This article has an erratum available at: http://dx.doi.org/10.21037/atm-2024-35 the article has been update on 2024-10-08 at here.
Candesartan induces tumor vascular normalization to improve the efficacy of radiotherapy in the therapeutic window
Introduction
Radiotherapy (RT), which is one of the most common and effective methods for treating cancer, has been administered to about 70–80% of tumor patients at different stages of treatment, and is essential for the comprehensive treatment of tumors (1,2). However, in RT, there is a curative-effect bottleneck that is difficult to breakthrough due to the radiation resistance of solid tumors (3). A Clinical study has confirmed that the oxygen content of tumor cells has a great effect on the killing effect of radiation (4). This is because the high-energy ionizing radiation generated during RT can produce reactive oxygen species (ROS) in the aerobic microenvironment, which in turn damage the normal structure of tumor deoxyribonucleic acid (DNA) and cause tumor cell death (4,5). Thus, the therapeutic effect of RT is positively correlated with the partial pressure of oxygen inside the solid tumor.
The current clinical cause of RT resistance is that tumor cells are located in a state of hypoxia for a long time, which affects the curative effect of radiation (6). The main reasons for the hypoxic state are immature new blood vessels and the tumor extracellular matrix in the tissues (7). The morphology and structure of tumor neovascularization are mostly abnormal, and manifest as curling, expansion, interlacing, different diameters, and increased branches. The density of the blood vessels is abundant; however, most tumor neovascularization is malformed, ineffective, and unevenly distributed (6,7). About 50% of tumor neovascularization do not possess the function of normal blood vessels, and the blood flow is locally stagnated or disordered, preventing them from effectively delivering oxygen (8). Additionally, the dense extracellular matrix of the tumor tissue significantly increases the interstitial fluid pressure (IFP) inside the tumor (9), which causes the tumor neovascularization passing through the matrix to be squeezed, resulting in the heterogeneity of blood flow distribution. It further reduces the total perfusion in tumor neovascularization, resulting in a reduction in the amount of oxygen reaching the deep tissues of the tumor, aggravating the hypoxia state of tumor tissues, and affecting the efficacy of RT and chemotherapy. Researchers have proposed the use of anti-tumor angiogenesis to achieve the goal of “starving the tumor” (10). However, further research in this area needs to be conducted and significant therapeutic effects have not yet been achieved. Even the local microenvironment is more hypoxic due to the vascular blocking drugs (11), which accelerate the growth of new blood vessels and tumors.
Tumor vascular normalization could improve the structure of tumor blood vessel wall and the function of peripheral cells, making the tumor microenvironment tend to be normal and increasing the oxygen content in the tumor. Thus, it sensitizes the tumor to radiotherapy. In recent years, tumor vascular normalization has been widely studied as a new type of tumor treatment (12,13). Combined with chemotherapy drugs or RT, the reasonable application of certain anti-angiogenetic drugs, chemotherapy drugs, or Chinese medicine ingredients, could induce tumor vessels to “normalize”, and thus temporarily improve tumor vascular system function, reduce tumor tissue hypoxia, enhance oxygenation, and promote the therapeutic effect (11,13). As Angiotensin II (AngII) is known to stimulate neovascularization in the tumor microenvironment, the inhibition of tumor-induced neovascularization has been considered a possible mechanism for achieving these effects (14).
Angiotensin receptor blockers (ARBs) have been widely used in the treatment of cardiovascular diseases and have considerable potential in the treatment of tumors (15). ARBs have been found to affect tumor growth and metastasis directly and indirectly by reshaping the tumor microenvironment (16,17). Studies have shown that ARBs reduce the production of matrix collagen and hyaluronic acid by affecting the AngII/AT1R (angiotensin II type 1 receptor) signaling pathway, thereby reducing IFP and increasing vascular perfusion, which in turn increases the infusion of anti-tumor drugs in tumor tissues and increases the oxygen content of tumor tissues (9,17). Further, the expression of vascular endothelial growth factor (VEGF) in vivo is decreased after ARB treatment, which reduces immature blood vessels to reduce tumor microvessel density, induces tumor vessels to “normalize”, and temporarily improves tumor vascular system function (18). Subsequently, reduced hypoxia and enhanced oxygenation improve the sensitization effect of tumor RT and chemotherapy.
