miR-651-5p expression level was found to decrease with increasing doses of UV radiation (Figure 2A). Flow cytometry and TUNEL staining results shown that UV radiation promoted SGC cell apoptosis in a dose-dependent manner, thus inhibiting the growth of the tumor (Figure 2B,2C). In contrast, UV exposure induced SGC cell invasion, migration, and EMT in a dose-dependent manner (Figure 2D-2F), which was conducive to tumor metastasis. These results suggested that although UV radiation could promote the apoptosis of SGC cells, it could also enhance the malignant biological behaviors of SGC cells.
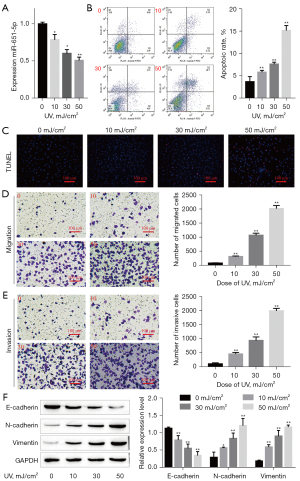
miR-651-5p affected UV-induced SGC cells
Based on the above results, we were further study the effect of miR-651-5p on the biological behaviors of SGC cells after UV treatment. First, the expression level of miR-651-5p in miR-651-5p mimic-, inhibitor-, inhibitor-NC-, and mimic-NC-transfected SGC cells treated with or without UV was verified by RT-qPCR. The results showed that UV radiation suppressed miR-651-5p expression, and the expression of miR-651-5p was upregulated and downregulated by miR-651-5p mimics and miR-651-5p inhibitors, respectively (Figure 3A,3B). The flow cytometry and TUNEL staining results showed that SGC cell apoptosis induced by UV exposure, and was enhanced by overexpression of miR-651-5p after UV radiation, but was reduced by downexpression of miR-651-5p (Figure 3C,3D). Transwell results showed that overexpression of miR-651-5p inhibited UV-induced SGC cell invasion and migration and downexpression of miR-651-5p promoted UV-induced SGC cell invasion and migration (Figure 4A,4B). Moreover, the western blotting results showed that overexpression of miR-651-5p inhibited EMT in UV-treated SGC cells and downexpression of miR-651-5p promoted EMT in UV-treated SGC cells (Figure 4C). Therefore, overexpression of miR-651-5p markedly inhibited the malignant biological behaviors of UV-induced SGC cells and enhanced cell apoptosis induced by UV radiation.
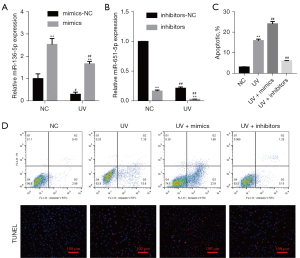
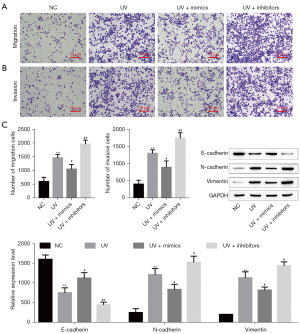
Knockdown of ZEB2 affected UV-induced SGC cells
Based on the above results, ZEB2 was downexpression to further study the effect of ZEB2 on the biological behaviors of SGC cells after UV treatment. The SGC cells were transfected with siRNAs to downregulate ZEB2 expression. The protein levels of ZEB2 were significantly reduced (Figure 5A). si-ZEB2#1 achieved more effective knockdown efficiency. The flow cytometry and TUNEL staining results showed that ZEB2 depletion significantly increased UV-induced SGC cell apoptosis (Figure 5B,5C). Transwell results showed that knockdown of ZEB2 inhibited UV-induced SGC cell invasion and migration (Figure 5D,5E). Moreover, the western blotting results showed that knockdown of ZEB2 inhibited EMT in UV-treated SGC cells (Figure 5F). This finding indicated that UV radiation regulates SGC cell apoptosis, migration, and invasion by downregulating ZEB2.
