Umbilical cord mesenchymal stem cells alleviate Sjögren’s syndrome and related pulmonary inflammation through regulating Vγ4+ IL-17+ T cells
Introduction
Primary Sjögren’s syndrome (SS) is a typical autoimmune disease characterized by progressive inflammation, tissue damage in salivary glands (SGs) and lacrimal glands (LGs), and reduced production of saliva and tears which may lead to symptoms like dry mouth and dry eye (1-3). It also impairs some organs, including those of the renal, respiratory, and nervous systems. Among them, SS-related complications in the lung are frequent, for example, pulmonary inflammation affects about 10–60% of SS patients (4,5). Moreover, SS-related pulmonary inflammation is a critical factor leading to poor prognosis in SS patients, with a 5-year cumulative mortality rate of up to 16% (6,7).
Gamma delta (γδ) T cells are minor populations of T lymphocytes rearranged with γ- and δ-T cell receptors (TCR) (8) which expressed on the surface of T cells (9). As a type of innate immune cells (10), γδ T cells rapidly recognize exogenous pathogens and endogenous stress-induced ligands in a major histocompatibility complex (MHC)-unrestricted manner and trigger adaptive immunity as the first line of immune defense (11). The γδ T cells are also involved in immune surveillance and immune defense against tumors and infection. Like αβ T cells, γδ T cells also generate type 1 T helper (Th1)-, Th2-, Th17-, and Treg-like phenotypes, and play an essential role in inflammation and immune tolerance. According to the properties of different regions in γ-chain and δ-chain, human γδ T cells are divided into Vδ1, Vδ2, and Vδ3 subsets. Murine γδ T cells have Vγ1, Vγ3, Vγ4, Vγ6, and Vγ7 subsets (Vγ2 cells distribute in the spleen, liver, and lung) (12,13), among which Vγ7 subsets only distribute in the intestines (12). Some studies have focused on interferon (IFN)-γ-secreting γδ T cells (IFN-γ+ γδ T cells) and interleukin (IL)-17A-secreting γδ T cells (IL-17A+ γδ T cells) (9,14), and it has been shown that these subsets of γδ T cells play critical roles in autoimmune diseases. The numbers of Vγ1+ T cells and Vγ4+ T cells have been shown to increase in collagen-induced arthritis (CIA) mice, and their CIA was exacerbated by IL-17A produced by the activated Vγ4+ T cells (15). It has been shown that Vγ4+ T cells and Vγ6+ T cells are involved in the development of psoriasis (16-18). In addition, studies have shown that γδ T cells play essential roles in myeloperoxidase (MPO)-induced glomerulonephritis and crescentic glomerulonephritis as well as SS patients (19,20). However, it is still largely unknown whether γδ T cells and their subsets participate in the pathogenesis of SS and SS-related pulmonary inflammation.
Mesenchymal stem cells (MSCs), a strain of multipotent progenitor cells, can differentiate into different cell lineages including osteoblasts, chondrocytes and adipocytes. Their paracrine action and ability to interact with different immune cells endow MSCs with a potential of multilineage differentiation and immunosuppression. The MSCs also display a wide range of immunomodulatory properties (21). MSCs transplantation (MSCT) displayed therapeutic effects on various autoimmune diseases, including systemic lupus erythematosus (22,23), SS (24), and rheumatoid arthritis (25,26). Our previous study showed that allogeneic MSCs prevented and suppressed SS-like symptoms in non-obese diabetic (NOD) mice (27). Our previous study also indicated that MSCT alleviated SS-like symptoms in NOD mice through reducing the aberrant accumulation and improving the suppressive function of myeloid-derived suppressor cells (28). In summary, the previous animal and human studies have demonstrated that MSC transplantation reestablishes salivary function and reduces lymphocytic infiltration in SG. However, whether MSCs have therapeutic effects in SS-related pulmonary inflammation remains to be determined. This study aimed to investigate the effects of MSCs on SS-related pulmonary inflammation and investigated the underlying mechanisms of MSC treatment in a mouse model. We present the following article in accordance with the ARRIVE reporting checklist (available at https://atm.amegroups.com/article/view/10.21037/atm-22-1855/rc).