Our research team investigated the clinical data of 381 patients with gastric malignant tumors and hypertension in the early stage, and found that angiotensin-converting enzyme inhibitors (ACEI)/ARBs treatment significantly increased the efficacy of 1st-line chemotherapy (19). Additionally, a recent clinical study showed that the recurrence rate of prostate cancer patients receiving ACEIs/ARBs after adjuvant RT was significantly reduced, especially among patients with hypertension (20). However, there are also problems, such as a narrow treatment time window and the aggravation of hypoxia in tumor tissues, which make it difficult for it to be widely used in clinical practice (13,21). This study further verified that candesartan, an ARB drug, promotes the temporary normalization of tumor neovascularization and improves the hypoxic environment of tumor tissues to enhance the efficacy of RT. Further, we confirmed the treatment window for tumor vascular normalization, and found that if administered during this period, RT has an enhancing anti-tumor effect. We present the following article in accordance with the ARRIVE reporting checklist (available at https://atm.amegroups.com/article/view/10.21037/atm-22-2108/rc).
Methods
Materials
Candesartan was obtained from Adamas Co., Ltd. (Shanghai, China). Dulbecco’s modified Eagle medium and fetal bovine serum were purchased from Gibco BRL (Gaithersburg, USA). Dimethyl sulfoxide was obtained from Sigma Aldrich (St. Louis, USA).
Cells and animals
The MDA-MB-231 cells were purchased from the Type Culture Collection of the Chinese Academy of Sciences (Shanghai, China). BALB/c female nude mice (weighing 20±2 g) were supplied by the Laboratory Animal Center of Zhejiang University. Animal experiments were performed under a project license (No. ZJU20210092) granted by laboratory animal welfare and ethics committee of Zhejiang University. All the experiments were performed in accordance with the guidelines for the care and use of animals established by Zhejiang University. A protocol was prepared before the study without registration.
Determination of the normalization window of tumor vessels
The BALB/c female nude mice were subcutaneously injected with breast cancer MDA-MB-231 cells into the right axillary vein, and the needle was subcutaneously inserted about 2 cm; the dose was 3×106/100 µL/mouse. When the tumor grew to 80–100 mm3, the animals were randomly divided into the following groups: (I) the control group (normal saline); and (II) the candesartan group (20 mg/kg, according to the literature which converted from clinical doses) (18,19). Depending on the group, the mice were given intravenous injections once every other day. The administration cycle was 14 days.
In each group (n=12), 3 the nude mice were respectively injected with Fluorescein Isothiocyanate (FITC)-conjugated lectin at a dose of 10 mg/kg on the 3rd, 6th, 9th, and 12th day. Next, the tumor tissue was collected and frozen sections prepared. Anti-CD31 antibody (1:500) was used to label the microvessels in the frozen sections, and the corresponding fluorescently labeled secondary antibody developed color. The fluorescence intensity of FITC in the CD31 positive area was calculated using Image J software to determine the perfusion of the tumor vessels (22).
On the 3rd, 6th, 9th, and 12th day, 3 nude mice were taken from each group, and the tumor tissues were cut out after sacrifice. The tumor tissues were fixed in formalin and sliced to a thickness of 20 µm. CD31 and α smooth muscle actin (α-SMA) staining were used to show tumor vessel density and the tumor perivascular cells, respectively. Next, hypoxia-inducible factor 1α (HIF-1α) staining was used to evaluate the hypoxia of the tumor tissues. Subsequently, the integral optical density (IOD) of CD31 (22), α-SMA (23), and HIF-1α were calculated for further evaluation (24).
Promoting the synergistic effect of RT
The breast cancer xenograft mice with MDA-MB-231 were randomly divided into 4 groups (n=5). The model (control) group was given a normal diet and sacrificed on the 14th day. The candesartan group was given 20 mg/kg of candesartan by gavage every 2 days and sacrificed on the 14th day. The RT group was given 5 Gay intensity RT on the 9th day and sacrificed on the 14th day. The window-period RT group was given 20 mg/kg of candesartan by gavage every 2 days, and then, given 5 Gay intensity RT on the 9th day, and sacrificed on the 14th day. Body weight and tumor size were measured before administration. After being sacrificed, the weight, tumor size, and tumor weight of the mice in each group were recorded. The tumor volumes and tumor growth inhibition rate (IR%) were calculated using Eqs. [1] and [2] as follows:
where a, b, Wt and Wc refer to the maximum diameter of the tumor, minimum diameter of the tumor, the mean tumor weight of treated groups and the mean tumor weight of the control group, respectively.
