GFAT1 is highly expressed in cancer stem cells of pancreatic cancer
Introduction
Pancreatic cancer (PC) is the fourth leading cause of cancer-related mortality, and the early diagnosis and effective treatment of PC remain top questions in the medical field (1). The low survival rate of PC was reported by the 2018 GLOBOCAN study based on data from 185 countries, demonstrating the closeness between the number of new cases of PC and the total number of deaths (2). The poor therapeutic outcome of PC patients not only results from late diagnosis, the degree of malignancy of the cancer, and its early metastasis, but is also attributable to the presence of a specific cell population in PC, namely cancer stem cells (CSCs), which are critical for the maintenance of tumor growth, survival, and migration, as well as distal tumor formation (3,4). Many surface markers for CSCs have been proposed in past studies, while CD133 (prominin-1) and CD44 are the most recognized and well-known CSC markers for PC (5-7). However, it is noteworthy that all currently used CSC markers are only capable of enriching CSCs, largely due to the lack of marker specificity (8-10).
Glutamine-fructose-6-phosphate transaminase 1 (GFAT1) produces uridine 5'-diphospho-N-acetyl-D-glucosamine (UDP-GlcNAc) to relate energy metabolism with post-translational protein glycosylation in the hexosamine pathway (HP) (11,12). Since GFAT1 has a strong association with glutamine in its function and has been linked to both glucose and glutamine metabolism, it is expected to play an important role in PC progression (11,12). Indeed, GFAT1 was recently reported to be highly expressed in PC (13) and hepatic cancer (14). However, the relevance of this high expression of GFAT1, especially its association with cancer stemness, has not been well defined, and is thus addressed in the current study.
In the current study, GFAT1 levels in PC were examined using a public database and were also examined in CD133+vs. CD133− PC cells and in CD44+vs. CD44− PC cells. In vivo, 2 PC cell lines, PANC-1 and HPAC, were transduced with a designed plasmid carrying a red fluorescent protein (RFP) reporter under the control of the GFAT1 promoter to allow separation of GFAT1+ cells from GFAT1− PC cells. Tumor sphere formation, tumor growth, tumor cell invasion, cell migration, and resistance to gemcitabine-induced apoptosis were assessed in GFAT1+vs. GFAT1− PC cells, showing that GFAT1 is highly expressed in CSCs in PC. We present the following article in accordance with the MDAR reporting checklist (available at https://atm.amegroups.com/article/view/10.21037/atm-22-1946/rc).
Methods
Cell culture and treatment
Human PC cell lines PANC-1 and HPAC were both purchased from American Type Culture Collection (ATCC, Rockville, MD, USA). PANC-1 and HPAC cells were cultured in Dulbecco’s Modified Eagle’s Medium (DMEM, Invitrogen, Carlsbad, CA, USA) supplemented with 10% fetal bovine serum (FBS, Sigma-Aldrich, St Louis, MO, USA) in a humidified chamber with 5% CO2 at 37 ℃. Gemcitabine (Sigma-Aldrich) was dissolved in phosphate buffered saline and applied to the culture with an end concentration of 5 µmol/L. Cells were analyzed 24 hours after gemcitabine administration.
Cell transfection and detection by flow cytometry
The PC cells were transfected with a designed plasmid. The plasmid carried an RFP reporter under the control of a GFAT1 promoter. Backbone plasmids were purchased from Clontech (Mountain View, CA, USA). The GFAT1 promoter was cloned with NheI-restriction-endonuclease-forward and EcoRII-restriction-endonuclease-reverse primers, using human genomic DNA as a template. Lipofectamine 3000 reagent (Invitrogen) was used for in vitro transfection of PC cells. Transfected cells were purified based on RFP expression by flow cytometry. Flow cytometry analysis was based on direct fluorescence of RFP and FITC from incubation of the cells with FITC-conjugated anti-CD133 (BD Bioscience, 567033) or anti-CD44 antibodies (Biolegend, 103005, Beijing, China).
