Seawater immersion exacerbates the pathological changes caused by incisive corneal injury in rabbit eyes
Introduction
Eye injury is often observed on the battlefield as modern weapons are designed to inflict maximal body injury on enemy personnel (1,2). Seawater immersion aggravates the injuries incurred at sea and causes specific pathological changes (3,4). After explosive corneal penetrating injuries, seawater has been shown to cause increased pigmentation, inflammation, corneal thickening and vascularization, as well as delayed healing in rabbit eyes (5).
Aquaporins (AQPs) are a family of transmembrane water channels involved in various physiological functions (6,7). By following the local osmotic gradient, AQPs facilitate fluids crossing the cell membranes into and out tissues, thereby regulating the cell volume. In addition, AQPs also play a role in cell proliferation, migration, cancer metastasis (8-10), and angiogenesis (11,12).
All thirteen AQPs identified in humans are expressed in ocular tissues (13). The mRNAs of AQP0, 1, 2, 3, 4, 5, 6, 11, and 12, as well as the proteins of AQP1, 3, 5, and 11, have been detected in the rat cornea (14), whereas the mRNA and protein expression of AQP1, 3, and 5 have been detected in the canine cornea (15). AQP is involved in corneal injury repair and regulation of stromal thickness. This study investigated the changes in AQP expression in the rabbit cornea following seawater immersion after explosive penetrating injury, and its implication in pathological progression and injury healing was examined. We present the following article in accordance with the ARRIVE reporting checklist (available at https://atm.amegroups.com/article/view/10.21037/atm-22-2198/rc).
Methods
Animal treatment
New Zealand White rabbits of either gender, weighing 2.0–2.5 kg, were provided by the Jiangnan Experimental Animals of Huishan, Wuxi, China. Animal experiments were performed under a project license (No. ZZDXOHP20191011) granted by ethics board of Zhengzhou University, in compliance with China national guidelines for the care and use of animals. The experiment used seawater from the east China sea. Animals were divided into the following 3 groups, with 6 rabbits per group: (I) the model group; (II) the seawater immersion group; and (III) the seawater immersion combined with dexamethasone (DXM) treatment group. Before experimentation, rabbits were anesthetized with 1% pentobarbital sodium via intravenous injection to the ear. Rabbits were fixed onto a frame. The eyelid was opened through the use of an eyelid retractor. The rabbit eyes were injured using a firecracker, with the explosion center 5 mm away from corneal vertex. After the firecracker explosion, a 3-mm long whole-layer incision parallel to the corneal limbus was made in the center of the cornea. In the seawater immersion group, 0.2 mL of seawater was injected into the anterior chamber via corneal incision. After the anterior chamber was cleared with slight pressing the incision, seawater was injected again, and this procedure was repeated three times. The model group underwent the procedure as for the experimental group, with the exception that seawater was replaced by physiological saline. In the seawater immersion group, the eye surface was flushed for 30 minutes with seawater, and in the model group, physiological saline was used during this procedure. For the treatment group, the rabbits were given neomycin/polymyxin B/DXM eye drops every 3 hours, 5 times. The aqueous humor was collected and the cornea was harvested on days 1 and 7 post-injury, respectively.
Histopathological evaluation
After euthanasia, the cornea was fixed in 10% formaldehyde and embedded in paraffin. Histological sections of 5 µm were obtained and stained with hematoxylin and eosin (HE).
Determination of cytokine concentrations in the aqueous humor
The levels of tumor necrosis factor α (TNF-α), interleukin (IL)-1β, IL-6, monocyte chemoattractant protein 1 (MCP-1), IL-8, transforming growth factor β (TGF-β), and vascular endothelial growth factor A (VEGFA) in the aqueous humor were measured using commercial enzyme-linked immunosorbent assay (ELISA) kits (Elabscience Company, Wuhan, China) according to the manufacturer’s instructions.