The tumor tissues were fixed with 10% neutral formaldehyde for 24 h, and then the tumor pieces of each group were taken and embedded in paraffin. The expression levels of Tunnel and Ki-67 in the tumor tissues were detected via immunohistochemistry to evaluate the effects on tumor proliferation and apoptosis.
Statistical analysis
All data is presented as the mean ± SD. Statistical significance was determined by one-way analysis of variance used SPSS software (IBM Inc., Chicago, IL, USA). P<0.05 was considered statistically significant, and extreme significance was set at P<0.01.
Results
Determination of the normalization window of the tumor vessels
Reducing tumor vessel density
As a marker of vascular endothelial cells, CD31 can be used to monitor the morphological and quantitative changes of blood vessels (22). In the model group, CD31 immunostaining (Figure 1) showed that the expression of CD31 continued to increase. In the candesartan group, the expression began to decrease on the 6th day after anti-tumor vascular treatment, and returned to its initial level on the 12th day (6th and 9th day, P<0.01). Thus, the tumor vessel density was temporarily decreased after candesartan administration. However, the inhibition of tumor vascular-related factors might lead to the strengthening expression of other signal pathways and a compensatory increase in tumor angiogenesis. In addition, the mechanism of action needs to be further studied.
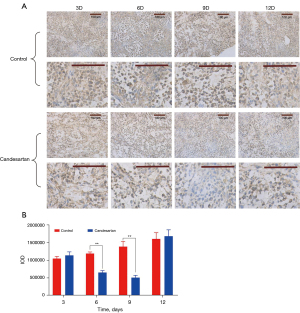
Increasing pericyte coverage
Pericytes, which are a sign of vascular stability and maturity, are one of the important manifestations of blood vascular normalization (23). The α-SMA immunostaining (Figure 2) showed that the expression of α-SMA in the model group continued to decrease. Conversely, the expression of α-SMA in the candesartan group rose again after a brief decline, which increased the wrapping of the pericytes around the blood vessels (9th and 12th day, P<0.01). Thus, the anti-vascular treatment increased the coverage of the perivascular cells and the tumor blood vessels were more mature than control group. The mechanism of pericyte change was not clear before and after the normalization window. Thrombospondin-1 (TSP-1) and angiopoietin 1 (Ang-1) are known to play important roles in the vascular recruitment of pericytes; however, this process involves complex factor regulation, which requires further study.
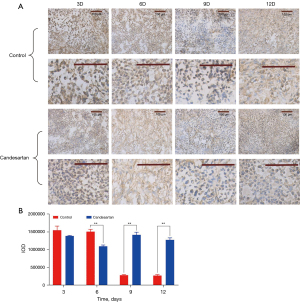
Increasing the blood perfusion of tumor tissue
The abnormality of tumor vessels is manifested in blood perfusion disorders. The high permeability of blood vessels increases the extravasation of plasma components, resulting in increased IFP, vascular compression, and decreased blood perfusion (24). An increase in blood perfusion is an important indicator of the recovery of vascular function. In this study, FITC-labeled lectin was injected intravenously. Next, the distribution of green fluorescence was observed under a fluorescence microscope to determine the amount of perfusion. The results illustrated that the intensity of green fluorescence distribution in the tumor tissues of the candesartan administration group was significantly higher than that of the control group on the 6th day (P<0.01; Figure 3), and this phenomenon continued until the 12th day (P<0.01). However, there was a downward trend over time between the 9th and 12th day in the candesartan group (P<0.01), proving that the excessive anti-vascular effect reduced blood vessel density, leading to a decrease in the blood perfusion of the tumor tissues. The results revealed that perfusion was significantly correlated with time, which was consistent with the change in permeability. It further established that the induction of tumor vascular normalization required the rational application of anti-vascular drugs rather than high-dose or long-term application, both which cause vascular damage.
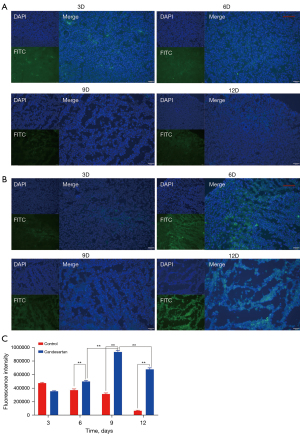
Decreasing the expression of HIF-1α
The rapid growth of tumors leads to hypoxia. As a central indicator of hypoxia, increased HIF-1α expression has become the initiating factor for the expression of a variety of pro-angiogenic factors, promoting angiogenesis and tumor progression (25). As Figure 4 shows, the expression of HIF-1α in the tumor tissues of the candesartan group showed a significant decline (6th, 9th, and 12th day, P<0.01). However, there was an upward trend between the 9th and 12th day in the candesartan group (P<0.01), indicating that hypoxia gradually recovered over time after the window period. The change trend was also in line with the permeability and perfusion trends. Thus, changes in vascular morphology and function improved the hypoxia of the tumors after a short period of anti-vascular treatment.