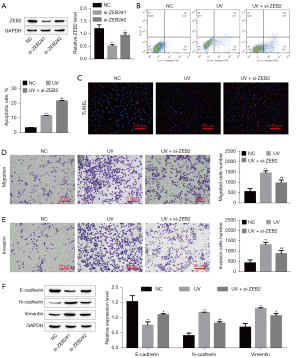
Interaction between miR-651-5p and ZEB2
According to the bioinformatics data, the binding sites between miR-651-5p and ZEB2 were predicted by the StarBase database (Figure 6A). Moreover, the dual-luciferase reporter assay results showed that overexpression of miR-651-5p dramatically decreased the luciferase activity of the wt-ZEB2 3'UTR reporter, but had no effect on the activity of the mut-ZEB2 reporter (Figure 6B). In addition, the results showed that compared with mimic-NC-transfected SGC cells, the expression of ZEB2 in the miR-651-5p mimic-transfected SGC cells was significantly decreased (Figure 6C,6D). Thus, the expression of ZEB2 was inhibited by miR-651-5p in SGC cells.
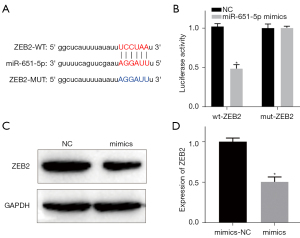
MiR-651-5p regulated the malignant biological behavior of UV-induced SGC by targeting ZEB2 in vivo and in vitro
MiRNAs are involved in the progression of cancer, as they regulate target genes (42). The above results showed that miR-651-5p targets ZEB2 in SGC cells (Figure 6). Because miR-651-5p overexpression and ZEB2 downexpression enhanced cell apoptosis and suppressed the malignant biological behaviors of UV-induced SGC cells (Figures 3-5), ZEB2 was overexpressed concurrently to verify whether miR-651-5p regulated the malignant biological behaviors of UV-exposed SGC cells. The flow cytometry and TUNEL staining results showed that cell apoptosis of UV-induced SGC cells was inhibited in the miR-651-5p mimic- and pcDNA-ZEB2-cotransfection group compared with the miR-651-5p mimic-group (Figure 7A,7B). In contrast, the inhibition of invasion, migration, and EMT by miR-651-5p overexpression in UV-induced SGC cells was reversed by ZEB2 overexpression (Figure 7C-7E). Moreover, the in vivo tumor growth assay results showed that overexpression of miR-651-5p inhibited tumor growth, while the volume and weight of tumors in the miR-651-5p mimic- and pcDNA-ZEB2-cotransfected group were not significantly different from those in the UV radiation-only groups (Figure 7F-7H). Consequently, the above results confirmed that miR-651-5p overexpression suppressed the malignant biological behaviors of UV-treated SGC cells by targeted downregulation of ZEB2 expression in vivo and in vitro.
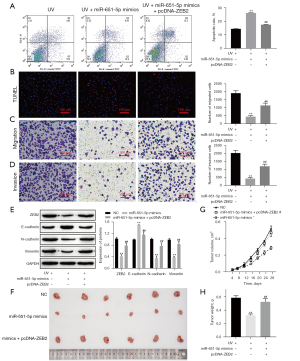
Discussion
Tumor microenvironment (TME) refers to the cellular environment in which tumors or cancer stem cells exist. The TME is a complex ecology consisting of cells that evolve with cancer cells and provide support during malignant transformation, which contains cells and molecules that can increase the stemness of tumor cells, promote angiogenesis, mediate migration, induce drug resistance, and suppress the immune system (43). It has been reported that UV radiation affects the proliferation, apoptosis (9), migration, and invasion of tumor cells, as well as EMT (10). UV radiation damages DNA to cells triggers mutations that lead the cells to multiply rapidly and form malignant tumors (44). SGC is a rare invasive tumor with a high local recurrence rate and poor prognosis after metastasis. Previous studies reported that UV exposure is showed poorly differentiated infiltrating of SGC, which is related to the UV-induced DNA damage, gene mutation, infiltrating neutrophils, proteolytic enzymes induced and keratinocyte activation (3,4,45,46). UV-induced SGC show more aggressive and sebocytic differentiation histopathologic features (4). It was demonstrated that skin irradiation by UV downregulates the expression of cysteine-rich protein 1, which leads to the occurrence of SGC (47). In addition, UV light signature mutations in the telomerase reverse transcriptase gene promoter are very common in SGC, indicating the important roles of UV light in the pathogenesis of SGC (48). However, even though various factors caused by UV exposure lead to the occurrence and development of SGC, a study reported the existence of UV-independent p53 mutations in SGC (49). Moreover, a recent study found that UV radiation upregulates the expression of miR-27a, which affects the apoptosis of immortalized human epidermal cells by removing cyclobutene pyrimidine dimers and by downregulating its target genes, namely transactive response DNA-binding protein and apoptotic protease activating factor-1 (50). In addition, miR-26a expression is upregulated upon UV irradiation and promotes UV-induced apoptosis in immortalized human epidermal cells by targeting the histone methyltransferase EZH2 (51). In this study, miR-651-5p expression was confirmed to be abnormally low in SGC tissues and SGC cells, and its expression had a negative correlation with the intensity of UV exposure. These results suggested that UV might affect the development of SGC by regulating the expression of miR-651-5p.
