The silencing of miR-199a-5p protects the articular cartilage through MAPK4 in osteoarthritis
Introduction
Osteoarthritis (OA) is a very common form of musculoskeletal disease, and its risk factors include aging, gender, obesity, and chronic systemic diseases (1-4). With aging and the increased prevalence of obesity, the prevalence of OA continues to increase year by year, and currently, about 250 million individuals have OA worldwide (1,5). Despite great advances in OA treatments, including intra-articular injection, oral administration, and surgery, there is still no cure for OA (6,7). Notably, gene therapies and small-molecule therapies based on the pathogenesis of the disorder represent a promising alternative treatment option for OA, and it may be an important way to treat OA in the future. In fact, autophagy is thought to play a key role in osteoarthritis. A new study shows that inhibiting autophagy in chondrocytes may accelerate the progression of osteoarthritis (8). Li et al. Also found that inducing autophagy of chondrocytes is conducive to reducing cartilage loss and matrix degradation(9). However, the mechanism of autophagy in osteoarthritis is still unclear. Thus, it is essential to further unravel the mechanism and pathological changes of the disorder.
Micro ribonucleic acids (miRNAs), a type of non-coding RNAs (ncRNAs), are widely studied in the human body, and ncRNAs also include long non-coding RNAs and circular RNAs (ribonucleic acid) (10,11). As we all know, ncRNAs, including miRNAs, pathologically regulate gene transcription and translation by RNA/protein sponging and epigenetic alternation, and thus participate in various cellular processes (11-15). Research has shown that some miRNAs take part in the pathogenesis and progression of diverse diseases, such as cancers (13) and neurodegenerative diseases (16), and mediate the process of inflammation (17,18). miRNAs also play a crucial role in OA and osteoporosis. For example, research has shown that intra-articularly injected miR-140-5p enters the cartilage and restrains the progression of early OA in rats (19). As a matter of fact, miR-199a-5p has been found to be increasing in kee osteoarthritis (20). Wu et al. found that inhibition of miR-199a-5p expression alleviated synovitis caused by osteoarthritis (21). However, the mechanism of miR-199a-5p in osteoarthritis remains to be explored.
The mitogen-activated protein kinase (MAPK) family exerts a core effect on the signal cascade, and mediates cellular responses, including the cell cycle, proliferation, chromatin remodeling (22,23). Previous study on MAPKs have focused on MAPK3/1 (ERK1/2), MAPK8/9/10 (JNK1/2/3) (c-Jun N-terminal kinase 1/2/3), and MAPK14/11/12/13 (P38α/β/γ/δ), but only a few studies have examined the role of MAPK4 (24). MAPK4 (also known as ERK4, or p63 MAPK) is an atypical MAPK located on chromosome 18q12-21 (25). Structurally, MAPK4 lacks the Thr-X-Tyr activation motif, but contains the SEG (single exon gene) sequence and SPR motif, and thus cannot be phosphorylated by dual Ser/Thr and Tyr MAPK kinase (23,26,27). Research has shown the significance of MARK in the progression of several diseases, including prostate cancer (28), triple negative breast cancer (29), and cervical cancer (23). Additionally, MAPK4 could serve as a new modulator of acute lung injury (30). Thus, research on MAPK4 may extend understandings of the disease mechanism. However, to date, the function of MAPK4 in OA remains unknown.
This study sought to screen the differentially expressed miRNAs in OA, explore the potential functions of these miRNAs in OA and investigate the underlying mechanism of OA to provide novel therapeutic strategies for treating OA. We present the following article in accordance with the ARRIVE reporting checklist (available at https://atm.amegroups.com/article/view/10.21037/atm-22-2057/rc).
Methods
Animal model of OA
The Nanfang South Medical University Animal Resource Center provided the rats (which weighed 300–350 g and were aged 11–12 weeks old). The rats underwent a transection of the anterior and posterior cruciate ligament, medial collateral ligament, and medial meniscus of the knee to establish the rat knee OA model. On the 7th day post-surgery, the rats were gathered to increase the weight of the surgical limbs and accelerate the cartilage degeneration. For the treatment group, the joints of the rats were injected with miR-199a-5p antagomir (dose: 50 nmol/L; volume: 200 µL) every week after modeling. The blood of the rats was collected, and the serum was obtained at the 6th and 12th week for the RNA-sequencing analysis. Animal experiments were performed under a project license (No. RH2021-05-02) granted by ethics committee of Guangdong Second Provincial General Hospital, in compliance with Guangdong Second Provincial General Hospital guidelines for the care and use of animals.
Library prep and miRNAs sequencing
miRNA was extracted from the joint tissues using a Qiagen miRNeasy Kit. The TruSeq quick SBS kit (Illumina, San Diego, CA, USA) was used in accordance with the manufacturer’s instructions to construct a small RNA-sequencing library. For the specifically enriched miRNAs, the RNAs were separated by 4–20% sodium dodecyl-sulfate polyacrylamide gel electrophoresis (SDS-PAGE) and eluted from the gel. The Agilent 2100 bioanalyzer was used to measure the quality of the miRNAs, and the sequencing was made using Illumina HiSeq2500 (Illumina, San Diego, CA, USA).
