Fluoride-coated high-purity magnesium cage promotes bone fusion in goat models
Introduction
Complete decompression of the spinal cord and nerve roots is crucial to successful surgical treatment of cervical spondylosis, and intervertebral bone graft fusion is required to reconstruct height and stability of the space to obtain good long-term effects. Since Smith and Robinson described anterior cervical discectomy and bone graft fusion (ACDF) in 1958 (1,2), autogenous bone has been the “gold standard” for clinical interbody fusion; however, common complications of donor site, such as pain, bleeding, fracture, and nerve injury have limited widespread use of the iliac crest as an intervertebral bone graft material. The cervical fusion device made by polyether ether ketone (PEEK), which is widely used in clinical practice, can cause a strong non-specific inflammatory response in the surrounding bone tissue, triggering fibroblast and macrophage infiltration as well as bone resorption and osteolysis, mimicking the local reaction caused by ultra-high molecular weight polyethylene powder after joint replacement.
Magnesium (Mg) has attracted attention in biodegradable orthopedic implant material research, displaying characteristics of good biocompatibility, bioactivity, and desirable elastic modulus similar to bone (3). Success has been reported in high-purity Mg (HP-Mg) (99.99 wt.%) screw in in vivo studies with femoral intracondylar fractured rabbit models (4) and HP-Mg interference screws can promote fibrocartilaginous entheses regeneration in the anterior cruciate ligament reconstruction rabbit model (5). However, the results of in vivo ACDF experiments on animal models of Mg-based cervical cages have been unsatisfactory (6,7). Potential reasons include that co-implantation of titanium and Mg accelerates degradation of Mg cages, and hydrogen and excessively increasing pH value produced by rapid Mg corrosion negatively influence surrounding tissues healing. Recently, Guo et al. claimed that they achieved histological fusion of HP-Mg cages in a goat model, although total fusion area was less than 30% (8).
Coating is an effective way to regulate degradation rate of Mg fusion cages (9). Some studies have suggested that fluoride-coated Mg alloy could improve corrosion resistance and promote osteogenic differentiation (10,11). In this study, we tried to improve interbody fusion of HP-Mg cages by coating fluoride on HP-Mg (F-HP-Mg). We present the following article in accordance with the ARRIVE reporting checklist (available at https://atm.amegroups.com/article/view/10.21037/atm-22-2098/rc).
Methods
Sample and materials
The HP-Mg cages were built with 99.982 wt.% Mg (Suzhou Origin Medical Technology, Jiangsu, China), 0.0178 wt.% silicon (Si), <0.001 wt.% iron (Fe), and <0.001 wt.% aluminum (Al) in the State Key Laboratory of Metal Matrix Composites of Shanghai Jiao Tong University. Cages were designed and fabricated for ACDF and possessed dimension parameters similar to PEEK cage (Medtronic Cornerstone-SR PEEK; Medtronic, Parkway Minneapolis, MN, USA), which was modified with specification of 14 mm × 11 mm × 4 mm and a 4-degree wedge angle.
The F-HP-Mg cages (mainly composed of MgO and MgF2) were made through immersion of HP-Mg cages in a hydrofluoric acid solution (9). Untreated HP-Mg cages of the same size were used as controls in goat models. An Mg sheet (size 12 mm × 10 mm × 1 mm) was prepared for in vitro testing.
Surface modification
The fluoridation process was operated in fume hood hydrofluoric acid. The Mg sheet samples that had been polished and ultrasonically cleaned and dried were removed and placed into a plastic beaker reaction vessel with a plastic agitator at the bottom and a suspended yarn net. The samples were placed into each beaker on the yarn net, and then 250 mL 40% hydrofluoric acid reagent was poured into the beaker. It was observed that all Mg samples were immersed in liquid. The beaker was then sealed with plastic wrap and the opening was secured with a rubber band. The beaker was placed on the magnetic stirrer at low speed, with the sample fully immersed in the acid flow at room temperature for 96 hours. Finally, all samples were rinsed in ethanol and dried. The untreated pure Mg group was used as the control.