UV radiation can regulate the expression of miRNAs, and it has been reported that abnormal expression of miRNAs is closely related to SGC. For example, low levels of miRNA-200c and miRNA-141 in patients with SGC facilitate tumor progression (29). In addition, overexpression of miR-486-5p and miR-184 has been reported to be oncogenic in SGC, and downregulation of miR-211 and miR-518d has been reported to have tumor-suppressive effects in SGC (52). Focusing on miR-651-5p, a study reported that miR-651 is associated with the clinical outcomes of NSCLC patients (19), and circRNA_0066755 acts as an oncogene by sponging miR-651 in nasopharyngeal carcinoma (18), which indicates that miR-651 might act as a tumor suppressor gene. The results of the present study suggested that overexpression of miR-651-5p promoted cell apoptosis and inhibited the invasion, migration, and EMT of UV-treated SGC cells, thereby inhibiting the malignant biological behaviors of UV-treated SGC cells. Mechanistically, miRNAs affect the malignant biological behaviors of cancer cells by regulating target genes. Based on the bioinformatics data and the results of the dual-luciferase reporter assay, miR-651-5p targeted ZEB2 and downregulated its expression in SGC cells.
As a DNA-binding transcription factor, ZEB2 is closely related to the progression of cancer. One study reported that overexpression of ZEB2 promotes the expression of EMT-associated genes and proteins, and alters cell morphology and invasive capability, and the results clearly identified ZEB2-mediated transcription as a key mechanism that transforms epithelial-like trophoblasts into cells with a mesenchymal, invasive phenotype (53). Because ZEB2 is an important regulator of EMT, the miRNA-ZEB2 axis has been reported to play a crucial role in the tumor progression of prostate carcinoma (54), oral squamous cell carcinoma (55), and esophageal cancer (56), among others. Moreover, a study found that the overexpression of ZEB2 in SGC is associated with systemic metastasis in 8–14% of patients and death in 10–30% of patients (57). In addition, the expression of ZEB2 is directly associated with vimentin, which is overexpressed in SGC and leads to poor clinical outcomes (58). In this study, the overexpression of ZEB2 was proven to enhance the invasion, migration, and EMT of UV-induced SGC cells, but inhibited the apoptosis of miR-651-5p-overexpressing SGC cells after UV exposure. These results indicated that miR-651-5p regulated the malignant biological behaviors of UV-induced SGC cells by targeting ZEB2.
The present study only selected ZEB2 as the targets of miR-651-5p, miR-651-5p may have the possibilities to targeting other genes and future studies may investigate into more targets of miR-651-5p. In addition, we find the miR-651-5p lowly expressed in the SGC, whether the reasons of miR-651-5p downexpression. As such, future studies may investigate into the upstream mechanism of regulating miR-651-5p expression, such as long non-coding RNA, circular RNA, m6A modification regulates miRNA mature processing, which will further reveal the underlying anti-tumor mechanisms in SGC. Interestingly, heavily UV-damaged samples SGC shared transcriptomic patterns resembling those found in squamous cell carcinomas and basal cell carcinomas, implicating poorly differentiated UV-damage SGC, arise from a subpopulation of more superficial keratinocytes vulnerable to UV mutagenesis, either from the epidermis or superficial portion of the folliculosebaceous unit (4).