Immunocytochemistry
Histological slices were dewaxed, rehydrated, and treated with 3% hydrogen peroxide for 10 minutes to inactivate the endogenous peroxidase. Antigen repaired was performed using 40× antigen repair liquid, followed by blocking in 10% normal goat serum for 1 hour at room temperature. The slices were then incubated with primary antibodies against AQP1 (1:500, 1/A5F6, Bio-Rad, Shanghai, China), CD68 (1:200, ab955, Abcam, Cambridge, MA, USA), AQP3 (1:200, ab153694, Abcam), or AQP5 (1:100, SCBT, Dallas, TX, USA) at 4 ℃ overnight, followed by goat anti-mouse, goat anti-rabbit, or monkey anti-goat CY3 secondary antibodies (SA00009, Proteintech, Wuhan, China) away from light for 1 hour at room temperature. Slides were then counterstained with Hoechst and mounted.
Quantitative real-time polymerase chain reaction (qRT-PCR)
Total RNA was extracted from the cornea using RNeasy mini kits (Qiagen, Venlo, Netherlands). Reverse transcription was performed with the SuperScript® III First-Strand Synthesis System (Life Tech, Shanghai, China) according to the manufacturer’s instructions using 1 g total RNA. qRT-PCR was performed using the SYBR® Green PCR Master Mix (Life Tech) on an ABI 7300 system (Applied Biosystems, Foster City, CA, USA) with the following program: 95 ℃ for 3 minutes followed by 40 cycles of 95 ℃ for 15 seconds, 55 ℃ for 15 seconds, and 68 ℃ for 30 seconds. The relative transcription levels were calculated using the 2−ΔΔCt method with β-actin as the internal control. The primer sequences were listed in Table 1.
Table 1
Gene | Primer | Sequence (5'-3') |
---|---|---|
IL-6 | Forward | GCGGCCTCACAAACTTCCTG |
Reverse | GGTGTGTTCTGACCGTGGGA | |
TNF-α | Forward | ATCGGCCCTCAGGAGGAAGA |
Reverse | CGTGGGCTAGAGGCTTGTCA | |
MCP-1 | Forward | GCTCTGCTTGCTGCCATTCT |
Reverse | ACGTAGATGGCCACGTCCTG | |
IL-1β | Forward | CTGCTCGCAGACACCCTCAT |
Reverse | CGTGCCAGACAACACCAAGG | |
IL-8 | Forward | CTGCTCGCAGACACCCTCAT |
Reverse | TTGAGGCAGCTGTGCAGGAA | |
TGF-β | Forward | GCTCCTTGGACGGATGCAGA |
Reverse | GGCCACGACGGGAACTTCTA | |
VEGFA | Forward | AGAGTGGTGGGAGGGCAGAT |
Reverse | GCCTCGGCTTGTCACATCTC | |
AQP1 | Forward | CTGGGACACCTGTTGGCGAT |
Reverse | CCACCCAGAAGATCCAGTGGT | |
AQP3 | Forward | TGAACCCTGCCGTGACCTTT |
Reverse | CAAAGATGCCTGCTGTGCCA | |
AQP5 | Forward | TGGTGCTGGCATCCTCTACG |
Reverse | GCGTGAGTCGGTGGAAGAGA | |
CD68 | Forward | CCTACCACTGGCCACGGAAA |
Reverse | GCCTGGGCTAGGACTTGGAG | |
β-actin | Forward | AGGGCGTCATGGTGGGTATG |
Reverse | GCCGTGCTCGATGGGATACT |
IL, interleukin; TNF-α, tumor necrosis factor α; MCP-1, monocyte chemoattractant protein 1; TGF-β. transforming growth factor β; VEGFA, vascular endothelial growth factor A; AQP, aquaporin.