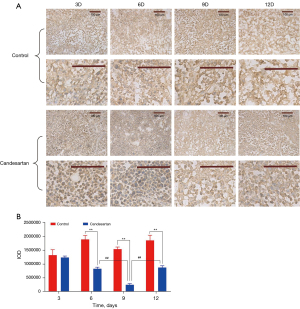
Promoting the synergistic effects of RT
The results of the time window study indicated that RT should commence on the 9th day. As Figure 5 shows, the tumor volume of the control group was significantly larger than that of the RT group (P<0.05) and the window-period RT group (P<0.01). Further, the anti-tumor effect was the best when RT was administered in the normalization window. As Table 1 shows, the tumor weight of the window-period RT group was lighter than that of the control group (P<0.01) and the RT group (P<0.05). According to the formula, the tumor IR% of the candesartan group, RT group and window-period RT group were 35.04%, 41.88%, and 66.67%, respectively. Thus, during the window period, candesartan enhanced the sensitivity of RT and produced the highest IR%.
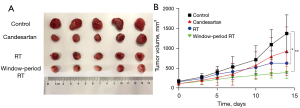
Table 1
Groups | Tumor weight | IR% |
---|---|---|
Control | 1.17±0.37 | – |
Candesartan | 0.76±0.44 | 35.04 |
RT | 0.68±0.20* | 41.88 |
Window-period RT | 0.39±0.09**# | 66.67 |
The results are expressed as the mean ± SD (n=5). *P<0.05, **P<0.01 vs. control group; #P<0.05 vs. RT group. RT, radiotherapy; IR, inhibition rate.
To further explore the therapeutic effects of RT on tumor cells in the window period, the Tunnel and Ki-67 staining methods were used to analyze the effects on tumor cell apoptosis and proliferation. The results (Figure 6) showed that window-period RT significantly promoted tumor cell apoptosis and limited proliferation compared to the control group (P<0.05 in Tunnel expression and P<0.01 in Ki-67 expression). The significant difference in value was small, but the overall efficacy of the window-period RT group tended to be better. This may be due to the insufficient amount of staining (n=3), which meant that the significance was not reflected. Thus, further research needs to be conducted on the effects on tumor cell apoptosis and proliferation.
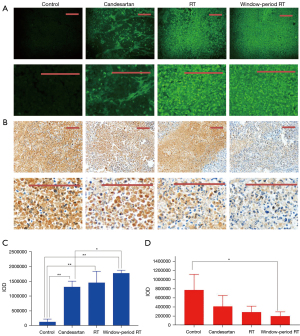
Discussion
Angiogenesis is pivotal in tumor metastasis. Hypoxia is a common regulatory target between tumor angiogenesis and metastasis (26). Tumor cells secrete a variety of pro-angiogenic factors to induce angiogenesis, extending in a “sprouting” manner to create their own escape route (27). Due to the weak vascular structure, incomplete basement membrane, large endothelial cell space, and incomplete pericyte coverage, tumor neovascularization is highly permeable, which provides a good channel for tumor cells to invade the circulatory system. Thus, moderate anti-tumor angiogenesis therapy could induce the normalization of tumor vessels and temporarily improve abnormal blood vessel structure and function. This makes the vessels more mature, enhances the integrity of the tube wall, improves blood perfusion, and increases the oxygen partial pressure of the tumor tissue. The improvement of the hypoxic microenvironment makes tumor cells more sensitive to RT (28).
In this study, we found that a series of changes took place in the structure and function of the tumor vessels after candesartan administration. First, it caused a decrease in vessel density; however, this was only a temporary decrease. Conversely, blood perfusion was increased. Second, pericyte coverage was more extensive. The pericytes wrapped the endothelial cells and coordinated signal transduction between cells to establish complete cell contact and participated in physiological and pathological vascular stability and permeability (23). In the process of tumor angiogenesis, the recruitment of pericytes is a key link in the maturation of tumor vessels, which is regulated by complex signal pathways. In the early stage of tumor angiogenesis, the dissociation of pericytes and endothelial cells is a critical event. A lack of pericytes, which is one of the causes of tumor vascular abnormalities, increases vascular permeability, IFP, and tissue edema (29). Accordingly, pericytes are the target of vascular normalization. The results showed that after a short-term anti-vascular treatment, the expression of α-SMA around the blood vessels was increased, indicating that the perivascular pericyte coverage was increased, and the blood vessels were more mature than before.