In conclusion, this study revealed that the expression of miR-651-5p was low in SGC tissues, and its expression decreased as the intensity of UV exposure increased. Moreover, overexpression of miR-651-5p inhibited the malignant biological behaviors of UV-induced SGC cells via targeted downregulation of the expression of ZEB2. Our study has elucidated one of the mechanisms of UV-induced SGC growth and metastasis and provides a new target for gene therapy for SGC.
Acknowledgments
Funding: This study was supported by grants from (I) Science and Technology Talents and Platform Plan of Yunnan Province (grant No. 2019HB050); (II) Yunnan Science and Technology Commission from Yunnan provincial Science and Technology Department and Kunming Medical University (grant No. 2018FE001-180); (III) Youth Top-Notch Talent Project of Ten Thousand People Plan of Yunnan Province (grant No. YNWR-QNBJ-2018-315); (IV) Yunnan Jing Shangzhen Expert Workstation (grant No. 202005AF150030).
Footnote
Reporting Checklist: The authors have completed the ARRIVE reporting checklist. Available at https://atm.amegroups.com/article/view/10.21037/atm-21-3897/rc
Data Sharing Statement: Available at https://atm.amegroups.com/article/view/10.21037/atm-21-3897/dss
Conflicts of Interest: All authors have completed the ICMJE uniform disclosure form (available at https://atm.amegroups.com/article/view/10.21037/atm-21-3897/coif). The authors have no conflicts of interest to declare.
Ethical Statement: The authors are accountable for all aspects of the work in ensuring that questions related to the accuracy or integrity of any part of the work are appropriately investigated and resolved. The study was conducted in accordance with the Declaration of Helsinki (as revised in 2013). This study was approved by the Medical Ethics Committee of Kunming University of Science and Technology (No. KMUST-MEC-040), and written informed consent was obtained from the patients. Animal experiments were approved by the Institutional Animal Care and Use Committee of Kunming University of Science and Technology, in compliance with the Kunming University of Science and Technology guidelines for the care and use of animals.
Open Access Statement: This is an Open Access article distributed in accordance with the Creative Commons Attribution-NonCommercial-NoDerivs 4.0 International License (CC BY-NC-ND 4.0), which permits the non-commercial replication and distribution of the article with the strict proviso that no changes or edits are made and the original work is properly cited (including links to both the formal publication through the relevant DOI and the license). See: https://creativecommons.org/licenses/by-nc-nd/4.0/.
References
- Orr CK, Yazdanie F, Shinder R. Current review of sebaceous cell carcinoma. Curr Opin Ophthalmol 2018;29:445-50. [Crossref] [PubMed]
- Sekgololo JM, Chauke RF, Tshazi N. A rare giant extraocular, anterior chest wall sebaceous carcinoma. Int J Surg Case Rep 2020;66:110-4. [Crossref] [PubMed]
- Zouboulis CC, Boschnakow A. Chronological ageing and photoageing of the human sebaceous gland. Clin Exp Dermatol 2001;26:600-7. [Crossref] [PubMed]
- North JP, Golovato J, Vaske CJ, et al. Cell of origin and mutation pattern define three clinically distinct classes of sebaceous carcinoma. Nat Commun 2018;9:1894. [Crossref] [PubMed]
- D'Orazio J, Jarrett S, Amaro-Ortiz A, et al. UV radiation and the skin. Int J Mol Sci 2013;14:12222-48. [Crossref] [PubMed]