Methods
Animals
A total of 100 female NOD/Ltj mice and 20 outbred strain (a subline of NOD/Ltj strain) Institute of Cancer Research (ICR) mice were provided by Beijing HFK Bioscience Co., Ltd. (Beijing, China) and maintained in a pathogen-free animal facility with free access to water and food. A protocol was prepared before the study without registration. Animal experiments were approved by the Animal Care and Use Committee of Nanjing Drum Tower Hospital, Clinical College of Traditional Chinese and Western Medicine, Nanjing University of Chinese Medicine (Approval No. 20180401), in compliance with Chinese national guidelines for the care and use of animals. In order to reduce pain, suffering and distress, 3R principle was observed to conduct the experiments.
Saliva flow rate measurement
After anesthetizing the mice, the saliva secretion was induced by intraperitoneal injection of pilocarpine (Sigma Aldrich, St. Louis, MO, USA) at a dose of 0.1 mg/kg (body weight). Stimulated whole saliva was collected using a 200 µL pipette tip within 15 minutes after injection.
Histology of SG and lung
The SG and lungs were collected and fixed with 4% paraformaldehyde (PFA) for 24 hours. Then the tissues were embedded in paraffin, cut into 3-µm-thick sections, and stained with hematoxylin and eosin (H&E). The sections were photographed by a light microscope (Olympus FSX100; Olympus Corp., Tokyo, Japan). The number of inflammatory foci (containing 50 lymphocytes in every 4 mm2 of tissue) was calculated in each field at ×200 magnification by Image-Pro Plus Version 6.0 software (Media Cybernetics, Silver Springs, MD, USA). For each gland, 10 fields in each section were observed by an experienced expert of histopathology in a blinded fashion.
Isolation of lymphocytes from peripheral blood, spleen, and lung
Peripheral blood was centrifuged at 400 ×g for 5 minutes. The plasma was collected and stored at -80 °C. Phosphate-buffered saline (PBS) was added to the remaining blood cells. The mixture was loaded onto the same volume of lymphocyte separation medium for mice. Centrifugation was performed at 715 ×g for 20 minutes in brake off mode. The lymphocytes were collected and washed twice with PBS. The spleens were ground, and the splenic slurry was filtered through a 200-mesh sieve. The cell suspension was centrifuged at 400 ×g for 5 minutes. Then, the cell pellets were resuspended with red cell lysis buffer and washed with PBS. The lungs were cut up and digested with type II collagenase under 37 °C for 45 minutes. Then, they were ground and filtered through a 200-mesh sieve. The cell suspension was centrifuged at 400 ×g for 5 minutes. The cell pellets were lysed with red cell lysis buffer and washed twice with PBS.
Adoptive transfer of γδ T cells
To identify the role of γδ T cells in the development of SS, we transferred γδ T cells from control mice into NOD/Ltj mice. Purification of γδ T cells was performed using a CD3 enrichment assay kit (Miltenyi Biotec, Bergisch Gladbach, Germany) and BD FACSAria [Becton, Dickinson, and Co. (BD), San Jose, CA, USA]. We randomly allocated 20 mice to the 2 groups using a random number method. Purified γδ T cells (1×105 cells/mouse) were intravenously transferred into NOD/Ltj mice via the tail vein. The control group mice were injected with an equal volume of PBS.
MSC transplantation
Fresh human umbilical cords were obtained from Nanjing Drum Tower Hospital (Nanjing, China). The MSCs were prepared as described previously (29). A total of 30 mice were randomly allocated to the 3 groups using a random number method. For MSCT, the 8-week NOD/Ltj mice were injected with MSCs (5×105 cells/mouse) in 0.15 mL PBS via the tail vein. The dose and site of MSCs were chosen according to our previous studies (27-29). The PBS treated and untreated NOD/Ltj mice served as controls. The study was conducted in accordance with the Declaration of Helsinki (as revised in 2013). The study was approved by institutional ethics committee board of Nanjing Drum Tower Hospital, Clinical College of Traditional Chinese and Western Medicine, Nanjing University of Chinese Medicine (Approval No. SC201600202) and informed consent was taken from all the patients.
Flow cytometric analysis
The cells derived from peripheral blood, spleen, and lung were stimulated with 100 µL of Roswell Park Memorial Institute (RPMI) 1640 (Hyclone, Logan, UT, USA) containing 10% fetal bovine serum (FBS) and 0.1 µg/mL phorbol myristate acetate plus 1 µg/mL ionomycin in 5 µg/mL Brefeldin A for 4 hours in 5% CO2 at 37 °C. After incubation, the cells were collected and washed twice with 200 µL staining buffer (1× PBS containing 1% FBS) at 400 ×g for 5 minutes.