Western blot
The nucleoproteins were extracted using the Nucleoprotein Extracted Kit (Bestbio, Shanghai, China), according to the manufacturer’s instructions. Total protein was extracted using the following conventional method. Tissues were lysed in RIPA buffer (50 mM Tris-Cl pH7.4, 150 mM NaCl, 1% NP40, 0.25% Na-deoxycholate) containing 1× complete protease inhibitor cocktail (Roche Diagnostics, Indianapolis, IN, USA). The samples were vigorously vortexed for 15 seconds and centrifuged at 10,000 g for 15 minutes at 4 ℃. Total protein (40 µg) was resolved on 8% sodium dodecyl sulfate polyacrylamide gels and transferred onto polyvinylidene fluoride (PVDF) membranes. The membranes were blocked for 30 minutes with 5% nonfat milk in phosphate buffered saline Tween (PBST; 0.1% Tween 20 in PBS) at room temperature, incubated with specific primary antibodies at 4 ℃ overnight, washed 3 times with PBST, and incubated with the appropriate secondary horseradish peroxidase-conjugated antibody (Pierce Antibody, Thermo Scientific, Shanghai, China) for 60 minutes at room temperature. The primary antibodies used were p65 (bs-0465R; Bioss, China), lamin B (ab8982; Abcam), and β-actin (ab8227; Abcam). The specific protein bands were detected with Immun-StarTM Western Chemiluminescence kit (Bio-Rad).
Statistical analysis
The data was expressed as mean ± standard deviation. The differences between groups were analyzed by one way analysis of variance using GraphPad Prism 5 and Origin 6.1. A P value less than 0.05 was considered statistically significant.
Results
Seawater immersion aggravates penetrating explosion injuries to the cornea
Penetrating explosion injury and injury followed by seawater immersion caused progressive damage to corneal tissues. One day after injury, the epithelium was significantly damaged and seawater immersion worsened the damage, which was alleviated by neomycin/polymyxin B/DXM (NPD) eye drop treatment (Figure 1A). At 7 days post-injury, prominent apoptosis of the corneal epithelial cells could be observed, as well as vacuolar degeneration and disarrangement of endothelial cells. Seawater immersion after injury caused corneal atrophy, increased apoptosis of epithelial cells, more vacuoles, and disintegration of the endothelium (Figure 1A). Moreover, the amount of CD68-positive inflammatory cells was markedly elevated in the stroma of injured corneas, and further increased in corneas that suffered both injury and seawater immersion (Figure 1B).
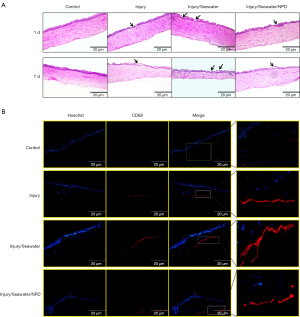
Seawater immersion exacerbates the inflammation caused by penetrating explosion injury to the cornea
To determine the effects of seawater immersion on corneal injury caused by penetrating explosions, the levels of inflammatory cytokines/chemokines in the aqueous humor were examined at 1 day and 7 days after injury. The levels of IL-6, IL-1β, IL-8, TNF-α, and MCP-1 in the aqueous humor were significantly elevated after penetrating explosion injury, and further exacerbated by seawater immersion at 1 day (Figure 2A) and 7 days (Figure 2B) after injury.
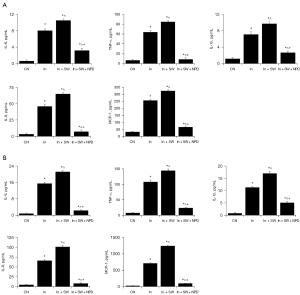
Seawater immersion enhances injury-induced activation of NF-κB
As explosion injury and seawater immersion caused significant inflammation in rabbit eyes, the expression of the inflammation regulator NF-κB was evaluated by Western blot analysis. Penetrating explosion injury caused drastic increases in the expression of total and nuclear-localized NF-κB p65 protein and these increases were heightened by seawater immersion after injury (Figure 3).