Immersion test
The method of the immersion test was employed as described in our previous study, which was in accordance to ASTM-G31-72 (12). The F-HP-Mg and HP-Mg sheets were incubated in PBS (pH =7.40, 1 cm2/20 mL) separately at 37 ℃ for 2 weeks, with 3 samples in each group. The pH value of PBS was measured across time by pH meter (FE20; Mettler Toledo, Columbus, OH, USA). After 14 days, 1 side of the sheets was ultrasonically cleaned with 100% ethanol, and the other side was rinsed with 180 g/L chromic acid solution and distilled water, air dried, and weighed.
Animals and surgery
Experiments were performed under a project license (No. SYXK2011-0128) granted by ethics board of Shanghai Jiao Tong University Affiliated Sixth People’s Hospital, in compliance with institutional guidelines for the care and use of animals. A protocol was prepared before the study without registration. Goats have similar cervical spines to human, especially at the C2-C3 level, which makes them ideal experimental models to test cervical spine fusion (13,14). A total of 10 healthy 1.5-year-old goats weighing 38.78±3.72 kg (range, 33 to 46 kg) were purchased from Shanghai Jiagan Biotechnology Co., Ltd. (Shanghai, China) and given adequate access to sterilized water and food. All animals were randomly assigned to either the experimental or control group for assessment at post-operative 4, 12, and 24 weeks.
Goats were anesthetized with 2.5% pentobarbital sodium, followed by ACDF surgery at C2-C3 segment, which was implanted with a HP-Mg or F-HP-Mg cage, a titanium plate, and fixed with screws. Cage holes were filled with autogenous bone from the anterior spinous process of cervical vertebra.
Radiographical analysis
Anteroposterior and lateral radiographs were performed between post-surgery and sacrifice to determine implant location, gas accumulation, disc space height (DSH), and implant settlement. The DSH is the distance between upper and lower anterior edge of the vertebral body fixed by screws of the titanium plate.
Micro-CT scan and three-dimensional (3D) reconstruction were performed on sacrificed specimens, and quantitative analysis of the fusion device was carried out. Interbody fusion could be scored with CT images (15), as follows: level 0, no new bone or even vertebral endplate destruction; level 1, new bone formation but not continuous between C2/3; and levels 2 and 3, continuous bridging new bone and comprises <30% and >30% of fusion area, respectively.
Histological analysis
Sacrificed goat vertebrae were fixed in paraformaldehyde, dehydrated in acetone, embedded in methyl methacrylate, cut into 300 µm sections and ground to 20 µm. Staining was performed on 4 sections with hematoxylin-eosin (HE), toluidine blue, Van Gieson, and Masson to determine osteogenesis and inflammation.
Statistical analysis
No data was excluded. Data were presented as mean ± standard deviation (SD). A two-tailed t-test was used to compare CT fusion scores between the HP-Mg cage group and F-HP-Mg cage group. The two-way analysis of variance (ANOVA) was used to compare weight of cages, Mg concentrations, alanine aminotransferase (ALT), aspartate aminotransferase (AST), and creatinine (CREA). Statistical analysis was conducted with the software SPSS 20 (IBM Corp., Armonk, NY, USA), and P<0.05 was statistically significant.
Results
In vitro test
Gross characteristics
The MgF2 films formed in 40% hydrofluoric acid after 96 hours appeared dark black. The MgF2 layer did not change macro sizes of samples (Figure 1A). No significant difference was detected in weight between the two groups.
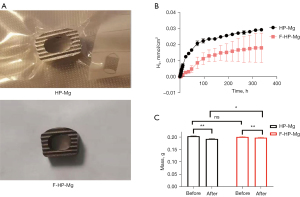
Hydrogen release
Hydrogen release in the buffer was measured for 336 hours. With the prolongation of immersing time, hydrogen (H2) release was increased from both HP-Mg and F-HP-Mg cages (Figure 1B). The H2 concentration was significantly higher in HP-Mg cages compared to F-HP-Mg cages.