For surface staining, the cells were labeled with the following antibodies: Percp/Cy5.5 anti-mouse CD45 (BioLegend, San Diego, CA, USA), APC-eFluor®780 anti-CD3e-mouse (eBioscience, San Diego, CA, USA), PE/Cy7 anti-mouse TCR γ/δ (BioLegend), BV421 anti-mouse TCR Vγ1.1 (BD Pharmingen, San Diego, CA, USA), APC anti-mouse TCR Vγ2 (BioLegend), and BV711 anti-mouse TCR Vγ3 (BD Pharmingen). For intracellular cytokines staining, the cells were fixed and permeabilized with BD Fixation/Permeabilization Kit, followed by staining with FITC anti-mouse IFN-γ (eBioscience) and PE anti-mouse IL-17A (eBioscience). Data were acquired on a BD FACSAria™ III (BD Biosciences, USA) and analyzed with FlowJo software (Tree Star, Ashland, OR, USA).
Statistical analysis
All normally distributed data were presented as mean ± standard error of the mean (SEM). Differences between 2 groups were analyzed using the Student’s t-test. Comparison between multiple groups was performed using one-way analysis of variance (ANOVA) test followed by post hoc test [least significant difference (LSD)]. A P value <0.05 was regarded statistically significant and was adjusted by the Bonferroni method to allow for multiple comparisons. Charts were made with the software GraphPad Prism 5.0 (GraphPad Inc., La Jolla, CA, USA).
Results
NOD mice displayed SS-like symptoms
Firstly, we evaluated the SS-like symptoms between 8-week-old NOD/Ltj mice (n=10) and ICR mice (n=10). Compared with ICR mice, NOD/Ltj mice developed the hallmark symptoms of SS, including larger and heavier salivary glands (Figure 1A,1B) and less saliva flow rate (Figure 1C). In addition, the weight coefficient of LG in NOD/Ltj mice (Figure 1D), and decreased of spleen (Figure 1E), but cervical lymph nodes did not show any differences in NOD/Ltj mice (Figure 1F). Notably, prominent lymphocytic infiltration was found in submandibular glands (Figure 1G) and LGs (Figure 1H). No lymphocytic infiltration was detected in kidneys and livers (Figure S1A,S1B).
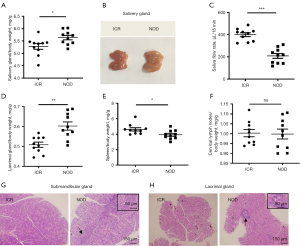
γδ T cells increased in lung of NOD mice
To verify the presence of lymphocytic infiltration in the lung, the lung tissues of NOD/Ltj mice were histologically analyzed at 6, 8, and 12 weeks, respectively (n=10 at each time point, respectively) (Figure 2A). Compared with the number of lymphocytes at 6 weeks, lymphocytic infiltration became more prominent at 8 weeks, and multiple lymphocytic foci and extensive acinar destruction were detected at 12 weeks. The proportion and number of γδ T cells in the lung increased at 8 and 12 weeks, compared with those at 6 weeks (Figure 2B). However, compared with the NOD/Ltj mice at 6 weeks, the levels of γδ T cells in the peripheral blood decreased at 8 and 12 weeks (Figure 2C). Taken together, γδ T cells increased in lung but decreased in the peripheral blood of NOD/Ltj mice at 8 and 12 weeks.