Hypoxia is a predictive factor for angiogenesis, invasion, metastasis, and the effects of RT or chemotherapy, and plays a central role in tumor progression (30). Hypoxia is the result of tumor development to a certain extent, and promotes tumor progression and metastasis through a variety of direct and indirect mechanisms. Additionally, tumor cells are resistant to RT and chemotherapy in a hypoxic environment. Thus, hypoxia is an important obstacle in tumor treatment. In a tumor site, oxygen within 70–150 µm from blood vessels is consumed by rapidly proliferating tumor cells, limiting its spread to greater distances. Thus, hypoxia usually occurs at a distance of 100–200 µm from blood vessels, exposing different proportions of tumor cells (<1% to >50%) to a low-oxygen environment (pO2 <10 mmHg) (31). HIF-1α is the main transcriptional regulator of tumor cell response to hypoxia, which can promote tumor invasion, interfere with glucose metabolism, maintain the characteristics of tumor stem cells, and induce multi-drug resistance. HIF-1α, which is the central link in stimulating the growth of tumor vessels, also promotes the expression of VEGF, AngII, fibroblast growth factor 2 (FGF2), and other factors (25).
We found that the expression of HIF-1α in tumor tissues was significantly reduced on the 6th and 9th days after treatment (P<0.05). This may be due to tumor vascular normalization induced by candesartan, which resulted in secondary changes of increased vascular perfusion and oxygenation. However, HIF-1α began to increase significantly on the 12th day. As the time window for tumor vascular normalization receded, further anti-vascularization led to a decrease in oxygen content. According to the results of the anti-tumor studies, the tumor IR% given by RT during the window period was the highest. Additionally, the immunohistochemical results showed that tumor cell apoptosis was the highest and the proliferation ability was significantly inhibited. Thus, alleviating hypoxia is beneficial to improving sensitivity to RT, increasing the effect of RT, inhibiting tumor growth, and improving the survival rate.
Notably, there is a significant correlation between the normalization of blood vessels and the dosage and the treatment time of anti-vascular drugs (32). Any increase in anti-vascular therapy would reduce the time window for tumor vascular normalization, thereby achieving tumor vascular inhibition. This aggravates hypoxia and induces the formation of cancer stem cells. For example, high-dose anti-VEGF/VEGFR (VEGF receptor) could block excessive blood vessels, reducing tumor vascular perfusion rapidly, and hindering the therapeutic effect. Conversely, lower doses may improve perfusion and treatment results. Indeed, low-dose anti-VEGFR2 antibodies (10 or 20 mg/kg) were shown to increase blood vessel perfusion in breast cancer models compared to high-dose (40 mg/kg) anti-VEGFR2 antibodies or control immunoglobulin G (18,33).
As a typical drug of ARBs, candesartan has been extensively studied in relation to the anti-angiogenesis of various tumors (18,34-37). Although candesartan is effective in inducing tumor vascular normalization in preclinical studies, it still faces many challenges in clinical application. In addition, only a minority of patients treated with candesartan achieved significantly enhanced therapeutic effects during radiotherapy, mainly due to radiotherapy given outside the therapeutic window of tumor vascular normalization (19,34). However, the therapeutic window for vascular normalization must be precisely detected before clinical application. Based on these studies (18,34-37) and our previous work (19), this study further explored and determined the normalization window period of tumor blood vessels after candesartan administration in tumor-bearing mice. We found that administering RT improved the curative effect during the therapeutic window. The normalization time of tumor vessels is short and there are individual differences. Thus, the question of how to find an effective monitoring method is particularly important. This study provided ideas and methods for monitoring the normalization-time window of tumor vessels. Unfortunately, due to the problem of traumatic sampling, it may not be effectively promoted in clinical practice. Recently, magnetic resonance imaging technology was applied to monitor the morphology and function of tumor vessels, and it is expected that this will be further promoted in clinical practice (38). Once the time window is accurately identified in clinical settings, timely combined RT and chemotherapy technology could significantly improve the curative effect and reduce the organ toxic side effects caused by the overdose of cytotoxic drugs. Further clarification of the sensitivity of anti-vascular drugs would help patients obtain more precise treatment. Further, loading nanocarriers with candesartan could improve the tumor targeting effect, which in turn could improve the therapeutic effect and reduce the toxic and side effects (39-41). However, changes in the therapeutic effect need to be further explored to determine the therapeutic window. It is worth mentioning that, according to our previous study, the application of candesartan during tumor treatment did not increase the incidence of adverse reactions, including systemic (vomiting, dizziness) and local (erythema, blisters, and itching) adverse effects of radiation therapy (19,42).