Weight loss
Weight of HP-Mg cages and F-HP-Mg cages was similar before the weight loss experiment. After rinsing with 180 g/L chromic acid, both the HP-Mg cages and F-HP-Mg cages lost a significant volume of weight (Figure 1C). The F-HP-Mg cages were significantly heavier than the HP-Mg cages after chromic acid, indicating that the former were more resistant to corrosion than the latter.
In vivo test
Radiological findings
The interbody fusion was evaluated with 64-slice-spiral CT. The DSH measurement is shown in Figure 2A. At 4 weeks postoperatively, there was no significant difference in DSH, but a significant difference could be observed in CT images between the two groups, with a small amount of gas observed in the anterior tissue of the cervical spine in HP-Mg cages (Figure 2B). We found that HP-Mg cages caused damage to the upper and lower endplates and surrounding bone, forming cavities at 12 weeks postoperatively. The cavity was further enlarged at 24 weeks, and the autogenous bone filling in the middle hole of the cage was completely lost (Figure 2C).
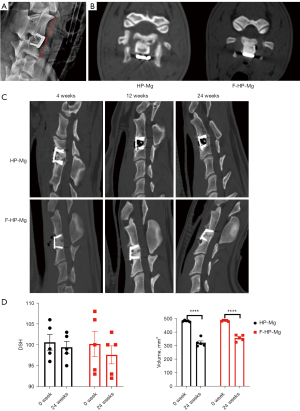
No significant DSH difference and implant settlement was found in HP-Mg cages and F-HP-Mg cages at post-operative 4, 12, and 24 weeks (Figure 2D). The remaining volume of both kinds of cages was significantly lower after 24 weeks implantation, while not significantly different between HP-Mg and F-HP-Mg cages (Figure 2D).
Histological results
Bone fusion was observed in F-HP-Mg cages with a continuous bone found between endplates (Figure 3A-3E). However, osteolytic phenomenon with destroyed bone tissues was found in HP-Mg cages.
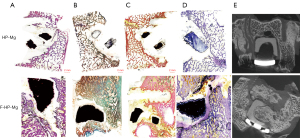
Mg concentrations, ALT, AST, and CREA in serum
Serum Mg2+ concentration did not markedly change in both groups (Figure 4A). No complications related to hypermagnesemia were found. Serum ALT level was similar between the 2 goat groups (Figure 4B), while AST level was statistically higher in the HP-Mg group compared to the F-HP-Mg group at postoperative weeks 12 and 24 (Figure 4C). Serum CREA was not statistically different (Figure 4D).
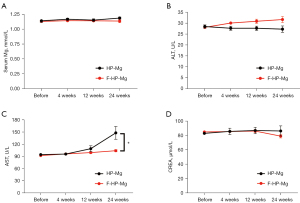
Fusion results
During the experiment, CT fusion score of F-HP-Mg cages increased over time, but the score of HP-Mg cage decreased at weeks 12 and 24. The fusion score of segments with F-HP-Mg cages was remarkably higher than HP-Mg cages at 24 weeks, with HP-Mg as (0.2±0.45) and F-HP-Mg as (2.8±0.45) (P<0.01) (Figure 5A). After 24 weeks, new bone tissue between endplates in graft segments was found in CT 3D reconstruction images (Figure 5B), while no fusion with a cage was observed (Figure 5C).
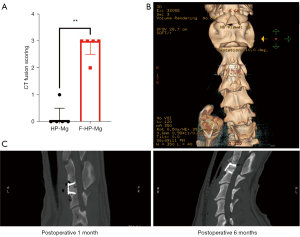
Discussion
Guo et al. reported the use of an HP-Mg cage to perform ACDF surgery on a goat model, and achieved the first successful interbody fusion, indicating the possibility of Mg-based cage application in ACDF (8). However, this study also reported that the total area of interbody fusion was less than 30%. There was a 300–400 µm gap between the cage and the bone tissue, which was filled by hyperplastic fibrous tissue. The bone was not tightly bound to the cage interface.