Immunohistochemistry
The above transfected PC cells were stained with same anti-CD133 or anti-CD44 antibodies mentioned above, and DAPI (4',6-diamidino-2-phenylindole) for nuclei counterstain. Cells suspension was put onto a microscope slide, and taken pictures with a fluorescence microscope (Nikon, Tokyo, Japan).
Primary tumor sphere culture and cell viability assay
Matrigel™ was thawed and put on ice. To generate spheres from PC cells, 2,000–6,000 cells/well were plated in a 12-well plate. Slowly, 100 µL media was pipetted around the bottom rim of a well in the 12-well plate. Matrigel™ was solidified in a humidified incubator at 37 ℃ for 50 min. The original plates were replenished with warm media every 2–3 days. Spheres could be counted starting on day 7. The cell viability assay was performed with a specific cell counting kit-8 (CCK-8, Invitrogen).
Cell invasion assay and migration assay
GFAT1+ and GFAT− HPAC and PANC-1 cells were used for invasion and migration assay. Upper chamber of transwell plates (Millipore) were coated with growth factor reduced basement membrane Matrigel (Corning). Cells were seeded in the upper chamber (2×104/well) in 500 µL FBS-free DMEM, and lower chamber with DMEM supplemented with 10% FBS. Cells were cultured for 1 day at 37 ℃ with 5% CO2. Then cells retained in the upper chamber surface were removed, and cells migrated to the lower chamber were fixed with methanol followed by Giemsa staining (Millipore Sigma). Pictures were taken with Olympus microscope and quantified with NIH ImageJ.
Migration assay was performed with same method as invasion assay except no Matrigel coating in the transwell chamber.
TUNEL assay
A terminal deoxynucleotidyl transferase (TdT)-mediated dUTP nick end labeling (TUNEL) assay kit (R&D Biosystems, Shanghai, China) was used for TUNEL staining, and 4',6-diamidino-2-phenylindole (DAPI, Sigma-Aldrich) was used for nuclear staining.
Reverse transcription-quantitative polymerase chain reaction (RT-qPCR)
A QuantiTect SYBR Green PCR kit (Qiagen, Shanghai, China) was applied for RT-qPCR with primers designed and commercially distributed by Qiagen. Calculations of gene expression was performed using the 2−∆∆Ct method. Glyceraldehyde-3-phosphate dehydrogenase (GAPDH) was used as a housekeeping gene to calculate the relative expression levels of genes.
Statistical analysis
Statistical analysis was performed with GraphPad Prism 7 (GraphPad Software, San Diego, CA, USA). Comparisons between 2 groups were carried out with Student’s t-test. All values represent the mean ± standard deviation (SD). No data was excluded. A value of P<0.05 was considered as significant.
Results
GFAT1 is upregulated in PC
A recent study showed that GFAT1 is upregulated in PC samples (13). First, we validated this result using a public database. A public dataset (GSE62452) was analyzed using a boxplot (Figure 1A), t-statistic quantile-quantile plot (Figure 1B), mean-variance trend plot (Figure 1C), and principal components analysis (PCA) plot (Figure 1D), which confirmed that the data quality and data distribution across PC and adjacent non-PC tissue in this database were good for analysis. Next, we examined the differential genes between PC and adjacent non-PC tissue. We found that GFAT1 levels were significantly higher in PC vs. adjacent non-PC tissue (Figure 1E). These data confirmed the previous report on the high expression of GFAT1 in PC (13).