Conclusions
To improve the RT effect, an ARB (i.e., candesartan) was used to induce tumor vascular normalization, which temporarily matured the vascular structure, increased blood perfusion, and relieved the hypoxic state. On the 9th day of RT, high-energy ionizing radiation generated ROS in the aerobic microenvironment, damaging the DNA structure of the tumor cells and causing tumor cell death. In summary, candesartan exerted a synergistic effect on RT by inducing tumor vascular normalization in the therapeutic window.
Acknowledgments
Funding: This work was supported by the Natural Science Foundation of China (No. 82003669), the Zhoushan Medical and Health Science and Technology Plan (Nos. 2020YA06 and 2021YB06), and the Zhejiang Medical and Health Science and Technology Plan (No. 2020KY477).
Footnote
Reporting Checklist: The authors have completed the ARRIVE reporting checklist. Available at https://atm.amegroups.com/article/view/10.21037/atm-22-2108/rc
Data Sharing Statement: Available at https://atm.amegroups.com/article/view/10.21037/atm-22-2108/dss
Conflicts of Interest: All authors have completed the ICMJE uniform disclosure form (available at https://atm.amegroups.com/article/view/10.21037/atm-22-2108/coif). Fei Zhu reports that the study was supported by the Zhoushan Medical and Health Science and Technology Plan (Nos. 2020YA06 and 2021YB06). The other authors have no conflicts of interest to declare.
Ethical Statement: The authors are accountable for all aspects of the work in ensuring that questions related to the accuracy or integrity of any part of the work are appropriately investigated and resolved. Animal experiments were performed under a project license (No. ZJU20210092) granted by laboratory animal welfare and ethics committee of Zhejiang University. All the experiments were performed in accordance with the guidelines for the care and use of animals established by Zhejiang University.
Open Access Statement: This is an Open Access article distributed in accordance with the Creative Commons Attribution-NonCommercial-NoDerivs 4.0 International License (CC BY-NC-ND 4.0), which permits the non-commercial replication and distribution of the article with the strict proviso that no changes or edits are made and the original work is properly cited (including links to both the formal publication through the relevant DOI and the license). See: https://creativecommons.org/licenses/by-nc-nd/4.0/.
References
- Da F, Guo J, Yao L, et al. Pretreatment with metformin protects mice from whole-body irradiation. J Radiat Res 2021;62:618-25. [Crossref] [PubMed]
- Jairam V, Lee V, Park HS, et al. Treatment-Related Complications of Systemic Therapy and Radiotherapy. JAMA Oncol 2019;5:1028-35. [Crossref] [PubMed]
- Chen M, Singh AK, Repasky EA. Highlighting the Potential for Chronic Stress to Minimize Therapeutic Responses to Radiotherapy through Increased Immunosuppression and Radiation Resistance. Cancers (Basel) 2020;12:3853. [Crossref] [PubMed]
- Vostrikova SM, Grinev AB, Gogvadze VG. Reactive Oxygen Species and Antioxidants in Carcinogenesis and Tumor Therapy. Biochemistry (Mosc) 2020;85:1254-66. [Crossref] [PubMed]
- Qin X, Yang C, Xu H, et al. Cell-Derived Biogenetic Gold Nanoparticles for Sensitizing Radiotherapy and Boosting Immune Response against Cancer. Small 2021;17:e2103984. [Crossref] [PubMed]
- Huang Y, Fan J, Li Y, et al. Imaging of Tumor Hypoxia With Radionuclide-Labeled Tracers for PET. Front Oncol 2021;11:731503. [Crossref] [PubMed]