The results of this experiment revealed that in the experimental group using an HP-Mg cage, because the cage caused osteolysis to the surrounding bone tissue, the CT sagittal view showed the formation of a cavity around the cage, and the pathological section showed that there was a space between the cage and the bone tissue. With infiltration of fibrous tissue, the effect of vertebral fusion was not ideal. However, after the surface modification of HP-Mg with hydrofluoric acid, the degradation rate of HP-Mg slowed down, continuous bone tissue formation was seen on a CT sagittal view, the gap between the cage and the bone tissue was reduced, and new trabecular bone formation was seen surrounding the cage.
For the cage material, we chose to use HP-Mg (99.98 wt.%) for 2 reasons: first, Mg-based implants had been revealed as osteoinductive in in vivo studies in unstressed bones, leading to successful fusion. The mechanism may be related to the release of Mg2+ from Mg-based implants in neuronal calcitonin gene-related polypeptide (CGRP)-mediated osteogenic differentiation, which plays an important role in induction of osteogenesis (16). Secondly, compared with Mg alloys, HP-Mg has better corrosion resistance. Preliminary research shows that the higher the purity, the stronger the corrosion resistance, and the slower the degradation rate. This experiment required Mg-based cages in the vertebral column which takes about 6 months, so the purity of HP-Mg needs to be improved as much as possible to obtain sufficient support time.
It has been shown that Mg-based materials immersed in hydrofluoric acid can form a fluoride coating on the metal surface (10), which can effectively reduce the degradation rate of Mg, thereby slowing the release rate of Mg2+ and increased deposition of localized calcium and phosphate. The results of this study showed that fluoride-coated Mg alloy had good osteogenic activity and biocompatibility, and the fluoride coating significantly upregulated expressions of type I collagen and bone morphogenetic protein 2 (BMP-2). Therefore, in this experiment, we modified the surface of the HP-Mg cage in hydrofluoric acid to form a MgF2 coating, and the thickness of the MgF2 coating depended on the reaction time of the MgF2 cage in hydrofluoric acid, which we continued for 96 hours.
The results of this experiment showed that after the HP-Mg cage was implanted into the goat cervical spine, galvanic corrosion occurred with the fixed anterior cervical vertebrae titanium plate, which accelerated degradation of the HP-Mg cage and damaged the upper and lower vertebral body endplates. As a result, the fusion failed. Despite this, the prevailing view is that locally high Mg ion concentrations do not produce significant cytotoxicity and are safe for topical application as a degradable material (17). However, the accelerated corrosion rate of Mg can lead to loss of mechanical properties of orthopedic implants and failure (18). If Mg cages are coated with MgF2, the degradation rate can be reduced, galvanic corrosion can be isolated, and osteogenic activity and biocompatibility can be improved.
In this study, serological tests were performed at 4, 12, and 24 weeks after the operation. Serum levels of Mg2+, AST, ALT, and CREA were examined, and no obvious liver and kidney function damage was found. In addition, studies involving pathological sectioning on animal organs found no obvious lesions, which supports the good biosafety of HP-Mg fusion in vivo. Excessive Mg ions can be excreted in urine and not absorbed by the body.
The results of this study suggest that the application of the HP-Mg interbody fusion cage with MgF2 coating can achieve cervical vertebral body fusion, and thus may be used in ACDF surgery. However, more in vivo and in vitro experiments and longer observation times are needed. In addition, the fusion effect of the HP-Mg cage group in our study was not satisfactory, the rapid degradation of Mg2+ would destroy the bone of the upper and lower vertebral body endplates and form a cavity in the intervertebral space, although it was possible to form a bone bridge connection at the anterior and posterior borders of the vertebral body. Partial fusion was achieved, which was different from the related research results of using the same material to make cages (8), and might be the result of difference of surgical operation, animal breeding environment, and other factors.