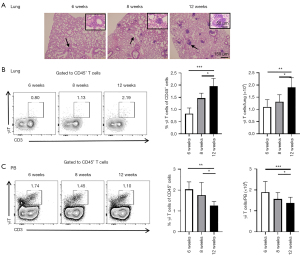
γδ T cell subsets in NOD mice
We detected Vγ4, Vγ3, and double negative subsets using anti-TCR Vγ2 and anti-TCR Vγ3 antibodies according to a previously published staining protocol about NOD/Ltj mice (n=10) and ICR mice (n=10) (30). We then used an anti-TCR Vγ1.1 antibody to determine the Vγ1 and Vγ6 subsets of NOD/Ltj mice (n=10) and ICR mice (n=10). In peripheral blood, the frequency of γδ T cell populations, including Vγ4+ T cells and Vγ4+ IL-17A+ T cells, was significantly reduced in NOD/Ltj mice compared with ICR mice (Figure 3A,3B). Furthermore, in the lung, the frequency of γδ T cells increased, as manifested by increased proportions and numbers of IL-17A+ γδ T cells, Vγ4+ T cells and Vγ4+ IL-17A+ T cells (Figure 3C,3D). However, IFN-γ+ γδ T cells showed no difference between NOD/Ltj mice and ICR mice (Figure S2A,S2B). The Vγ6+ T cells (Vγ6+ T cells are represented by Vγ− T cells) did not show any significant differences in the spleen (Figure S3A,S3B), as did Vγ1+ T cells (Figure S3A,S3C). The Vγ6+ T cells did not show any significant differences in peripheral blood (Figure S4A,S4B), as did Vγ1+ T cells (Figure S4A,S4C). The Vγ6+ T cells showed no obvious changes in the lungs (Figure S5A,S5B), as did Vγ1+ T cells (Figure S5A,S5C). Interestingly, almost no Vγ3+ T cells were detected in the lung. Altogether, our data suggested that γδ T cell subsets might play a role in SS related lung injury of NOD/Ltj mice.
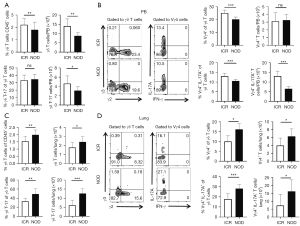
γδ T cells aggravated SS-related pulmonary inflammation
To further identify the role of γδ T cells and their subsets in the pathogenesis of SS-related pulmonary inflammation, the SS mice were adoptively transferred with γδ T cells (n=10) and PBS (n=10) respectively. At 5 weeks after adoptive transfer of purified γδ T cells, NOD/Ltj mice showed markedly reduced saliva flow rates and increased SG weight/body weight (Figure 4A). Histological examination showed that periductal foci of lymphocytic cells had infiltrated into the lung in γδ T cells-transferred NOD/Ltj mice (Figure 4B). Compared with the control NOD/Ltj mice injected with PBS, the proportions of Vγ4+ T cells and the proportions and numbers of Vγ4+ IL-17A+ T cells significantly increased in the lung, and also increased in peripheral blood (Figure 4C,4D), indicating that SS-related features and pulmonary inflammation in NOD/Ltj mice were at least partially contributed by the infiltration of Vγ4+ IL-17A+ T cells in the lung.
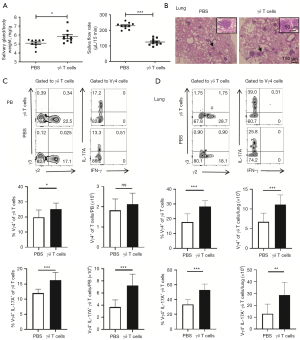
MSCs alleviated SS-like symptoms and reduced γδ T subsets in lung of NOD/Ltj mice
To verify the role and the underlying mechanisms of MSCs on SS-like symptoms. MSCs were transplanted in NOD/Ltj mice. The MSC-treated (n=10), PBS (n=10) and untreated (n=10) NOD/Ltj mice were further investigated. It was revealed that the severity of lymphocytes infiltration in the SG and lung were significantly reduced at 12 weeks after MSCs therapy (Figure 5A,5B), as evidenced by the decreased gland weight and elevated saliva flow rate (Figure 5C). Furthermore, the proportions and numbers of Vγ4+ T cells and Vγ4+ IL-17A+ T cells in the spleen significantly decreased after MSCs therapy (Figure 5D), and the numbers of Vγ4+ T cells and the proportions and numbers of Vγ4+ IL-17A+ T cells in the lung significantly decreased after MSCs therapy (Figure 5E). Together, our data suggested that MSCs alleviated SS-like symptoms, which might through reducing infiltration of Vγ4+ IL-17A+ T cells in lung of NOD/Ltj mice.