- Wei X, Chen Y, Jiang X, et al. Mechanisms of vasculogenic mimicry in hypoxic tumor microenvironments. Mol Cancer 2021;20:7. [Crossref] [PubMed]
- Sasajima J, Mizukami Y, Sugiyama Y, et al. Transplanting normal vascular proangiogenic cells to tumor-bearing mice triggers vascular remodeling and reduces hypoxia in tumors. Cancer Res 2010;70:6283-92. [Crossref] [PubMed]
- Sandha KK, Shukla MK, Gupta PN. Recent Advances in Strategies for Extracellular Matrix Degradation and Synthesis Inhibition for Improved Therapy of Solid Tumors. Curr Pharm Des 2020;26:5456-67. [Crossref] [PubMed]
- Li B, Chu T, Wei J, et al. Platelet-Membrane-Coated Nanoparticles Enable Vascular Disrupting Agent Combining Anti-Angiogenic Drug for Improved Tumor Vessel Impairment. Nano Lett 2021;21:2588-95. [Crossref] [PubMed]
- Shang B, Cao Z, Zhou Q. Progress in tumor vascular normalization for anticancer therapy: challenges and perspectives. Front Med 2012;6:67-78. [Crossref] [PubMed]
- Guo F, Cui J. Anti-angiogenesis: Opening a new window for immunotherapy. Life Sci 2020;258:118163. [Crossref] [PubMed]
- Matuszewska K, Pereira M, Petrik D, et al. Normalizing Tumor Vasculature to Reduce Hypoxia, Enhance Perfusion, and Optimize Therapy Uptake. Cancers (Basel) 2021;13:4444. [Crossref] [PubMed]
- Shrestha S, Noh JM, Kim SY, et al. Angiotensin converting enzyme inhibitors and angiotensin II receptor antagonist attenuate tumor growth via polarization of neutrophils toward an antitumor phenotype. Oncoimmunology 2015;5:e1067744. [Crossref] [PubMed]
- Afsar B, Afsar RE, Ertuglu LA, et al. Renin-angiotensin system and cancer: epidemiology, cell signaling, genetics and epigenetics. Clin Transl Oncol 2021;23:682-96. [Crossref] [PubMed]
- Chen X, Meng Q, Zhao Y, et al. Angiotensin II type 1 receptor antagonists inhibit cell proliferation and angiogenesis in breast cancer. Cancer Lett 2013;328:318-24. [Crossref] [PubMed]
- Wadsworth BJ, Cederberg RA, Lee CM, et al. Angiotensin II type 1 receptor blocker telmisartan inhibits the development of transient hypoxia and improves tumour response to radiation. Cancer Lett 2020;493:31-40. [Crossref] [PubMed]
- Alhusban A, Al-Azayzih A, Goc A, et al. Clinically relevant doses of candesartan inhibit growth of prostate tumor xenografts in vivo through modulation of tumor angiogenesis. J Pharmacol Exp Ther 2014;350:635-45. [Crossref] [PubMed]
- Qi Yajun, Ding Haiying, Yang Guonong, et al. Effects of ACEI/ARB Agent on the Prognosis of Patients with Advanced Gastric Cancer Complicated with Hypertension. China Pharmacy 2017;28:4517-4519.
- Alashkham A, Paterson C, Windsor P, et al. The Incidence and Risk of Biochemical Recurrence Following Radical Radiotherapy for Prostate Cancer in Men on Angiotensin-Converting Enzyme Inhibitors (ACEIs) or Angiotensin Receptor Blockers (ARBs). Clin Genitourin Cancer 2016;14:398-405. [Crossref] [PubMed]
- Yang T, Xiao H, Liu X, et al. Vascular Normalization: A New Window Opened for Cancer Therapies. Front Oncol 2021;11:719836. [Crossref] [PubMed]
- Lertkiatmongkol P, Liao D, Mei H, et al. Endothelial functions of platelet/endothelial cell adhesion molecule-1 (CD31). Curr Opin Hematol 2016;23:253-9. [Crossref] [PubMed]
- Mukwaya A, Mirabelli P, Lennikov A, et al. Revascularization after angiogenesis inhibition favors new sprouting over abandoned vessel reuse. Angiogenesis 2019;22:553-67. [Crossref] [PubMed]
- Jain RK, Martin JD, Stylianopoulos T. The role of mechanical forces in tumor growth and therapy. Annu Rev Biomed Eng 2014;16:321-46. [Crossref] [PubMed]
- Tirpe AA, Gulei D, Ciortea SM, et al. Hypoxia: Overview on Hypoxia-Mediated Mechanisms with a Focus on the Role of HIF Genes. Int J Mol Sci 2019;20:6140. [Crossref] [PubMed]