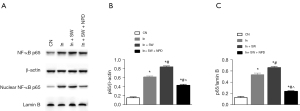
Seawater immersion enhances the changes in corneal AQP expression caused by explosion injury
Since the eye is a water-transporting organ (16), the changes in the expression of water channel AQPs in the corneas were examined after explosion injury and seawater immersion. The mRNA (Figure 4) and protein levels of AQP1 (Figure 5) and AQP5 (Figure 6) were significantly elevated following penetrating explosion injury, while the expression of AQP3 (Figure 7) was markedly reduced in corneas after explosion injury compared to uninjured control corneas. Post-injury seawater immersion further enhanced the changes in the expression of the AQPs in the rabbit corneas (Figures 5-7).
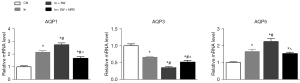
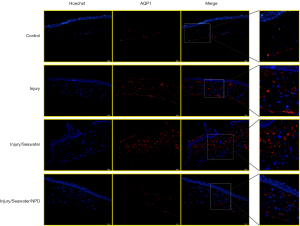
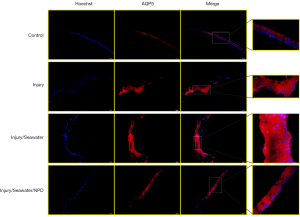
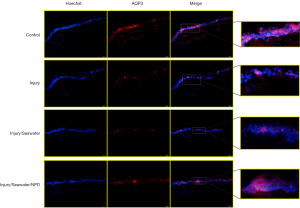
Discussion
To mimic injuries suffered during maritime conflicts, a rabbit model was established where penetrating explosion corneal injury was followed by seawater immersion. The molecular, histological, and physiological changes after such insults were evaluated. Compared to injury-only corneas, injury followed by seawater immersion caused more severe and profound damage to the cornea, greater inflammation, and more significant changes in the expression of AQPs.
Ocular inflammation is one of the most prominent events during corneal wound recovery (16). A blast injury causes a series of pathological changes in the ocular system, including corneal edema, inflammation, glial reactivation, neovascularization, and apoptosis (17,18). Exposure to sulfur mustard has been shown to cause corneal epithelium loss, corneal edema, limbal engorgement, and ocular inflammation in mice, as well as significant increases in the expression of IL-1α, IL-1β, IL-6, MCP-1, and soluble vascular cell adhesion molecule-1 (sVCAM-1) in the eyes (19). Nicotinamide adenine dinucleotide phosphate (NADPH) oxidases 2 and 4 have been shown to be upregulated in alkali-burned human and mouse corneas, and induced oxidative stress and inflammation (20). Telomeric repeat binding factor 2, interacting protein (TERF2IP) knockout mice had substantially lower IL-1α levels compared to wild type mice after alkali burn to the cornea (21). Deletion of transient receptor potential ankyrin 1 (TRPA1) also suppressed corneal inflammation and fibrosis after alkali burn (22). Taken together, these results demonstrated that inflammation is a natural pathological process after corneal injuries, and this was confirmed in our current study showing that proinflammatory cytokines and chemokines were upregulated after corneal injury.
AQPs have been shown to participate in many physiopathological processes. Pulmonary AQP5 levels were reduced after acute kidney injury caused by indoxyl sulfate, which was alleviated by oral administration of clinically-used oral spherical adsorptive carbon beads AST-120 (23). X-ray irradiation on the rat thorax caused lung inflammation and fibrosis, decreased expression of AQP1, as well as a short-term increase (7 days after irradiation) followed by a decrease in AQP5 levels in the lungs (24). AQP3 has been shown to be significantly upregulated in the epidermis around the burn wound edge (25,26), but was unchanged after a postmortem burn or mechanical wound of skin (26). Moreover, severe burning can cause myocardial edema and upregulation of AQP1 and other AQPs, including AQP3 (27). Seawater drowning results in acute lung injury and overexpression of AQP1 and AQP5 in lung tissues (28). Together with these studies, the data presented herein demonstrated the importance of AQPs in maintaining water homeostasis and the pathological processes of different tissues including the eyes. AQP1 is expressed in corneal endothelium and critical for transport water out of the corneal stroma while AQP3 and AQP5 are expressed in stratified epithelium of the anterior corneal epithelium to transport water away from the cornea (29). Moreover, knockout mouse AQP5 inhibited corneal wound healing (30), deletion of AQP3 substantially delayed mouse corneal re-epithelialization after injury (31), and AQP1 levels were decreased in cornea of human corneal endothelial disease or mouse corneal endothelial injury (32).