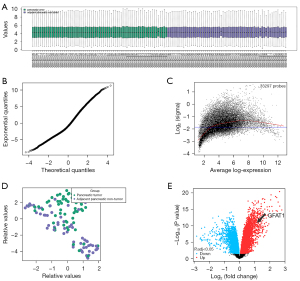
GFAT1 is highly expressed in CD133+ and CD44+ PC cells
In order to assess whether GFAT1 plays a role in PC stemness, the PC cell line PANC-1 underwent separation of CD133+ cells from CD133− cells by flow cytometry (Figure 2A). The CD133+ population is known to be enriched in CSCs in PC. We found that the CD133+ population expressed about 9-fold higher levels of GFAT1 than the CD133− population as determined by RT-qPCR (Figure 2B). Moreover, significantly higher GFAT1 expression was detected in CD133+ cells compared to CD133− cells as determined by immunocytochemistry in cytospun cells from sorting (Figure 2C). Similarly, CD44+vs. CD44− cell fractions were isolated from PANC-1 cells (Figure 2D). The CD44+ population is also known to be enriched in CSCs. We found that the CD44+ population expressed about 8-fold higher GFAT1 than the CD44− population as determined by RT-qPCR (Figure 2E). Moreover, significantly higher GFAT1 expression was detected in CD44+ cells compared with CD44− cells as determined by immunocytochemistry in cytospun cells from sorting (Figure 2F). Together, these data suggest that GFAT1 is highly expressed in CSCs in PC.
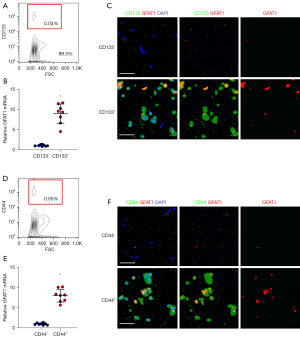
Separation of GFAT1+vs. GFAT1− PC cells with genetic labeling
In order to determine whether GFAT1 is a CSC marker for PC, we transfected PC cells with plasmids carrying an RFP reporter under the control of a GFAT1 promoter (pGFAT1-RFP) (Figure 3A). Two human PC cell lines, PANC-1 and HPAC, were transfected with the designed plasmid. Flow cytometry analysis showed that 4.6% of PANC-1 cells and 2.5% of HPAC cells showed red fluorescence after transfection. These red cells were GFAT1+ PC cells (Figure 3B). To validate the enrichment of GFAT1 in the RFP+ population, we examined GFAT1 in RFP+ and RFP− cells compared to total unsorted cells by RT-qPCR. We found that GFAT1 mRNA in RFP+ cells was increased by about 10-fold compared to the total unsorted cells in either examined cell line, while GFAT1 mRNA in RFP− cells was decreased by more than 80% compared to the total unsorted cells in either examined cell line (Figure 3C). These data confirmed that the designed plasmids were capable of sorting GFAT1+ cells from GFAT1− cells.
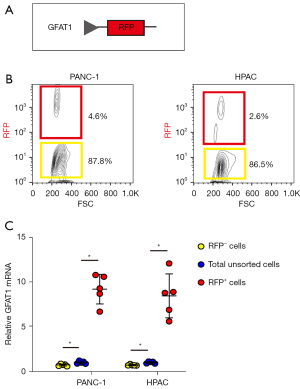
GFAT1+ PC cells form more tumor spheres in vitro
We then studied the CSC properties of GFAT1+ cells compared to GFAT1− cells. First, GFAT1− and GFAT1+ cells from either examined cell line were used in a tumor sphere formation assay. We detected significantly more tumor spheres formed by GFAT1+ cells compared to GFAT1− cells in either cell examined line, shown by representative images (Figure 4A) and by quantification (Figure 4B).
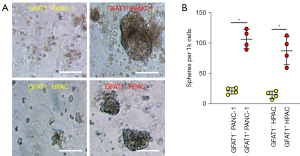
GFAT1+ PC cells have higher invasive and migratory potential in vitro
Next, GFAT1− and GFAT1+ cells from either examined cell line were used in a cell invasion assay. Significantly higher invasiveness was detected in GFAT1+ cells compared to GFAT1− cells, as shown by quantification (Figure 5A) and by representative images (Figure 5B). Moreover, in a cell migration assay, significantly higher migratory potential was detected in GFAT1+ cells compared to GFAT1− cells, as shown by quantification (Figure 5C) and by representative images (Figure 5D).