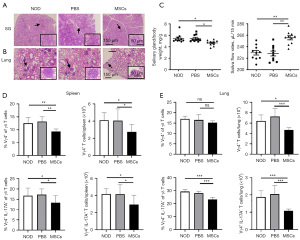
Discussion
Patients with SS may have a broad spectrum of pulmonary manifestations, including airways diseases (bronchiectasis, obstructive airways disease) and ILD. Increasingly, clinical studies have attempted to reveal the characteristics of primary (p)SS-related pulmonary inflammation (31,32). A previous study has indicated that abnormal serum nerve growth factor (NGF) and brain-derived neurotrophic factor (BDNF) may contribute to pSS-related pulmonary inflammation (33). Meanwhile, other studies report that serum IL-33 (34) and galectin-3 are involved in the pathogenesis of pSS-related pulmonary inflammation (35). In addition, a recent study has suggested that lymphoproliferative activity is active in the lungs of patients with pSS (36).
The γδ T cells can produce a wide range of antigens to transfer and stimulate other immune cells. A variety of immunomodulatory cytokines derived from γδ T cells are involved in disease, such as tumor-induced inflammation [IFN-γ, IL-6, and granulocyte-macrophage colony-stimulating factor (GM-CSF)], autoimmunity (IFN-γ and IL-17A), and allergy and asthma (IL-4 and IL-13). In addition, they can also produce immunosuppressive cytokines to down-regulate innate and adaptive immune cells, such as transforming growth factor-β (TGF-β) and IL-10 (12). However, until now there has been no evidence for the involvement of γδ T cells in pSS. In this study, γδ T cells, particularly Vγ4+ T cells, showed a critical role in pSS-related pulmonary inflammation. The lungs and spleens of NOD/Ltj mice were found to have increased γδ T cells and their subsets (Vγ4+ IL-17A+ T cells). The adoptive transfer of γδ T cells exaggerated symptoms of pSS, including pSS-related pulmonary inflammation. The Vγ4+ T cells are closely associated with lung injury in several immune diseases. For example, Costa et al. found that Vγ4+ T cells were abnormally increased in the lungs of mice with severe sepsis (37). Furthermore, IL-17A serves as an important effector of Vγ4+ T cells. It was reported that lung Vγ4+ T cells mediated influenza A (H1N1) pdm09-induced immunopathological injury through secreting IL-17A (8). In addition, Vγ4+ T cells are the major IL-17-producing subsets of γδ T cells at the early period of Chlamydial muridarum lung infection (38). Other subsets of γδ T cells, such as IFN-γ+ γδ T cells, Vγ1+ T cells, and Vγ6+ T cells, may play weak roles in pSS and pSS-related pulmonary inflammation, because their levels almost remained unchanged in this study. However, the mechanism of the chemotaxis of Vγ4+ T cells from peripheral blood to lung needs further investigation.
The MSCs possess potent immunomodulatory functions and regulate both adaptive and innate immune responses. Therefore, MSCs have potential to treat pSS (38) and related diseases, like pSS-related pulmonary inflammation. Clinically, our previous paper observes that SS disease activity score is greatly reduced after MSCs therapy in SS patients (27). In this study, γδ T cells and their subsets decreased in the peripheral blood, while their numbers increased in the lung and the spleen, indicating that Vγ4+ T cells accumulating in the lung or spleen originated from the peripheral blood. Moreover, the level of Vγ4+ IL-17A+ T cells decreased in the lung and the spleen after MSCT. The therapeutic effects of MSCs might be attributed to immune-regulatory activity, which could activate CD4 T cells, promote Treg and Th2 development, and inhibit Th17 and T follicular helper (Tfh) inflammatory responses (39). The MSCs have a direct influence on γδ T cells, which could inhibit IFN-γ production by activating Vα24+ Vβ11+ and impairing CD3-mediated proliferation of Vα24+ Vβ11+ and Vβ2+ T cells, but without affecting their cytotoxic potential (40). Moreover, a cross-talk has been detected between MSCs and Vγ9Vδ2 T cells (41). Meanwhile, previous studies indicate MSCs may regulate TGF-β1 and IL-17A to regulate γδ T cells (42,43). Further studies are needed to reveal the mechanism on the effects of MSCT on γδ T cells. In our opinion, the biggest advantage of MSCs for SS treatment is that it provides an optional treatment for refractory cases. The biggest disadvantage is that the side effects to MSC transplantation. The most difficult problem for its application in clinical filed is to identify the suitable patients that would benefit for MSC translation more than traditional therapies, and to avoid the side effects to MSC transplantation.