- Liu-Smith F, Jia J, Zheng Y. UV-Induced Molecular Signaling Differences in Melanoma and Non-melanoma Skin Cancer. Adv Exp Med Biol 2017;996:27-40. [Crossref] [PubMed]
- Cadet J, Douki T. Formation of UV-induced DNA damage contributing to skin cancer development. Photochem Photobiol Sci 2018;17:1816-41. [Crossref] [PubMed]
- Kraft S, Granter SR. Molecular pathology of skin neoplasms of the head and neck. Arch Pathol Lab Med 2014;138:759-87. [Crossref] [PubMed]
- Lin JR, Qin HH, Wu WY, et al. Vitamin C protects against UV irradiation-induced apoptosis through reactivating silenced tumor suppressor genes p21 and p16 in a Tet-dependent DNA demethylation manner in human skin cancer cells. Cancer Biother Radiopharm 2014;29:257-64. [Crossref] [PubMed]
- Noguchi K, Dincman TA, Dalton AC, et al. Interleukin-like EMT inducer (ILEI) promotes melanoma invasiveness and is transcriptionally up-regulated by upstream stimulatory factor-1 (USF-1). J Biol Chem 2018;293:11401-14. [Crossref] [PubMed]
- Saladi SV, Wong PG, Trivedi AR, et al. BRG1 promotes survival of UV-irradiated melanoma cells by cooperating with MITF to activate the melanoma inhibitor of apoptosis gene. Pigment Cell Melanoma Res 2013;26:377-91. [Crossref] [PubMed]
- Wäster P, Orfanidis K, Eriksson I, et al. UV radiation promotes melanoma dissemination mediated by the sequential reaction axis of cathepsins-TGF-β1-FAP-α. Br J Cancer 2017;117:535-44. [Crossref] [PubMed]
- Jiang Y, Ge XY, Liu SM, et al. Nimotuzumab suppresses epithelial-mesenchymal transition and enhances apoptosis in low-dose UV-C treated salivary adenoid cystic carcinoma cell lines in vitro. Anticancer Drugs 2014;25:1052-60. [Crossref] [PubMed]
- Sunitha S, Loyola R, Alcalde JA, et al. The Role of UV-B light on Small RNA Activity During Grapevine Berry Development. G3 (Bethesda) 2019;9:769-87. [Crossref] [PubMed]
- Bladen JC, Wang J, Sangaralingam A, et al. MicroRNA and transcriptome analysis in periocular Sebaceous Gland Carcinoma. Sci Rep 2018;8:7531. [Crossref] [PubMed]
- Hirano T, Yunoki T, Furusawa Y, et al. Bioinformatics analysis of the microRNA-mRNA network in sebaceous gland carcinoma of the eyelid. Mol Med Rep 2021;23:44. [PubMed]
- Chang JT, Wang F, Chapin W, et al. Identification of MicroRNAs as Breast Cancer Prognosis Markers through the Cancer Genome Atlas. PLoS One 2016;11:e0168284. [Crossref] [PubMed]
- Wang J, Kong J, Nie Z, et al. Circular RNA Hsa_circ_0066755 as an Oncogene via sponging miR-651 and as a Promising Diagnostic Biomarker for Nasopharyngeal Carcinoma. Int J Med Sci 2020;17:1499-507. [Crossref] [PubMed]
- Pu X, Roth JA, Hildebrandt MA, et al. MicroRNA-related genetic variants associated with clinical outcomes in early-stage non-small cell lung cancer patients. Cancer Res 2013;73:1867-75. [Crossref] [PubMed]
- Zou L, Sun P, Zhang L. miR-651-3p Enhances the Sensitivity of Hepatocellular Carcinoma to Cisplatin via Targeting ATG3-Mediated Cell Autophagy. J Oncol 2021;2021:5391977. [Crossref] [PubMed]
- Zhu X, Long L, Xiao H, et al. Cancer-Derived Exosomal miR-651 as a Diagnostic Marker Restrains Cisplatin Resistance and Directly Targets ATG3 for Cervical Cancer. Dis Markers 2021;2021:1544784. [Crossref] [PubMed]
- Wang Y, Zhao H, Zhi W. SEMA4D under the posttranscriptional regulation of HuR and miR-4319 boosts cancer progression in esophageal squamous cell carcinoma. Cancer Biol Ther 2020;21:122-9. [Crossref] [PubMed]
- Bhardwaj M, Sen S, Sharma A, et al. ZEB2/SIP1 as novel prognostic indicator in eyelid sebaceous gland carcinoma. Hum Pathol 2015;46:1437-42. [Crossref] [PubMed]