The in vivo experiment of cervical degradable cage is time consuming, requires complicated operation, and has big individual differences. Previous researchers used cages with β-calcium triphosphate as the core and polylactic acid as the shell to conduct a phase III clinical trial of cervical interbody fusion (19). After an average follow-up of 27 months, the fusion rate was 96% (26/27). However, in another study, the results of cages with the same specifications were disappointing. Although the experiment planned to include 50 participants, it had to terminate early due to the high dislocation and complication rates after recruiting only 33 participants (20). Therefore, animal experiments should be carried out to evaluate safety of degradable cages before the implementation of clinical trials.
Conclusions
The HP-Mg cage with fluoride coating can successfully achieve histological fusion in the cervical intervertebral space of goat: that is, the new bone is connected between the upper and lower vertebral bodies through the middle hole of the cage. It is worth noting that the fusion area of the HP-Mg cage with fluoride coating was greater than 30%, the fusion results of the fluoride coated group at 24 weeks were significantly better than that of the uncoated group, and the degradation rate was also significantly lower than that of the uncoated group. The F-HP-Mg cage can play a stable supporting role in the early post-implantation period, and then degrade steadily during the observation period. The cage has sufficient mechanical support for intervertebral applications. In addition, the F-HP-Mg cage has good biocompatibility, no adverse reactions for vital organs. More studies are needed to evaluate the long-term fusion effect and degradation behavior of this cage, which is critical for potential use in ACDF in the future.
Acknowledgments
Funding: This study was supported by grants from the National Natural Science Foundation of China (No. 81672217).
Footnote
Reporting Checklist: The authors have completed the ARRIVE reporting checklist. Available at https://atm.amegroups.com/article/view/10.21037/atm-22-2098/rc
Data Sharing Statement: Available at https://atm.amegroups.com/article/view/10.21037/atm-22-2098/dss
Conflicts of Interest: All authors have completed the ICMJE uniform disclosure form (available at https://atm.amegroups.com/article/view/10.21037/atm-22-2098/coif). The authors have no conflicts of interest to declare.
Ethical Statement: The authors are accountable for all aspects of the work in ensuring that questions related to the accuracy or integrity of any part of the work are appropriately investigated and resolved. Experiments were performed under a project license (No. SYXK2011-0128) granted by ethics board of Shanghai Jiao Tong University Affiliated Sixth People’s Hospital, in compliance with institutional guidelines for the care and use of animals.
Open Access Statement: This is an Open Access article distributed in accordance with the Creative Commons Attribution-NonCommercial-NoDerivs 4.0 International License (CC BY-NC-ND 4.0), which permits the non-commercial replication and distribution of the article with the strict proviso that no changes or edits are made and the original work is properly cited (including links to both the formal publication through the relevant DOI and the license). See: https://creativecommons.org/licenses/by-nc-nd/4.0/.