The raw information was filtered with fastx (version: 0.0.13; (http://hannonlab.cshl.ed u/fastx_toolkit/index.html) to remove any unqualified reads of low quality, sequencing primers, or low end quality. To identify the differentially expressed miRNAs, a principal component analysis was conducted on the miRNA-sequencing data set. After quality control, 9 samples from the small RNA-sequencing data set were included in the downstream analysis.
Gene target and pathway enrichment analysis
The differentially expressed miRNAs were analyzed from the collected samples using edgeR (31). Multiple hypothesis testing was conducted to examine and adjust the P value and determine the beginning of the P value (a parameter to restrict false positive rate) by restricting the false discovery rate (32,33). The q-value, and adjusted P value were also calculated. Additionally, we calculated the fold-change of differentially expressed miRNAs based on the TPM (Transcripts Per Million) value and screened the differentially expressed miRNAs with a P value ≤0.05 and a fold-change ≥2.
miRNA-mRNA
The targeted binding of the differentially expressed miRNA and messenger RNA (mRNA) 3 prime untranslated region (3'UTR) was forecast using miRanda (http://www.microrna.org/microrna/home.do) (34). The miRTarBase (http://mirtarbase.mbc.nctu.edu.tw/index.html) was used to summarize the known target genes of the differentially expressed miRNAs (35). Gene ontology (GO) and Kyoto Encyclopedia of Genes and Genomes (KEGG) pathway enrichment analyses were subsequently conducted with DIANA-miRPath v 3.0 (http://diana.imis.athena-innovati on.gr/DianaTools/index.php) (36).
Reverse transcription-quantitative polymerase chain reaction (qRT-PCR)
Th overall RNA from the tissues and cells was extracted using TRIzol reagent (Invitrogen, Carlsbad, CA, USA), and then subjected to reverse transcription using the PrimeScript RT Reagent Kit (TaKaRa, Tokyo, Japan). The complementary RNA underwent a qRT-PCR analysis using the Thermal Cycler CFX6 System (Bio-Rad, Hercules, CA, USA), and the SYBR Green PCR Kit (TaKaRa). After normalizing miR-199a-5p to U6, the gene level was measured using the 2-ΔΔCt method.
Cell culture
At 7 days after birth, the primary chondrocytes were taken from 5 Sprague-Dawley rats (three male and two female). The digestion of the articular cartilage from the end of the tibia and femur was performed with 0.2% (w/v) type-II collagenase, and cultured in a medium with F12 and 10% (w/v) fetal bovine serum (FBS) and 1% (w/v) penicillin/streptomycin. The seeding of the chondrocytes at the first passage was made on glass slides in 24-well plates for the immunofluorescence analysis. The chondrocytes were treated with miR-199a-5p antagomir or agomir for the subsequent experiments 24 hours after the cell inoculation.
Immunofluorescence
After the cells adhered to the walls, the cells were seeded and exposed to 5Gy radiation. After being fixed with 4% paraformaldehyde, the SiHa or caSki cells were permeabilized in 0.1% Triton X-100. Next, the cells were blocked with 5% bovine serum albumin, and then underwent an overnight probe with anti-vimentin or anti-LC3 II primary antibodies at 4 ℃ and fluorescein isothiocyanate-conjugated secondary antibodies. To visualize the nuclear deoxyribonucleic acid, the cell nuclei were stained with 4',6-Diamidino-2-Phenylindole (Sigma-Aldrich, St. Louis, MO, USA), and then photographed using a confocal microscope (Nikon).
CCK-8 assays
Approximately 1×103 cells was seeded in 96-well plates, and then cultured for 0, 24, and 72 hours. A Cell Counting Kit-8 (CCK-8) (Dojindo Molecular Technology) was used to evaluate cell viability. CCK-8 reagent (10 µL) was added to every well, and the cells were incubated at 37 ℃ for 2 hours. The optical density at 450 nm was examined using an ELx800 absorbance microplate reader (BioTek Instruments).
EdU assay
The chondrocytes were seeded in a 6-well plate, and then incubated overnight. Subsequently, the cells were treated for 24 hours with a miR-199a-5p antagomir or agomir. Cell proliferation was then examined using a 5-ethynyl-2'-deoxyuridine (EdU) test kit (KTA2030; Abbkine, USA) in accordance with the manufacturer’s instructions.
Western blot
The chondrocytes were lysed with pre-chilled radioimmunoprecipitation assay buffer lysis buffer (Beyotime), and then quantified using a bicinchoninic acid protein detection kit (Solarbio, Beijing, China). The protein samples (50 µg) were isolated by 10% SDS-PAGE, and the nitrocellulose membranes were transfected (Millipore, New York, NY, USA). Next, the membranes were incubated overnight with primary antibodies at 4 ℃, and then with secondary antibodies for 2 hours at room temperature. The membranes were developed by chemiluminescence signal with an enhanced chemiluminescence imaging kit (Thermo Fisher Scientific, Waltham, MA, USA, Cat. #32209).