In the present study, we found that γδ T cells and their subsets accumulated in the lung and the spleen. The Vγ4+ T cells that secrete IL-17A aggravated SS-related pulmonary inflammation. Moreover, MSCT could reduce the accumulation of γδ T cells, which was accompanied by amelioration of the symptoms of SS and SS-related pulmonary inflammation.
In general, this study discloses that peripheral Vγ4+ T cells infiltrate into the lung or the spleen in SS mice, and aggravate the symptoms of SS and SS-related pulmonary inflammation by secreting IL-17A. Lymphocyte infiltration could be reversed by MSCT, which signals the potential of MSCs in the treatment of SS and SS-related pulmonary inflammation patients.
Acknowledgments
Funding: The study was supported by grants from the Major International (Regional) Joint Research Project (81720108020), the National Natural Science Foundation of China (NSFC) (81970062, 81770061, and 81571583), Jiangsu Province Major Research and Development Program (BE2020621), and Jiangsu Province 333 Talent Grant (BRA2016001).
Footnote
Reporting Checklist: The authors have completed the ARRIVE reporting checklist. Available at https://atm.amegroups.com/article/view/10.21037/atm-22-1855/rc
Data Sharing Statement: Available at https://atm.amegroups.com/article/view/10.21037/atm-22-1855/dss
Conflicts of Interest: All authors have completed the ICMJE uniform disclosure form (available at https://atm.amegroups.com/article/view/10.21037/atm-22-1855/coif). The authors have no conflicts of interest to declare.
Ethical Statement: The authors are accountable for all aspects of the work in ensuring that questions related to the accuracy or integrity of any part of the work are appropriately investigated and resolved. The study was also conducted in accordance with the Declaration of Helsinki (as revised in 2013). The study was approved by institutional ethics committee board of Nanjing Drum Tower Hospital, Clinical College of Traditional Chinese and Western Medicine, Nanjing University of Chinese Medicine (Approval No. SC201600202) and informed consent was taken from all the patients. Animal experiments were approved by the Animal Care and Use Committee of Nanjing Drum Tower Hospital, Clinical College of Traditional Chinese and Western Medicine, Nanjing University of Chinese Medicine (Approval No. 20180401), in compliance with Chinese national guidelines for the care and use of animals. In order to reduce pain, suffering and distress, 3R principle was observed to conduct the experiments.
Open Access Statement: This is an Open Access article distributed in accordance with the Creative Commons Attribution-NonCommercial-NoDerivs 4.0 International License (CC BY-NC-ND 4.0), which permits the non-commercial replication and distribution of the article with the strict proviso that no changes or edits are made and the original work is properly cited (including links to both the formal publication through the relevant DOI and the license). See: https://creativecommons.org/licenses/by-nc-nd/4.0/.
References
- Negrini S, Emmi G, Greco M, et al. Sjögren's syndrome: a systemic autoimmune disease. Clin Exp Med 2022;22:9-25. [Crossref] [PubMed]
- Fox RI, Fox CM, Gottenberg JE, et al. Treatment of Sjögren's syndrome: current therapy and future directions. Rheumatology (Oxford) 2021;60:2066-74. [Crossref] [PubMed]
- Jonsson R. Disease mechanisms in Sjögren's syndrome: What do we know? Scand J Immunol 2022;95:e13145. [Crossref] [PubMed]
- Roca F, Dominique S, Schmidt J, et al. Interstitial lung disease in primary Sjögren's syndrome. Autoimmun Rev 2017;16:48-54. [Crossref] [PubMed]
- Mestre-Torres J, Solans-Laque R. Pulmonary involvement in Sjögren's syndrome. Med Clin (Barc) 2022;158:181-5. [Crossref] [PubMed]