AQPs are intimately involved in inflammation in different tissues caused by various stimuli (33-40). AQP5 is required for house dust mite-induced airway inflammation, as increased expression of inflammatory cytokines (including IL-2, IL-4, IL-10, and interferon-γ), mucin hypersecretion, and T helper 2 (Th2) inflammatory cells were prominent in wild type C57BL/6 mice but suppressed in AQP5 knockout mice (33).
Similarly, AQP3 knockout mice had significantly less airway inflammation than wild type mice upon ovalbumin-induced asthma and AQP3 stimulated the production of inflammatory chemokines including CCL22 and CCL24 by alveolar macrophages and T cell trafficking (34). AQP1 expression was upregulated in leukocytes of sepsis patients (37) and in fibrochondrocytes of anterior cruciate ligament transaction induced osteoarthritic rat meniscus (38). In cultured polymorphonuclear granulocytes, AQP1 expression was induced by lipopolysaccharide (LPS) in a NF-κB dependent manner and accompanied by cellular edema and IL-8 production (37). AQP1 deficiency or blockade impeded macrophage swelling, NLRP3 inflammasome activation, and IL-1β mature and secretion (35). Similarly, the current study showed that seawater immersion of injured eyes resulted in changes of AQPs accompanied by macrophage infiltration and increases of cytokines/chemokines.
In summary, seawater immersion aggravated corneal injury, inflammatory response, cytokine/chemokine secretion, and AQP expression, which demonstrated the urgent need for fast care for wound individuals during maritime events.
Acknowledgments
Funding: This study was supported by Young Talents in Military Medical Science and Technology (grant number: #14QNP062).
Footnote
Reporting Checklist: The authors have completed the ARRIVE reporting checklist. Available at https://atm.amegroups.com/article/view/10.21037/atm-22-2198/rc
Data Sharing Statement: Available at https://atm.amegroups.com/article/view/10.21037/atm-22-2198/dss
Conflicts of Interest: All authors have completed the ICMJE uniform disclosure form (available at https://atm.amegroups.com/article/view/10.21037/atm-22-2198/coif). The authors have no conflicts of interest to declare.
Ethical Statement: The authors are accountable for all aspects of the work in ensuring that questions related to the accuracy or integrity of any part of the work are appropriately investigated and resolved. Animal experiments were performed under a project license (No. ZZDXOHP20191011) granted by ethics board of Zhengzhou University, in compliance with China national guidelines for the care and use of animals.
Open Access Statement: This is an Open Access article distributed in accordance with the Creative Commons Attribution-NonCommercial-NoDerivs 4.0 International License (CC BY-NC-ND 4.0), which permits the non-commercial replication and distribution of the article with the strict proviso that no changes or edits are made and the original work is properly cited (including links to both the formal publication through the relevant DOI and the license). See: https://creativecommons.org/licenses/by-nc-nd/4.0/.
References
- Hilber DJ. Eye injuries, active component, U.S. Armed Forces, 2000-2010. MSMR 2011;18:2-7. [PubMed]
- Gay DA, Ritchie JV, Perry JN, et al. Ultrasound of penetrating ocular injury in a combat environment. Clin Radiol 2013;68:82-4. [Crossref] [PubMed]
- Chen S, Huang Z, Wang L, et al. Effect of seawater soaking on explosive cornea injury. Yan Ke Xue Bao 2002;18:59-62. [PubMed]
- Fan Q, Chen Q, Hong J, et al. Corneal histopathological changes and interleukin-6 level in aqueous humor of a rabbit model of penetrating corneal trauma combined with seawater immersion. Chinese Journal of Tissue Engineering Research 2011;15:9381-4.