Target prediction
To explore the mechanism by which miR-199a-5p affects OA, the TargetScan (http://www.targetscan.org) and StarBase (http://starbase.sysu.edu.cn/) databases were used to predict the target genes of miR-199a-5p.
Dual-luciferase reporter gene assay
To assess the binding association between miR-199a-5p and MAPK4, a fragment sequence with the putative binding site of MAPK4 3'UTR and miR-199a-5p and the corresponding mutation site was probed into pmirGLO plasmid (Promega, Madison, WI, USA) to construct the WT-MAPK4 and MUT-MAPK4 vectors. The constructed vectors were then transfected into chondrocytes with miR-199a-5p or NC (Negative control)mimic with Lipofectamine 3000 reagent (Invitrogen). After 48 hours, the dual-luciferase reporter system (Promega Corporation, USA) was used to assess luciferase activity.
shRNA
Sigma-Aldrich (St. Louis, Missouri, USA) provided short-hairpinRNA(ShRNA) targeting MAPK4, which was transfected in accordance with the manufacturer’s instructions. shRNA sequences: CGGCTGCATCCTGGCTGAGATGCdTdT.
Safranin O-fast green staining
The rat knee joints were fixed overnight in 4% paraformaldehyde, after which they were dehydrated, transparentized, and embedded in paraffin. Next, the paraffin was cut into 5-µm sections and collected on a glass slide. The sections were stained using the Safranin O/Fast Green staining kit (ICH World, Woodstock, MD, USA), and the specimens were then photographed under an inverted microscope.
Hematoxylin and eosin staining
The rat cartilage tissues were fixed for 12 hours in 4% paraformaldehyde phosphate buffer, followed by deparaffinization and gradient alcohol hydration (with absolute ethanol, 95% ethanol, and 75% ethanol for 3 minutes). After being boiled in 0.01 M citrate buffer for 15–20 minutes, the slides were sealed with goat serum blocking solution, and the excessive liquid was removed. The tissues were stained with hematoxylin and differentiated with hydrochloric acid and ethanol. After routine dehydration, the tissues were sealed and observed under an inverted microscope.
ELISA
Enzyme-linked immunosorbent assays (ELISAs) were conducted to assess the levels of interleukin (IL)-1β, IL-6, and IL-18 using an ELISA kit (R&D Systems, Minneapolis, MN, USA) in accordance with the manufacturer’s instructions.
Statistical analysis
The data are presented as the mean ± standard deviation, and the p values were determined using SPSS v22.0 with a 2-tailed unpaired Student’s t-test (95% confidence interval). A P value <0.05 indicated a statistically significant difference.
Results
Establishment of the OA model and identification of differentially expressed miRNAs in OA
To study the abnormal expression of miRNAs in OA, a rat OA model was established through surgery, and rat serum was collected at 6 and 12 weeks for miRNA sequencing (see Figure 1A,1B). The sequencing results showed 10upregulated miRNAs and 10 downregulated miRNAs. The qRT-PCR analysis of the upregulated miRNAs revealed that the expression levels of multiple miRNAs increased at 12 weeks, and the expression levels of miR-199a-5p increased the most significantly (see Figure 1C).
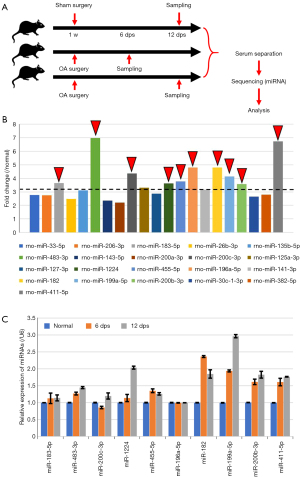
A bioinformatics analysis was conducted using the miRanda and miRTarBase database for the targeted binding prediction of the 3'UTR of differentially expressed miRNAs and mRNAs. The GO and KEGG analyses were subsequently conducted on the target genes (see Figure 2A,2B).
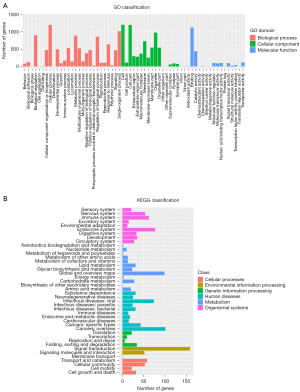
MiR-199a-5p is a significant regulator of chondrocyte proliferation and survival
The rat articular chondrocytes were isolated for the primary cultures, and immunofluorescence staining was conducted to identify the morphology of chondrocytes (see Figure 3A,3B). The chondrocytes were transfected for 48 hours with antagomiRs targeting miR-199a-5p, and the outcomes of the qRT-PCR analysis confirmed that the expression of miR-199a-5p decreased after the antagomir treatment (see Figure 3C). The CCK-8 and Edu proliferation tests showed that the knockdown of miR-199a-5p greatly increased the cell proliferation rate (see Figure 3D,3E). Additionally, the Western blot analysis revealed that upon treatment with antagomiRs, the expression of LC3B and Beclin 1 decreased and the expression of P62 increased, indicating that the downregulation of miR-199a-5p inhibited autophagy. Thus, our findings indicated that decreasing the expression of miR-199a-5p increased the proliferation and survival of chondrocytes (see Figure 3F).