- Fardi M, Alivand M, Baradaran B, et al. The crucial role of ZEB2: From development to epithelial-to-mesenchymal transition and cancer complexity. J Cell Physiol 2019; Epub ahead of print. [Crossref] [PubMed]
- Qi S, Song Y, Peng Y, et al. ZEB2 mediates multiple pathways regulating cell proliferation, migration, invasion, and apoptosis in glioma. PLoS One 2012;7:e38842. [Crossref] [PubMed]
- Morales-Cruz M, Delgado Y, Castillo B, et al. Smart Targeting To Improve Cancer Therapeutics. Drug Des Devel Ther 2019;13:3753-72. [Crossref] [PubMed]
- Kong MY, Li LY, Lou YM, et al. Chinese herbal medicines for prevention and treatment of colorectal cancer: From molecular mechanisms to potential clinical applications. J Integr Med 2020;18:369-84. [Crossref] [PubMed]
- Tang KY, Du SL, Wang QL, et al. Traditional Chinese medicine targeting cancer stem cells as an alternative treatment for hepatocellular carcinoma. J Integr Med 2020;18:196-202. [Crossref] [PubMed]
- Bhardwaj M, Sen S, Chosdol K, et al. miRNA-200c and miRNA-141 as potential prognostic biomarkers and regulators of epithelial-mesenchymal transition in eyelid sebaceous gland carcinoma. Br J Ophthalmol 2017;101:536-42. [Crossref] [PubMed]
- Saluja TS, Kumar V, Agrawal M, et al. Mitochondrial Stress-Mediated Targeting of Quiescent Cancer Stem Cells in Oral Squamous Cell Carcinoma. Cancer Manag Res 2020;12:4519-30. [Crossref] [PubMed]
- Zouboulis CC, Seltmann H, Neitzel H, et al. Establishment and characterization of an immortalized human sebaceous gland cell line (SZ95). J Invest Dermatol 1999;113:1011-20. [Crossref] [PubMed]
- Chen KC, Yang CH, Li TT, et al. Suppression of Propionibacterium acnes-stimulated proinflammatory cytokines by Chinese bayberry extracts and its active constituent myricetin in human sebocytes in vitro. Phytother Res 2019;33:1104-13. [Crossref] [PubMed]
- Jobe NP, Živicová V, Mifková A, et al. Fibroblasts potentiate melanoma cells in vitro invasiveness induced by UV-irradiated keratinocytes. Histochem Cell Biol 2018;149:503-16. [Crossref] [PubMed]
- Shi B, Li Y, Wang X, et al. Silencing of hypoxia inducible factor-1α by RNA interference inhibits growth of SK-NEP-1 Wilms tumour cells in vitro, and suppresses tumourigenesis and angiogenesis in vivo. Clin Exp Pharmacol Physiol 2016;43:626-33. [Crossref] [PubMed]
- Mitra AK, Davis DA, Tomar S, et al. In vivo tumor growth of high-grade serous ovarian cancer cell lines. Gynecol Oncol 2015;138:372-7. [Crossref] [PubMed]
- Jarrett SG, Novak M, Harris N, et al. NM23 deficiency promotes metastasis in a UV radiation-induced mouse model of human melanoma. Clin Exp Metastasis 2013;30:25-36. [Crossref] [PubMed]
- Gholami M, Larijani B, Zahedi Z, et al. Inflammation related miRNAs as an important player between obesity and cancers. J Diabetes Metab Disord 2019;18:675-92. [Crossref] [PubMed]
- Syed DN, Khan MI, Shabbir M, et al. MicroRNAs in skin response to UV radiation. Curr Drug Targets 2013;14:1128-34. [Crossref] [PubMed]
- Boecker W, Reusch M, Mielke V, et al. Spatial analysis of p63, K5 and K7 defines two groups of progenitor cells that differentially contribute to the maintenance of normal sebaceous glands, extraocular sebaceous carcinoma and benign sebaceous tumors. J Dermatol 2019;46:249-58. [Crossref] [PubMed]
- Schmitz EJ, Herwig-Carl MC, Holz FG, et al. Sebaceous gland carcinoma of the ocular adnexa - variability in clinical and histological appearance with analysis of immunohistochemical staining patterns. Graefes Arch Clin Exp Ophthalmol 2017;255:2277-85. [Crossref] [PubMed]