- Rankin EB, Nam JM, Giaccia AJ. Hypoxia: Signaling the Metastatic Cascade. Trends Cancer 2016;2:295-304. [Crossref] [PubMed]
- Schor AM, Woolston AM, Kankova K, et al. Migration Stimulating Factor (MSF): Its Role in the Tumour Microenvironment. Adv Exp Med Biol 2021;1329:351-97. [Crossref] [PubMed]
- Zheng R, Li F, Li F, et al. Targeting tumor vascularization: promising strategies for vascular normalization. J Cancer Res Clin Oncol 2021;147:2489-505. [Crossref] [PubMed]
- Krishna Priya S, Nagare RP, Sneha VS, et al. Tumour angiogenesis-Origin of blood vessels. Int J Cancer 2016;139:729-35. [Crossref] [PubMed]
- Liu Z, Zhao Q, Zheng Z, et al. Vascular normalization in immunotherapy: A promising mechanisms combined with radiotherapy. Biomed Pharmacother 2021;139:111607. [Crossref] [PubMed]
- Kulkarni P, Haldar MK, Katti P, et al. Hypoxia Responsive, Tumor Penetrating Lipid Nanoparticles for Delivery of Chemotherapeutics to Pancreatic Cancer Cell Spheroids. Bioconjug Chem 2016;27:1830-8. [Crossref] [PubMed]
- Chen X, Jin R, Jiang Q, et al. Delivery of siHIF-1α to Reconstruct Tumor Normoxic Microenvironment for Effective Chemotherapeutic and Photodynamic Anticancer Treatments. Small 2021;17:e2100609. [Crossref] [PubMed]
- Cai XJ, Wang Z, Xu YY, et al. Candesartan treatment enhances liposome penetration and anti-tumor effect via depletion of tumor stroma and normalization of tumor vessel. Drug Deliv Transl Res 2021;11:1186-97. [Crossref] [PubMed]
- Herr D, Sauer C, Holzheu I, et al. Role of Renin-Angiotensin-System in Human Breast Cancer Cells: Is There a Difference in Regulation of Angiogenesis between Hormone-Receptor Positive and Negative Breast Cancer Cells? Geburtshilfe Frauenheilkd 2019;79:626-34. [Crossref] [PubMed]
- Fan F, Tian C, Tao L, et al. Candesartan attenuates angiogenesis in hepatocellular carcinoma via downregulating AT1R/VEGF pathway. Biomed Pharmacother 2016;83:704-11. [Crossref] [PubMed]
- Nakamura S, Tsuruma K, Shimazawa M, et al. Candesartan, an angiotensin II type 1 receptor antagonist, inhibits pathological retinal neovascularization by downregulating VEGF receptor-2 expression. Eur J Pharmacol 2012;685:8-14. [Crossref] [PubMed]
- Kosugi M, Miyajima A, Kikuchi E, et al. Angiotensin II type 1 receptor antagonist candesartan as an angiogenic inhibitor in a xenograft model of bladder cancer. Clin Cancer Res 2006;12:2888-93. [Crossref] [PubMed]
- Jarzyna PA, Deddens LH, Kann BH, et al. Tumor angiogenesis phenotyping by nanoparticle-facilitated magnetic resonance and near-infrared fluorescence molecular imaging. Neoplasia 2012;14:964-73. [Crossref] [PubMed]
- Zhao L, Zhao W, Liu Y, et al. Nano-Hydroxyapatite-Derived Drug and Gene Co-Delivery System for Anti-Angiogenesis Therapy of Breast Cancer. Med Sci Monit 2017;23:4723-32. [Crossref] [PubMed]
- Bao X, Wang W, Wang C, et al. A chitosan-graft-PEI-candesartan conjugate for targeted co-delivery of drug and gene in anti-angiogenesis cancer therapy. Biomaterials 2014;35:8450-66. [Crossref] [PubMed]
- Ding X, Su Y, Wang C, et al. Synergistic Suppression of Tumor Angiogenesis by the Co-delivering of Vascular Endothelial Growth Factor Targeted siRNA and Candesartan Mediated by Functionalized Carbon Nanovectors. ACS Appl Mater Interfaces 2017;9:23353-69. [Crossref] [PubMed]
- Ashack KA, Kuritza V, Visconti MJ, et al. Dermatologic Sequelae Associated with Radiation Therapy. Am J Clin Dermatol 2020;21:541-55. [Crossref] [PubMed]
(English Language Editor: L. Huleatt)