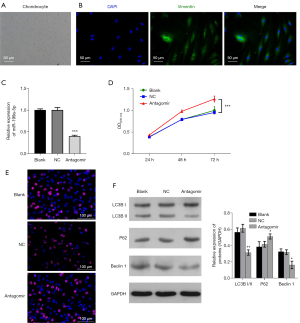
qRT-PCR was used to confirm the overexpression of miR-199a-5p by an agomir in chondrocytes (see Figure 4A), cell proliferation was decreased (see Figure 4B,4C). Additionally, the immunofluorescence staining, and Western blot analysis showed that the overexpression of miR-199a-5p led to an increase in autophagy (see Figure 4D,4E). Our findings confirm to the outcomes of antagomir treatment, and jointly prove that miR-199a-5p exerts a key effect on the proliferation and survival of chondrocytes.
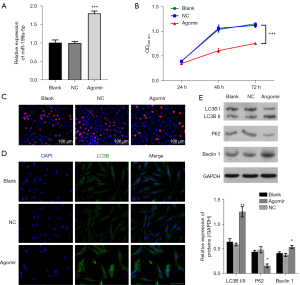
MiR-199a-5p targets MAPK4
To further examine the role of miR-199a-5p in chondrocytes, the target genes of miR-199a-5p were forecast with the TargetScan and StarBase databases, and the results revealed that MAPK4 may be the target gene of miR-199a-5p (see Figure 5A). To verify the interaction between MAPK4 and miR-199a-5p, wild-type and mutant MAPK4 were co-transfected into 293T cells. The results of the dual-luciferase reporter gene assays revealed that miR-199a-5p binds to MAPK4 and inhibits MARPK4 expression (see Figure 5B). When the chondrocytes were overexpressed for miR-199a-5p following the transfection with the agomir, the protein expression of MAPK4 was decreased; however, the protein expression of MAPK4 expression was increased after antagomir treatment (see Figure 5C). These results indicate that miR-199a-5p binds to the 3'UTR of MAPK4 and inhibits the expression of MAPK4.
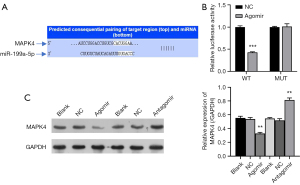
To further verify the effect of miR-199a-5p-MAPK4 on the growth of chondrocytes, human articular chondrocytes (HACs) were selected for the follow-up experiments and first treated with LPS (Lipopolysaccharide). As LPS can induce cell apoptosis and inflammation, LPS treatment decreases cell proliferation significantly (30). Notably, the miR-199a-5p antagomir treatment rescued the HAC proliferation, but when MAPK4 expression was silenced through shRNA treatment, the cell proliferation decreased (see Figure 6A,6B). Thus, miR-199a-5p functions by targeting MAPK4. Similarly, the expression of the autophagy-related protein LC3B increased after treatment with MAPK4shRNA (see Figure 6C). The electron microscopy results also revealed that the inhibition of miR-199a-5p decreased the production of autophagosome (see Figure 6D). Next, after examining the effect of miR-199a-5p-MAPK4 on the expression of key genes in the chondrocytes, the results revealed that silencing miR-199a-5p targets MAPK4 to regulate the protein expression of Collagen II, MMP13 (matrix metalloproteinases 13), and ADAMTS5 (ADAM metallopeptidase with thrombospondin type 1 motif 5) (Figure 6E).
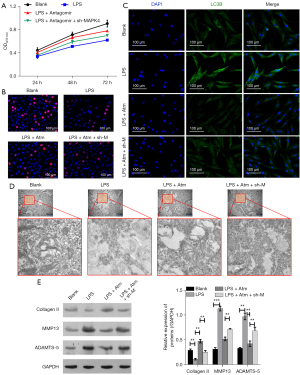
Controlling miR-199a-5p protects articular cartilage and improves gait abnormality
To further examine the role of miR-199-5p in OA, we established a rat knee OA model through the incision of the anterior and posterior cruciate ligaments, medial collateral ligaments, and medial meniscus. The rats in the treatment group were then injected with miR-199a-5p antagomir every week. The staining results of the knee joints revealed that the silencing of miR-199a-5p effectively decreased inflammation (see Figure 7A,7B). Further, the ELISA assays showed that inhibiting the expression of miR-199a-5p decreased the expression of the pro-inflammatory cytokines, IL-1β, IL-6, and IL-18 (see Figure 7C). Similarly, treatment with the miR-199a-5p antagomir in vivo also regulated the protein expression of Collagen II, MMP13, and ADAMTS5 (see Figure 7D), which supports the findings of the in-vitro experiments. Thus, our results indicate that miR-199a-5p is involved in the progression of OA by targeting MAPK4.