- Ramos CG, Sousa SA, Grilo AM, et al. The second RNA chaperone, Hfq2, is also required for survival under stress and full virulence of Burkholderia cenocepacia J2315. J Bacteriol 2014;196:3980. [Crossref] [PubMed]
- Lee CT, Strek ME. The other connective tissue disease-associated interstitial lung diseases: Sjogren's syndrome, mixed connective tissue disease, and systemic lupus erythematosus. Curr Opin Pulm Med 2021;27:388-95. [Crossref] [PubMed]
- Xue C, Wen M, Bao L, et al. Vγ4+γδT Cells Aggravate Severe H1N1 Influenza Virus Infection-Induced Acute Pulmonary Immunopathological Injury via Secreting Interleukin-17A. Front Immunol 2017;8:1054. [Crossref] [PubMed]
- Paul S, Singh AK. Phenotypic and functional plasticity of gamma-delta (γδ) T cells in inflammation and tolerance. Int Rev Immunol 2014;33:537-58. [Crossref] [PubMed]
- Li X, Kang N, Zhang X, et al. Generation of human regulatory gammadelta T cells by TCR gammadelta stimulation in the presence of TGF-beta and their involvement in the pathogenesis of systemic lupus erythematosus. J Immunol 2011;186:6693-700. [Crossref] [PubMed]
- Wu D, Wu P, Qiu F, et al. Human γδ T-cell subsets and their involvement in tumor immunity. Cell Mol Immunol 2017;14:245-53. [Crossref] [PubMed]
- Bonneville M, O'Brien RL, Born WK. Gammadelta T cell effector functions: a blend of innate programming and acquired plasticity. Nat Rev Immunol 2010;10:467-78. [Crossref] [PubMed]
- Papotto PH, Ribot JC, Silva-Santos B. IL-17+ γδ T cells as kick-starters of inflammation. Nat Immunol 2017;18:604-11. [Crossref] [PubMed]
- Prinz I, Silva-Santos B, Pennington DJ. Functional development of γδ T cells. Eur J Immunol 2013;43:1988-94. [Crossref] [PubMed]
- Roark CL, French JD, Taylor MA, et al. Exacerbation of collagen-induced arthritis by oligoclonal, IL-17-producing gamma delta T cells. J Immunol 2007;179:5576-83. [Crossref] [PubMed]
- Cai Y, Shen X, Ding C, et al. Pivotal role of dermal IL-17-producing γδ T cells in skin inflammation. Immunity 2011;35:596-610. [Crossref] [PubMed]
- Cai Y, Xue F, Fleming C, et al. Differential developmental requirement and peripheral regulation for dermal Vγ4 and Vγ6T17 cells in health and inflammation. Nat Commun 2014;5:3986. [Crossref] [PubMed]
- Ramírez-Valle F, Gray EE, Cyster JG. Inflammation induces dermal Vγ4+ γδ T17 memory-like cells that travel to distant skin and accelerate secondary IL-17-driven responses. Proc Natl Acad Sci U S A 2015;112:8046-51. [Crossref] [PubMed]
- Gan PY, Fujita T, Ooi JD, et al. Pathogenic role for γδ T cells in autoimmune anti-myeloperoxidase glomerulonephritis. J Immunol 2017;199:3042-50. [Crossref] [PubMed]
- Gerli R, Agea E, Muscat C, et al. Functional characterization of T cells bearing the gamma/delta T-cell receptor in patients with primary Sjögren's syndrome. Clin Exp Rheumatol 1993;11:295-9. [PubMed]
- Harrell CR, Djonov V, Volarevic V. The cross-talk between mesenchymal stem cells and immune cells in tissue repair and regeneration. Int J Mol Sci 2021;22:2472. [Crossref] [PubMed]
- Liang J, Zhang H, Hua B, et al. Allogenic mesenchymal stem cells transplantation in refractory systemic lupus erythematosus: a pilot clinical study. Ann Rheum Dis 2010;69:1423-9. [Crossref] [PubMed]
- Li A, Guo F, Pan Q, et al. Mesenchymal stem cell therapy: hope for patients with systemic lupus erythematosus. Front Immunol 2021;12:728190. [Crossref] [PubMed]
- Chihaby N, Orliaguet M, Le Pottier L, et al. Treatment of Sjögren's syndrome with mesenchymal stem cells: A systematic review. Int J Mol Sci 2021;22:10474. [Crossref] [PubMed]
- Sarsenova M, Issabekova A, Abisheva S, et al. Mesenchymal stem cell-based therapy for rheumatoid arthritis. Int J Mol Sci 2021;22:11592. [Crossref] [PubMed]