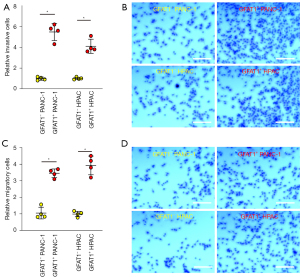
GFAT1+ PC cells are more resistant to gemcitabine
Finally, we examined the difference in the resistance of GFAT1−vs. GFAT1+ cells to chemotherapeutic drugs. Gemcitabine is the most commonly used chemotherapeutic drug for PC and was thus used for the experiment. GFAT1+ cells from either examined cell line exhibited significantly better viability in the CCK-8 assay compared to control GFAT1− cells (Figure 6A). Moreover, the resistance of GFAT1+ cells to gemcitabine seemingly resulted from reduced cell apoptosis, as determined by the TUNEL assay (Figure 6B,6C). Together, GFAT1+ PC cells demonstrate CSC properties.
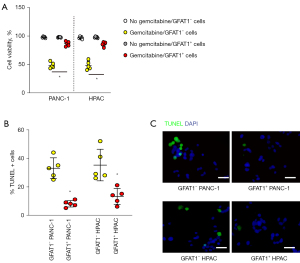
Discussion
Energy metabolism is reprogrammed in cancer, in which preferential utilization of glycolysis instead of oxidative phosphorylation by cancer cells generates energy without the need for oxygen. This is called aerobic glycolysis and this process uses a large amount of glutamines that significantly changes metabolism for both glucose and glutamine. The hexosamine biosynthesis pathway (HBP) plays a critical role in the consummation of glucose and glutamine to generate UDP-GlcNAc, which is profoundly involved in the classical glycosylation and O-GlcNAcylation process, requiring the participation of GFAT1 for HBP metabolism. Thus, GFAT1 alteration in cancer may be important for cancer energy metabolism reprogramming. Specifically, high GFAT1 expression has been demonstrated in pancreatic ductal adenocarcinoma (PDAC) (12,13). Nevertheless, to the best of our knowledge, the connection between GFAT1 and PC stemness has not been demonstrated in previous studies.
CD133 and CD44 have been used as CSC markers. However, all currently used CSC markers lack specificity. Hence, additional CSC markers are needed to further improve the purification of CSCs as well as their characterization in PC. In the current study, a set of gold standards for CSC identification were used, including tumor sphere formation, tumor cell invasiveness and migratory potential, and chemoresistance. We found that CSCs may be predominantly enriched in the GFAT1+ fraction. In other words, GFAT1 is highly expressed in the CSC population in PC.
In this study, we used 2 commonly used human PC cell lines. While PANC-1 cells are very aggressive and poorly differentiated, HPAC cells are less aggressive and modestly differentiated. Little difference was detected between the 2 cell lines for the GFAT1 studies, suggesting that GFAT1 may be a common CSC marker for different types of PDAC.
Our study did have some limitations. For example, GFAT1-overexpressing PC cells and GFAT1-depleted PC cells may be implanted into mice to examine their potential in tumor formation in vivo in future studies.
To summarize, our study demonstrates that GFAT1 may be used as a novel CSC marker in PC. Its contribution to tumor cell stemness and invasiveness warrants further studies at the molecular level.
Acknowledgments
Funding: This study was supported by National Natural Science Foundation of China (81972257) and Natural Science Foundation of Shanghai (19ZR1409700).
Footnote
Reporting Checklist: The authors have completed the MDAR reporting checklist. Available at https://atm.amegroups.com/article/view/10.21037/atm-22-1946/rc
Data Sharing Statement: Available at https://atm.amegroups.com/article/view/10.21037/atm-22-1946/dss
Conflicts of Interest: All authors have completed the ICMJE uniform disclosure form (available at https://atm.amegroups.com/article/view/10.21037/atm-22-1946/coif). The authors have no conflicts of interest to declare.