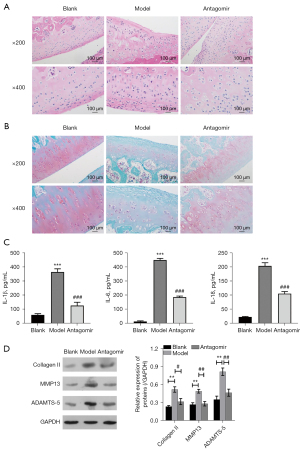
Discussion
The use of intra-articular administration to treat OA appears to have more advantages than systemic administration, including lower costs, fewer adverse reactions, and higher local utilization. Currently, hyaluronic acid (HA) and glucocorticoids (GCs) are commonly used drugs, but their effects on OA are still controversial. Dexamethasone has been shown to save cartilage matrix loss and chondrocyte viability in OA, but it also damages healthy cartilage at some doses and durations (37). Recent clinical trials have found in consistent results on the safety and effectiveness of GCs, but it should be noted that these clinical trials employed different methods (38). HA enables OA to recover the rheological nature of synovial fluid, thereby relieving the injury and improving functionality, but its efficacy remains controversial. GC injections (rather than HA intra-articular injections) are often recommended for knee OA and thumb-base OA (7,39,40). Other methods of intra-articular treatment, including small-molecule drugs, stem-cell therapy, and growth-factor therapy, are still under clinical development. In recent years, miRNAs have shown promise as biomarkers, as their expressions are closely related to the progression of OA (41). However, the mechanism underlying miRNAs in OA remains unclear. This research sought to identify differentially expressed miRNAs in OA, and then explore their functions and potential regulatory mechanisms.
We found that miR-199a-5p exerted a key effect on the OA rat model in vivo and in vitro. With the high expression of miR-199a-5p in the chondrocytes of OA rats, the control of miR-199a-5p increased the proliferation of chondrocytes and induced autophagy, while the overexpression of miR-199a-5p aggravated the progression of chondrocytes. At present, many miRNA studies related to OA have been based on bioinformatics analyses or the results of previous studies. The present study screened the differentially expressed miRNAs in a OA rat model by eukaryotic miRNA-sequencing analysis and then miRNAs were detected by qRT-PCR. Our results indicate that miR-199a-5p had the most obvious expression. Previous sequencing studies have also noted the abnormal expression of miR-199a-5p in OA at different stages, and it is believed that the expression of miR-199a-5p in late OA is significantly lower than that in early OA. However, these studies failed to clearly elucidate the functional verification of miR-199a-5p and its downstream targets, and the difference between OA and normal tissues has not yet been explored (20,42). Notably, it is likely that miR-199a-5p exerts a different function in the pathology of early OA and late OA, and we intend to examine this further in future research.
Additionally, this research further showed that MAPK4 is the downstream target of miR-199a-5p, as knocking down MAPK4 abrogated the role of miR-199a-5p in cell proliferation and autophagy. Further, miR-199a-5p regulates Collagen II, MMP13, and ADAMTS5 expression requiring MAPK4. The significance of MAPK4 (an atypical MAPK) has been noted in various cancers, but its function in OA is not yet known. Our research suggests that MAPK4 may drive the function of miR-199a-5p.
Cartilage loss is the leading cause of OA. Cartilage cells are enclosed by a large amount of extracellular matrix, of which collagen and aggrecan are important components (43). The deterioration of aggrecan is an early event in OA pathology, and the degradation and loss of collagen are irreversible (44). The ADAMTS family comprises the aggrecanase involved in cartilage degradation in OA. ADAMTS-5 is the main aggrecanase involved in cartilage catabolism, and its potency is about 30 times that of ADAMTS-4 (45,46). Collagen II is the main collagen in cartilage ECM, and is extremely resistant to degradation by most proteases, but it is degraded by collagenases, including MMP-1, MMP-8, and MMP-13 (43). MMP-13 is the primary collagenase involved in OA, and its expression increases in OA cartilage (47,48). Our outcomes showed that inhibiting miR-199a-5p protected Collagen II and decreased the expression of MMP13 and ADAMTS-5.
IL-1β, IL-6, and IL-18 are all inflammatory cytokines involved in the pathogenesis of OA. IL-1β has the function of inducing inflammation and catabolism, and it is associated with Toll-like receptors. IL-1β transmits the signal to the adaptor protein MyD88 to recruit TRAF6 and the downstream IKK kinase complex, stimulating the transcription element NF-κB and enhancing inflammatory response (49-51). IL-1β also affects the synthesis of MMP13 and ADAMTS-4 by chondrocytes (52,53), promotes the formation of osteoclasts, and is crucial to variations in the subchondral bone layer (54). Additionally, IL-6 acts synergistically with IL-1 β to promote the formation of MMPs and reduces the production of Collagen II (55). IL-18 also induces the destruction of chondrocytes by promoting the excessive synthesis of MMPs and inhibiting the synthesis of Aggrecan (56,57). Our research shows that the intra-articular injection of the miR-199a-5p antagomir significantly decreased the level of IL-1β, IL-6, and IL-18.