- Hwang JJ, Rim YA, Nam Y, et al. Recent developments in clinical applications of mesenchymal stem cells in the treatment of rheumatoid arthritis and osteoarthritis. Front Immunol 2021;12:631291. [Crossref] [PubMed]
- Xu J, Wang D, Liu D, et al. Allogeneic mesenchymal stem cell treatment alleviates experimental and clinical Sjögren syndrome. Blood 2012;120:3142-51. [Crossref] [PubMed]
- Yao G, Qi J, Liang J, et al. Mesenchymal stem cell transplantation alleviates experimental Sjögren's syndrome through IFN-β/IL-27 signaling axis. Theranostics 2019;9:8253-65. [Crossref] [PubMed]
- Sun L, Wang D, Liang J, et al. Umbilical cord mesenchymal stem cell transplantation in severe and refractory systemic lupus erythematosus. Arthritis Rheum 2010;62:2467-75. [Crossref] [PubMed]
- Kulig P, Musiol S, Freiberger SN, et al. IL-12 protects from psoriasiform skin inflammation. Nat Commun 2016;7:13466. [Crossref] [PubMed]
- Enomoto Y, Takemura T, Hagiwara E, et al. Prognostic factors in interstitial lung disease associated with primary Sjögren's syndrome: a retrospective analysis of 33 pathologically-proven cases. PLoS One 2013;8:e73774. [Crossref] [PubMed]
- Dong X, Zhou J, Guo X, et al. A retrospective analysis of distinguishing features of chest HRCT and clinical manifestation in primary Sjögren's syndrome-related interstitial lung disease in a Chinese population. Clin Rheumatol 2018;37:2981-8. [Crossref] [PubMed]
- Li YJ, Yang CS, Lei L, et al. Serum nerve grow factor and brain-derived neurotrophic factor profiles in Sjögren's syndrome concomitant with interstitial lung disease. Clin Rheumatol 2014;33:1161-4. [Crossref] [PubMed]
- Zhao L, Yao L, Yuan L, et al. Potential contribution of interleukin-33 to the development of interstitial lung disease in patients with primary Sjogren's Syndrome. Cytokine 2013;64:22-4. [Crossref] [PubMed]
- Zhang R, Sun T, Song L, et al. Increased levels of serum galectin-3 in patients with primary Sjögren's syndrome: associated with interstitial lung disease. Cytokine 2014;69:289-93. [Crossref] [PubMed]
- Kakugawa T, Sakamoto N, Ishimoto H, et al. Lymphocytic focus score is positively related to airway and interstitial lung diseases in primary Sjögren's syndrome. Respir Med 2018;137:95-102. [Crossref] [PubMed]
- Costa MF, de Negreiros CB, Bornstein VU, et al. Murine IL-17+ Vγ4 T lymphocytes accumulate in the lungs and play a protective role during severe sepsis. BMC Immunol 2015;16:36. [Crossref] [PubMed]
- Sun LD, Qiao S, Wang Y, et al. Vγ4+ T cells: A novel IL-17-producing γδ T subsets during the early phase of chlamydial airway infection in mice. Mediators Inflamm 2018;2018:6265746. [Crossref] [PubMed]
- Prigione I, Benvenuto F, Bocca P, et al. Reciprocal interactions between human mesenchymal stem cells and gammadelta T cells or invariant natural killer T cells. Stem Cells 2009;27:693-702. [Crossref] [PubMed]
- Chen W, Yu Y, Ma J, et al. Mesenchymal stem cells in primary Sjögren's syndrome: prospective and challenges. Stem Cells Int 2018;2018:4357865. [Crossref] [PubMed]
- Martinet L, Fleury-Cappellesso S, Gadelorge M, et al. A regulatory cross-talk between Vgamma9Vdelta2 T lymphocytes and mesenchymal stem cells. Eur J Immunol 2009;39:752-62. [Crossref] [PubMed]
- Chen Y, Hu Y, Zhou X, et al. Human umbilical cord-derived mesenchymal stem cells ameliorate psoriasis-like dermatitis by suppressing IL-17-producing γδ T cells. Cell Tissue Res 2022;388:549-63. [Crossref] [PubMed]
- Zou B, Zhuang RX, Sun XY, et al. Analysis of the expression changes of IL-17+ γδ T cells and treg cells in bone marrow mesenchymal stem cells targeted therapy for allergic rhinitis. Eur Rev Med Pharmacol Sci 2021;25:2858-65. [PubMed]
(English Language Editor: J. Jones)