References
- Smith GW, Robinson RA. The treatment of certain cervical-spine disorders by anterior removal of the intervertebral disc and interbody fusion. J Bone Joint Surg Am 1958;40-A:607-24. [Crossref] [PubMed]
- Cloward RB. The anterior approach for removal of ruptured cervical disks. J Neurosurg 1958;15:602-17. [Crossref] [PubMed]
- Witte F. The history of biodegradable magnesium implants: a review. Acta Biomater 2010;6:1680-92. [Crossref] [PubMed]
- Han P, Cheng P, Zhang S, et al. In vitro and in vivo studies on the degradation of high-purity Mg (99.99wt.%) screw with femoral intracondylar fractured rabbit model. Biomaterials 2015;64:57-69. [Crossref] [PubMed]
- Cheng P, Han P, Zhao C, et al. High-purity magnesium interference screws promote fibrocartilaginous entheses regeneration in the anterior cruciate ligament reconstruction rabbit model via accumulation of BMP-2 and VEGF. Biomaterials 2016;81:14-26. [Crossref] [PubMed]
- Zhang F, Xu H, Wang H, et al. Quantitative analysis of near-implant magnesium accumulation for a Si-containing coated AZ31 cage from a goat cervical spine fusion model. BMC Musculoskelet Disord 2018;19:105. [Crossref] [PubMed]
- Xu H, Zhang F, Wang H, et al. Evaluation of a Porous Bioabsorbable Interbody Mg-Zn Alloy Cage in a Goat Cervical Spine Model. Biomed Res Int 2018;2018:7961509. [Crossref] [PubMed]
- Guo X, Xu H, Zhang F, et al. Bioabsorbable high-purity magnesium interbody cage: degradation, interbody fusion, and biocompatibility from a goat cervical spine model. Ann Transl Med 2020;8:1054. [Crossref] [PubMed]
- Van Eps JL, Fernandez-Moure JS, Cabrera FJ, et al. Improved Posterolateral Lumbar Spinal Fusion Using a Biomimetic, Nanocomposite Scaffold Augmented by Autologous Platelet-Rich Plasma. Front Bioeng Biotechnol 2021;9:622099. [Crossref] [PubMed]
- Sun W, Zhang G, Tan L, et al. The fluoride coated AZ31B magnesium alloy improves corrosion resistance and stimulates bone formation in rabbit model. Mater Sci Eng C Mater Biol Appl 2016;63:506-11. [Crossref] [PubMed]
- Dong J, Tümer N, Putra NE, et al. Extrusion-based 3D printed magnesium scaffolds with multifunctional MgF2 and MgF2-CaP coatings. Biomater Sci 2021;9:7159-82. [Crossref] [PubMed]
- Lou J, Sun Y, Chen Y, et al. Effects of MgF2 coating on the biodegradation and biological properties of magnesium. Surface and Coatings Technology 2021;422:127552. [Crossref]
- Kandziora F, Pflugmacher R, Scholz M, et al. Comparison between sheep and human cervical spines: an anatomic, radiographic, bone mineral density, and biomechanical study. Spine (Phila Pa 1976) 2001;26:1028-37. [Crossref] [PubMed]
- Wang L, Wang Y, Shi L, et al. Can the sheep model fully represent the human model for the functional evaluation of cervical interbody fusion cages?. Biomech Model Mechanobiol 2019;18:607-16. [Crossref] [PubMed]
- Goldschlager T, Rosenfeld JV, Ghosh P, et al. Cervical interbody fusion is enhanced by allogeneic mesenchymal precursor cells in an ovine model. Spine (Phila Pa 1976) 2011;36:615-23. [Crossref] [PubMed]
- Zhang Y, Xu J, Ruan YC, et al. Implant-derived magnesium induces local neuronal production of CGRP to improve bone-fracture healing in rats. Nat Med 2016;22:1160-9. [Crossref] [PubMed]
- Wang J, Witte F, Xi T, et al. Recommendation for modifying current cytotoxicity testing standards for biodegradable magnesium-based materials. Acta Biomater 2015;21:237-49. [Crossref] [PubMed]
- Hou P, Han P, Zhao C, et al. Accelerating Corrosion of Pure Magnesium Co-implanted with Titanium in Vivo. Sci Rep 2017;7:41924. [Crossref] [PubMed]
- Debusscher F, Aunoble S, Alsawad Y, et al. Anterior cervical fusion with a bio-resorbable composite cage (beta TCP-PLLA): clinical and radiological results from a prospective study on 20 patients. Eur Spine J 2009;18:1314-20. [Crossref] [PubMed]
- Brenke C, Kindling S, Scharf J, et al. Short-term experience with a new absorbable composite cage (β-tricalcium phosphate-polylactic acid) in patients after stand-alone anterior cervical discectomy and fusion. Spine (Phila Pa 1976) 2013;38:E635-40. [Crossref] [PubMed]