Conclusions
Collectively, the current research showed that the silencing of miR-199a-5p protects the articular cartilage in OA by regulating MAPK4, improves gait abnormalities, and restrains the progress of OA. Inhibiting the proliferation and anti-inflammatory effects of miR-199a-5p may be an effective way to relieve OA, and our findings have certain clinical significance.
Acknowledgments
Funding: The research was supported by grant from the National Science and Technology Major Project (No. 2017YFC0806400).
Footnote
Reporting Checklist: The authors have completed the ARRIVE reporting checklist. Available at https://atm.amegroups.com/article/view/10.21037/atm-22-2057/rc
Data Sharing Statement: Available at https://atm.amegroups.com/article/view/10.21037/atm-22-2057/dss
Conflicts of Interest: All authors have completed the ICMJE uniform disclosure form (available at https://atm.amegroups.com/article/view/10.21037/atm-22-2057/coif). All authors report that the research was supported by grants from the National Science and Technology Major Project (No. 2017YFC0806400). The authors have no other conflicts of interest to declare.
Ethical Statement: The authors are accountable for all aspects of the work in ensuring that questions related to the accuracy or integrity of any part of the work are appropriately investigated and resolved. Animal experiments were performed under a project license (No. RH2021-05-02) granted by ethics committee of Guangdong Second Provincial General Hospital, in compliance with Guangdong Second Provincial General Hospital guidelines for the care and use of animals.
Open Access Statement: This is an Open Access article distributed in accordance with the Creative Commons Attribution-NonCommercial-NoDerivs 4.0 International License (CC BY-NC-ND 4.0), which permits the non-commercial replication and distribution of the article with the strict proviso that no changes or edits are made and the original work is properly cited (including links to both the formal publication through the relevant DOI and the license). See: https://creativecommons.org/licenses/by-nc-nd/4.0/.
References
- Hu Y, Chen X, Wang S, et al. Subchondral bone microenvironment in osteoarthritis and pain. Bone Res 2021;9:20. [Crossref] [PubMed]
- Blüher M. Metabolically Healthy Obesity. Endocr Rev 2020;41:bnaa004.
- Jeon OH, David N, Campisi J, et al. Senescent cells and osteoarthritis: a painful connection. J Clin Invest 2018;128:1229-37. [Crossref] [PubMed]
- Jamshidi A, Pelletier JP, Martel-Pelletier J. Machine-learning-based patient-specific prediction models for knee osteoarthritis. Nat Rev Rheumatol 2019;15:49-60. [Crossref] [PubMed]
- Latourte A, Kloppenburg M, Richette P. Emerging pharmaceutical therapies for osteoarthritis. Nat Rev Rheumatol 2020;16:673-88. [Crossref] [PubMed]
- Grandi FC, Bhutani N. Epigenetic Therapies for Osteoarthritis. Trends Pharmacol Sci 2020;41:557-69. [Crossref] [PubMed]
- Jones IA, Togashi R, Wilson ML, et al. Intra-articular treatment options for knee osteoarthritis. Nat Rev Rheumatol 2019;15:77-90. [Crossref] [PubMed]
- Zhang S, Jin Z. Bone Mesenchymal Stem Cell-Derived Extracellular Vesicles Containing Long Noncoding RNA NEAT1 Relieve Osteoarthritis. Oxid Med Cell Longev 2022;2022:5517648. [Crossref] [PubMed]
- Li M, Peng Z, Wang X, et al. Monoamine oxidase A attenuates chondrocyte loss and extracellular matrix degradation in osteoarthritis by inducing autophagy. Int Immunopharmacol 2022; [Epub ahead of print]. [Crossref] [PubMed]
- Ma P, Pan Y, Li W, et al. Extracellular vesicles-mediated noncoding RNAs transfer in cancer. J Hematol Oncol 2017;10:57. [Crossref] [PubMed]
- Dragomir MP, Kopetz S, Ajani JA, et al. Non-coding RNAs in GI cancers: from cancer hallmarks to clinical utility. Gut 2020;69:748-63. [Crossref] [PubMed]
- Tanwar VS, Reddy MA, Natarajan R. Emerging Role of Long Non-Coding RNAs in Diabetic Vascular Complications. Front Endocrinol (Lausanne) 2021;12:665811. [Crossref] [PubMed]