- Zhang Y, Yang C, Yang S, et al. MiRNA-27a decreases ultraviolet B irradiation-induced cell damage. J Cell Biochem 2020;121:1032-8. [Crossref] [PubMed]
- Wang N, Feng T, Liu X, et al. Curcumin inhibits migration and invasion of non-small cell lung cancer cells through up-regulation of miR-206 and suppression of PI3K/AKT/mTOR signaling pathway. Acta Pharm 2020;70:399-409. [Crossref] [PubMed]
- Arneth B. Tumor Microenvironment. Medicina (Kaunas) 2019;56:15. [Crossref] [PubMed]
- Kozovska Z, Gabrisova V, Kucerova L. Malignant melanoma: diagnosis, treatment and cancer stem cells. Neoplasma 2016;63:510-7. [Crossref] [PubMed]
- Sato T, Akimoto N, Takahashi A, et al. Triptolide suppresses ultraviolet B-enhanced sebum production by inhibiting the biosynthesis of triacylglycerol in hamster sebaceous glands in vivo and in vitro. Exp Ther Med 2017;14:361-6. [Crossref] [PubMed]
- Rijken F, Bruijnzeel PL, van Weelden H, et al. Responses of black and white skin to solar-simulating radiation: differences in DNA photodamage, infiltrating neutrophils, proteolytic enzymes induced, keratinocyte activation, and IL-10 expression. J Invest Dermatol 2004;122:1448-55. [Crossref] [PubMed]
- Latonen L, Järvinen PM, Suomela S, et al. Ultraviolet B radiation regulates cysteine-rich protein 1 in human keratinocytes. Photodermatol Photoimmunol Photomed 2010;26:70-7. [Crossref] [PubMed]
- Lin SY, Liao SL, Hong JB, et al. TERT promoter mutations in periocular carcinomas: implications of ultraviolet light in pathogenesis. Br J Ophthalmol 2016;100:274-7. [Crossref] [PubMed]
- Hussain RM, Matthews JL, Dubovy SR, et al. UV-independent p53 mutations in sebaceous carcinoma of the eyelid. Ophthalmic Plast Reconstr Surg 2014;30:392-5. [Crossref] [PubMed]
- Guo Y, Wang J, Zhou K, et al. Cytotoxic necrotizing factor 1 promotes bladder cancer angiogenesis through activating RhoC. FASEB J 2020;34:7927-40. [Crossref] [PubMed]
- Zhang T, Qian H, Hu C, et al. MiR-26a Mediates Ultraviolet B-Induced Apoptosis by Targeting Histone Methyltransferase EZH2 Depending on Myc Expression. Cell Physiol Biochem 2017;43:1188-97. [Crossref] [PubMed]
- Tetzlaff MT, Curry JL, Yin V, et al. Distinct pathways in the pathogenesis of sebaceous carcinomas implicated by differentially expressed microRNAs. JAMA Ophthalmol 2015;133:1109-16. [Crossref] [PubMed]
- DaSilva-Arnold SC, Kuo CY, Davra V, et al. ZEB2, a master regulator of the epithelial-mesenchymal transition, mediates trophoblast differentiation. Mol Hum Reprod 2019;25:61-75. [Crossref] [PubMed]
- Zhang J, Zhang H, Qin Y, et al. MicroRNA-200c-3p/ZEB2 loop plays a crucial role in the tumor progression of prostate carcinoma. Ann Transl Med 2019;7:141. [Crossref] [PubMed]
- Wu J, Cao J, Li X, et al. MicroRNA-345 functions as a tumor suppressor via targeting ZEB2 in oral squamous cell carcinoma. Arch Oral Biol 2020;116:104732. [Crossref] [PubMed]
- Yang S, Li X, Shen W, et al. MicroRNA-140 Represses Esophageal Cancer Progression via Targeting ZEB2 to Regulate Wnt/β-Catenin Pathway. J Surg Res 2021;257:267-77. [Crossref] [PubMed]
- Cicinelli MV, Kaliki S. Ocular sebaceous gland carcinoma: an update of the literature. Int Ophthalmol 2019;39:1187-97. [Crossref] [PubMed]
- Bhardwaj M, Sen S, Chosdol K, et al. Vimentin overexpression as a novel poor prognostic biomarker in eyelid sebaceous gland carcinoma. Br J Ophthalmol 2020;104:879-84. [Crossref] [PubMed]
(English Language Editor: C. Betlazar-Maseh)