Ethical Statement: The authors are accountable for all aspects of the work in ensuring that questions related to the accuracy or integrity of any part of the work are appropriately investigated and resolved.
Open Access Statement: This is an Open Access article distributed in accordance with the Creative Commons Attribution-NonCommercial-NoDerivs 4.0 International License (CC BY-NC-ND 4.0), which permits the non-commercial replication and distribution of the article with the strict proviso that no changes or edits are made and the original work is properly cited (including links to both the formal publication through the relevant DOI and the license). See: https://creativecommons.org/licenses/by-nc-nd/4.0/.
References
- Kalloger SE, Karasinska JM, Warren C, et al. Advancing the Care of Pancreatic Cancer Patients: Moving Beyond Just Tumour Tissue. Biomark Insights 2021;16:11772719211049852. [Crossref] [PubMed]
- Siegel RL, Miller KD, Jemal A. Cancer Statistics, 2017. CA Cancer J Clin 2017;67:7-30. [Crossref] [PubMed]
- Patil K, Khan FB, Akhtar S, et al. The plasticity of pancreatic cancer stem cells: implications in therapeutic resistance. Cancer Metastasis Rev 2021;40:691-720. [Crossref] [PubMed]
- Mortezaee K. Enriched cancer stem cells, dense stroma, and cold immunity: Interrelated events in pancreatic cancer. J Biochem Mol Toxicol 2021;35:e22708. [Crossref] [PubMed]
- Gzil A, Zarębska I, Bursiewicz W, et al. Markers of pancreatic cancer stem cells and their clinical and therapeutic implications. Mol Biol Rep 2019;46:6629-45. [Crossref] [PubMed]
- Perusina Lanfranca M, Thompson JK, Bednar F, et al. Metabolism and epigenetics of pancreatic cancer stem cells. Semin Cancer Biol 2019;57:19-26. [Crossref] [PubMed]
- Raj D, Aicher A, Heeschen C. Concise Review: Stem Cells in Pancreatic Cancer: From Concept to Translation. Stem Cells 2015;33:2893-902. [Crossref] [PubMed]
- Askan G, Sahin IH, Chou JF, et al. Pancreatic cancer stem cells may define tumor stroma characteristics and recurrence patterns in pancreatic ductal adenocarcinoma. BMC Cancer 2021;21:385. [Crossref] [PubMed]
- Liu H, Tao H, Wang H, et al. Doxycycline Inhibits Cancer Stem Cell-Like Properties via PAR1/FAK/PI3K/AKT Pathway in Pancreatic Cancer. Front Oncol 2021;10:619317. [Crossref] [PubMed]
- Nimmakayala RK, Leon F, Rachagani S, et al. Metabolic programming of distinct cancer stem cells promotes metastasis of pancreatic ductal adenocarcinoma. Oncogene 2021;40:215-31. [Crossref] [PubMed]
- Ruegenberg S, Mayr FAMC, Atanassov I, et al. Protein kinase A controls the hexosamine pathway by tuning the feedback inhibition of GFAT-1. Nat Commun 2021;12:2176. [Crossref] [PubMed]
- Ruegenberg S, Horn M, Pichlo C, et al. Loss of GFAT-1 feedback regulation activates the hexosamine pathway that modulates protein homeostasis. Nat Commun 2020;11:687. [Crossref] [PubMed]
- Yang C, Peng P, Li L, et al. High expression of GFAT1 predicts poor prognosis in patients with pancreatic cancer. Sci Rep 2016;6:39044. [Crossref] [PubMed]
- Li L, Shao M, Peng P, et al. High expression of GFAT1 predicts unfavorable prognosis in patients with hepatocellular carcinoma. Oncotarget 2017;8:19205-17. [Crossref] [PubMed]
(English Language Editor: C. Betlazar-Maseh)