- Zhang L, Xu X, Su X. Noncoding RNAs in cancer immunity: functions, regulatory mechanisms, and clinical application. Mol Cancer 2020;19:48. [Crossref] [PubMed]
- Forero A, So L, Savan R. Re-evaluating Strategies to Define the Immunoregulatory Roles of miRNAs. Trends Immunol 2017;38:558-66. [Crossref] [PubMed]
- Song P, Yang F, Jin H, et al. The regulation of protein translation and its implications for cancer. Signal Transduct Target Ther 2021;6:68. [Crossref] [PubMed]
- Oliveira SR, Dionísio PA, Gaspar MM, et al. miR-335 Targets LRRK2 and Mitigates Inflammation in Parkinson's Disease. Front Cell Dev Biol 2021;9:661461. [Crossref] [PubMed]
- Akbaba TH, Akkaya-Ulum YZ, Tavukcuoglu Z, et al. Inflammation-related differentially expressed common miRNAs in systemic autoinflammatory disorders patients can regulate the clinical course. Clin Exp Rheumatol 2021;39:109-17. [Crossref] [PubMed]
- Jiao P, Wang XP, Luoreng ZM, et al. miR-223: An Effective Regulator of Immune Cell Differentiation and Inflammation. Int J Biol Sci 2021;17:2308-22. [Crossref] [PubMed]
- Cao F, Chen Y, Wang X, et al. Therapeutic effect and potential mechanisms of intra-articular injections of miR-140-5p on early-stage osteoarthritis in rats. Int Immunopharmacol 2021;96:107786. [Crossref] [PubMed]
- Ali SA, Gandhi R, Potla P, et al. Sequencing identifies a distinct signature of circulating microRNAs in early radiographic knee osteoarthritis. Osteoarthritis Cartilage 2020;28:1471-81. [Crossref] [PubMed]
- Wu MH, Tsai CH, Huang YL, et al. Visfatin Promotes IL-6 and TNF-α Production in Human Synovial Fibroblasts by Repressing miR-199a-5p through ERK, p38 and JNK Signaling Pathways. Int J Mol Sci 2018;19:190. [Crossref] [PubMed]
- Johnson GL, Lapadat R. Mitogen-activated protein kinase pathways mediated by ERK, JNK, and p38 protein kinases. Science 2002;298:1911-2. [Crossref] [PubMed]
- Tian S, Lou L, Tian M, et al. MAPK4 deletion enhances radiation effects and triggers synergistic lethality with simultaneous PARP1 inhibition in cervical cancer. J Exp Clin Cancer Res 2020;39:143. [Crossref] [PubMed]
- Wang W, Shen T, Dong B, et al. MAPK4 overexpression promotes tumor progression via noncanonical activation of AKT/mTOR signaling. J Clin Invest 2019;129:1015-29. [Crossref] [PubMed]
- Gonzalez FA, Raden DL, Rigby MR, et al. Heterogeneous expression of four MAP kinase isoforms in human tissues. FEBS Lett 1992;304:170-8. [Crossref] [PubMed]
- Aberg E, Perander M, Johansen B, et al. Regulation of MAPK-activated protein kinase 5 activity and subcellular localization by the atypical MAPK ERK4/MAPK4. J Biol Chem 2006;281:35499-510. [Crossref] [PubMed]
- Kant S, Schumacher S, Singh MK, et al. Characterization of the atypical MAPK ERK4 and its activation of the MAPK-activated protein kinase MK5. J Biol Chem 2006;281:35511-9. [Crossref] [PubMed]
- Shen T, Wang W, Zhou W, et al. MAPK4 promotes prostate cancer by concerted activation of androgen receptor and AKT. J Clin Invest 2021;131:135465. [Crossref] [PubMed]
- Zeng X, Jiang S, Ruan S, et al. MAPK4 silencing together with a PARP1 inhibitor as a combination therapy in triplenegative breast cancer cells. Mol Med Rep 2021;24:548. [Crossref] [PubMed]
- Mao L, Zhou Y, Chen L, et al. Identification of atypical mitogen-activated protein kinase MAPK4 as a novel regulator in acute lung injury. Cell Biosci 2020;10:121. [Crossref] [PubMed]
- Robinson MD, McCarthy DJ, Smyth GK. edgeR: a Bioconductor package for differential expression analysis of digital gene expression data. Bioinformatics 2010;26:139-40. [Crossref] [PubMed]
- Benjamini Y, Hochberg Y. Controlling the False Discovery Rate: A Practical and Powerful Approach to Multiple Testing. Journal of the Royal Statistical Society Series B (Methodological) 1995;57:289-300. [Crossref]
- Yoav B, Daniel Y. The control of the false discovery rate in multiple testing under dependency. The Annals of Statistics 2001;29:1165-88.