- Chen S, Shen W, Shi Y, et al. Changes in corneal morphology after seawater soaking following explosive injury in rabbit. Journal of Medical Postgraduates 2004;17:220-1.
- Papadopoulos MC, Saadoun S. Key roles of aquaporins in tumor biology. Biochim Biophys Acta 2015;1848:2576-83. [Crossref] [PubMed]
- Finn RN, Cerdà J. Evolution and functional diversity of aquaporins. Biol Bull 2015;229:6-23. [Crossref] [PubMed]
- Hara-Chikuma M, Verkman AS. Aquaporin-3 facilitates epidermal cell migration and proliferation during wound healing. J Mol Med (Berl) 2008;86:221-31. [Crossref] [PubMed]
- Zhang Z, Chen Z, Song Y, et al. Expression of aquaporin 5 increases proliferation and metastasis potential of lung cancer. J Pathol 2010;221:210-20. [Crossref] [PubMed]
- Esteva-Font C, Jin BJ, Verkman AS. Aquaporin-1 gene deletion reduces breast tumor growth and lung metastasis in tumor-producing MMTV-PyVT mice. FASEB J 2014;28:1446-53. [Crossref] [PubMed]
- Jiang Y, Liu H, Liu WJ, et al. Endothelial Aquaporin-1 (AQP1) Expression Is Regulated by Transcription Factor Mef2c. Mol Cells 2016;39:292-8. [Crossref] [PubMed]
- Zou LB, Shi S, Zhang RJ, et al. Aquaporin-1 plays a crucial role in estrogen-induced tubulogenesis of vascular endothelial cells. J Clin Endocrinol Metab 2013;98:E672-82. [Crossref] [PubMed]
- Schey KL, Wang Z, L, Wenke J, et al. Aquaporins in the eye: expression, function, and roles in ocular disease. Biochim Biophys Acta 2014;1840:1513-23. [Crossref] [PubMed]
- Yu D, Thelin WR, Randell SH, et al. Expression profiles of aquaporins in rat conjunctiva, cornea, lacrimal gland and Meibomian gland. Exp Eye Res 2012;103:22-32. [Crossref] [PubMed]
- Karasawa K, Tanaka A, Jung K, et al. Patterns of aquaporin expression in the canine eye. Vet J 2011;190:e72-7. [Crossref] [PubMed]
- Kuo IC. Corneal wound healing. Curr Opin Ophthalmol 2004;15:311-5. [Crossref] [PubMed]
- Choi JH, Greene WA, Johnson AJ, et al. Pathophysiology of blast-induced ocular trauma in rats after repeated exposure to low-level blast overpressure. Clin Exp Ophthalmol 2015;43:239-46. [Crossref] [PubMed]
- Bricker-Anthony C, Hines-Beard J, D'Surney L, et al. Exacerbation of blast-induced ocular trauma by an immune response. J Neuroinflammation 2014;11:192. [Crossref] [PubMed]
- Ruff AL, Jarecke AJ, Hilber DJ, et al. Development of a mouse model for sulfur mustard-induced ocular injury and long-term clinical analysis of injury progression. Cutan Ocul Toxicol 2013;32:140-9. [Crossref] [PubMed]
- Gu XJ, Liu X, Chen YY, et al. Involvement of NADPH oxidases in alkali burn-induced corneal injury. Int J Mol Med 2016;38:75-82. [Crossref] [PubMed]
- Poon MW, Yan L, Jiang D, et al. Inhibition of RAP1 enhances corneal recovery following alkali injury. Invest Ophthalmol Vis Sci 2015;56:711-21. [Crossref] [PubMed]
- Okada Y, Shirai K, Reinach PS, et al. TRPA1 is required for TGF-β signaling and its loss blocks inflammatory fibrosis in mouse corneal stroma. Lab Invest 2014;94:1030-41. [Crossref] [PubMed]