- Betel D, Wilson M, Gabow A, et al. The microRNA.org resource: targets and expression. Nucleic Acids Res 2008;36:D149-53. [Crossref] [PubMed]
- Hsu SD, Lin FM, Wu WY, et al. miRTarBase: a database curates experimentally validated microRNA-target interactions. Nucleic Acids Res 2011;39:D163-9. [Crossref] [PubMed]
- Vlachos IS, Zagganas K, Paraskevopoulou MD, et al. DIANA-miRPath v3.0: deciphering microRNA function with experimental support. Nucleic Acids Res 2015;43:W460-6. [Crossref] [PubMed]
- Black R, Grodzinsky AJ. Dexamethasone: chondroprotective corticosteroid or catabolic killer? Eur Cell Mater 2019;38:246-63. [Crossref] [PubMed]
- Conaghan PG, Hunter DJ, Cohen SB, et al. Effects of a Single Intra-Articular Injection of a Microsphere Formulation of Triamcinolone Acetonide on Knee Osteoarthritis Pain: A Double-Blinded, Randomized, Placebo-Controlled, Multinational Study. J Bone Joint Surg Am 2018;100:666-77. [Crossref] [PubMed]
- Tenti S, Cheleschi S, Mondanelli N, et al. New Trends in Injection-Based Therapy for Thumb-Base Osteoarthritis: Where Are We and where Are We Going? Front Pharmacol 2021;12:637904. [Crossref] [PubMed]
- Kolasinski SL, Neogi T, Hochberg MC, et al. 2019 American College of Rheumatology/Arthritis Foundation Guideline for the Management of Osteoarthritis of the Hand, Hip, and Knee. Arthritis Rheumatol 2020;72:220-33. [Crossref] [PubMed]
- Jones TL, Esa MS, Li KHC, et al. Osteoporosis, fracture, osteoarthritis & sarcopenia: A systematic review of circulating microRNA association. Bone 2021;152:116068. [Crossref] [PubMed]
- Prasadam I, Batra J, Perry S, et al. Systematic Identification, Characterization and Target Gene Analysis of microRNAs Involved in Osteoarthritis Subchondral Bone Pathogenesis. Calcif Tissue Int 2016;99:43-55. [Crossref] [PubMed]
- Troeberg L, Nagase H. Proteases involved in cartilage matrix degradation in osteoarthritis. Biochim Biophys Acta 2012;1824:133-45. [Crossref] [PubMed]
- Karsdal MA, Madsen SH, Christiansen C, et al. Cartilage degradation is fully reversible in the presence of aggrecanase but not matrix metalloproteinase activity. Arthritis Res Ther 2008;10:R63. [Crossref] [PubMed]
- Yamamoto K, Wilkinson D, Bou-Gharios G. Targeting Dysregulation of Metalloproteinase Activity in Osteoarthritis. Calcif Tissue Int 2021;109:277-90. [Crossref] [PubMed]
- Gendron C, Kashiwagi M, Lim NH, et al. Proteolytic activities of human ADAMTS-5: comparative studies with ADAMTS-4. J Biol Chem 2007;282:18294-306. [Crossref] [PubMed]
- Mitchell PG, Magna HA, Reeves LM, et al. Cloning, expression, and type II collagenolytic activity of matrix metalloproteinase-13 from human osteoarthritic cartilage. J Clin Invest 1996;97:761-8. [Crossref] [PubMed]
- Kevorkian L, Young DA, Darrah C, et al. Expression profiling of metalloproteinases and their inhibitors in cartilage. Arthritis Rheum 2004;50:131-41. [Crossref] [PubMed]
- Martin MU, Wesche H. Summary and comparison of the signaling mechanisms of the Toll/interleukin-1 receptor family. Biochim Biophys Acta 2002;1592:265-80. [Crossref] [PubMed]
- Kawai T, Akira S. TLR signaling. Semin Immunol 2007;19:24-32. [Crossref] [PubMed]
- Wojdasiewicz P, Poniatowski ŁA, Szukiewicz D. The role of inflammatory and anti-inflammatory cytokines in the pathogenesis of osteoarthritis. Mediators Inflamm 2014;2014:561459. [Crossref] [PubMed]
- Meszaros E, Malemud CJ. Prospects for treating osteoarthritis: enzyme-protein interactions regulating matrix metalloproteinase activity. Ther Adv Chronic Dis 2012;3:219-29. [Crossref] [PubMed]
- Koshy PJ, Lundy CJ, Rowan AD, et al. The modulation of matrix metalloproteinase and ADAM gene expression in human chondrocytes by interleukin-1 and oncostatin M: a time-course study using real-time quantitative reverse transcription-polymerase chain reaction. Arthritis Rheum 2002;46:961-7. [Crossref] [PubMed]
- Chenoufi HL, Diamant M, Rieneck K, et al. Increased mRNA expression and protein secretion of interleukin-6 in primary human osteoblasts differentiated in vitro from rheumatoid and osteoarthritic bone. J Cell Biochem 2001;81:666-78. [Crossref] [PubMed]
- Sakao K, Takahashi KA, Arai Y, et al. Osteoblasts derived from osteophytes produce interleukin-6, interleukin-8, and matrix metalloproteinase-13 in osteoarthritis. J Bone Miner Metab 2009;27:412-23. [Crossref] [PubMed]
- Dai SM, Shan ZZ, Nishioka K, et al. Implication of interleukin 18 in production of matrix metalloproteinases in articular chondrocytes in arthritis: direct effect on chondrocytes may not be pivotal. Ann Rheum Dis 2005;64:735-42. [Crossref] [PubMed]
- Inoue H, Hiraoka K, Hoshino T, et al. High levels of serum IL-18 promote cartilage loss through suppression of aggrecan synthesis. Bone 2008;42:1102-10. [Crossref] [PubMed]