- Yabuuchi N, Sagata M, Saigo C, et al. Indoxyl Sulfate as a Mediator Involved in Dysregulation of Pulmonary Aquaporin-5 in Acute Lung Injury Caused by Acute Kidney Injury. Int J Mol Sci 2016;18:11. [Crossref] [PubMed]
- Sun CY, Zhao YX, Zhong W, et al. The expression of aquaporins 1 and 5 in rat lung after thoracic irradiation. J Radiat Res 2014;55:683-9. [Crossref] [PubMed]
- Sebastian R, Chau E, Fillmore P, et al. Epidermal aquaporin-3 is increased in the cutaneous burn wound. Burns 2015;41:843-7. [Crossref] [PubMed]
- Kubo H, Hayashi T, Ago K, et al. Forensic diagnosis of ante- and postmortem burn based on aquaporin-3 gene expression in the skin. Leg Med (Tokyo) 2014;16:128-34. [Crossref] [PubMed]
- Li W, Zha L, Li Z, et al. The expression profile of aquaporin 1 in rat myocardium after severe burns. Turk J Med Sci 2015;45:481-8. [Crossref] [PubMed]
- Fan Q, Zhao P, Li J, et al. 17β-Estradiol administration attenuates seawater aspiration-induced acute lung injury in rats. Pulm Pharmacol Ther 2011;24:673-81. [Crossref] [PubMed]
- Tran TL, Hamann S, Heegaard S. Aquaporins in the Eye. Adv Exp Med Biol 2017;969:193-8. [Crossref] [PubMed]
- Kumari SS, Varadaraj M, Menon AG, et al. Aquaporin 5 promotes corneal wound healing. Exp Eye Res 2018;172:152-8. [Crossref] [PubMed]
- Levin MH, Verkman AS. Aquaporin-3-dependent cell migration and proliferation during corneal re-epithelialization. Invest Ophthalmol Vis Sci 2006;47:4365-72. [Crossref] [PubMed]
- Macnamara E, Sams GW, Smith K, et al. Aquaporin-1 expression is decreased in human and mouse corneal endothelial dysfunction. Mol Vis 2004;10:51-6. [PubMed]
- Shen Y, Wang Y, Chen Z, et al. Role of aquaporin 5 in antigen-induced airway inflammation and mucous hyperproduction in mice. J Cell Mol Med 2011;15:1355-63. [Crossref] [PubMed]
- Ikezoe K, Oga T, Honda T, et al. Aquaporin-3 potentiates allergic airway inflammation in ovalbumin-induced murine asthma. Sci Rep 2016;6:25781. [Crossref] [PubMed]
- Rabolli V, Wallemme L, Lo Re S, et al. Critical role of aquaporins in interleukin 1β (IL-1β)-induced inflammation. J Biol Chem 2014;289:13937-47. [Crossref] [PubMed]
- Tyteca D, Nishino T, Debaix H, et al. Regulation of macrophage motility by the water channel aquaporin-1: crucial role of M0/M2 phenotype switch. PLoS One 2015;10:e0117398. [Crossref] [PubMed]
- Vassiliou AG, Maniatis NA, Orfanos SE, et al. Induced expression and functional effects of aquaporin-1 in human leukocytes in sepsis. Crit Care 2013;17:R199. [Crossref] [PubMed]
- Musumeci G, Leonardi R, Carnazza ML, et al. Aquaporin 1 (AQP1) expression in experimentally induced osteoarthritic knee menisci: an in vivo and in vitro study. Tissue Cell 2013;45:145-52. [Crossref] [PubMed]
- Mezzasoma L, Cagini L, Antognelli C, et al. TNF-α regulates natriuretic peptides and aquaporins in human bronchial epithelial cells BEAS-2B. Mediators Inflamm 2013;2013:159349. [Crossref] [PubMed]
- Dicay MS, Hirota CL, Ronaghan NJ, et al. Interferon-γ suppresses intestinal epithelial aquaporin-1 expression via Janus kinase and STAT3 activation. PLoS One 2015;10:e0118713. [Crossref] [PubMed]
(English Language Editor: J. Teoh)