ASPM and TROAP gene expression as potential malignant tumor markers
Introduction
As life expectancy increases, cancers are gradually surpassing cardiovascular diseases as a major risk factor for human health and the most common cause of death in China (1). However, there are still no effective strategies for cancer therapy (2). One convenient approach to find potential therapy targets is pancancer expression analysis of genes in public databases, such as TCGA and HPA, to evaluate their correlations with clinical prognosis and relevant signaling pathways in cancer (3-5).
Assembly factor for spindle microtubules (ASPM) is overexpressed in a variety of cancer cell lines, including colorectal cancer, breast cancer and gastric cancer (6-8). A previous study suggested that the ASPM-katanin complex regulated microtubule disassembly at spindle poles (9). Furthermore, downregulation of ASPM could arrest the cell cycle of glioblastoma multiforme (GBM) cells and attenuate Wnt/β-catenin signaling activity in GBM (10). ASPM serves as an oncogenic regulator of tumor cell proliferation and metastasis in lung carcinoma (7). However, the role of ASPM in tumors remains elusive.
Trophinin-associated protein (TROAP), also known as tastin, is involved in centrosome integrity and spindle assembly during mitosis (11). TROAP promotes tumorigenesis and predicts poor prognosis in prostate cancer, breast cancer and hepatocellular carcinoma (HCC) (12-14). However, TROAP was also reported to suppress cellular growth and migration in HCC (15), which indicated the intricate mechanism of TROAP in tumor progression. In general, the biological functions of TROAP in cancer remain to be elucidated.
Cell division cycle 20 (CDC20), also called Fizzy, is a modulator of the ubiquitin E3 ligase anaphase promoting complex/cyclosome (APC/C). APC/C is well known for its role in regulating the mitotic transition from metaphase to anaphase (16). Recent evidence indicates that CDC20 is overexpressed in various cancers, such as prostate cancer, glioblastoma, and breast cancer (17-19). Furthermore, a previous study has reported the regulators of CDC20. For example, p53 inhibits tumor cell growth through the indirect regulation of CDC20 (20). USP44 (ubiquitin-specific protease 44) deubiquitinates CDC20 and blocks premature activation of APC by stabilizing the APC-inhibitory Mad2-CDC20 complex (21). CDC20 also promotes cell proliferation and invasion by regulating the Wnt/β-catenin signaling pathway in prostate cancer and cutaneous squamous cell carcinoma (22,23). In summary, increasing evidence suggests that CDC20 is an emerging oncogenic protein and that its expression can impact tumor patient prognosis.
In the present study, we reported that ASPM and TROAP were highly expressed in most cancers and predicted a poor prognosis among tumor patients. Meanwhile, these 2 genes presented a strict positive correlation. Mechanistically, ASPM and TROAP both regulated cell replication in the S&G2 phase of the cell cycle. As CDC20 is involved in the regulation of the cell cycle, we identified a new upstream regulator and downstream substrate of CDC20, TROAP and ASPM. Thus, TROAP regulates the role of ASPM in cancers, and ASPM is likely associated with cancer cell proliferation. Therefore, targeting ASPM may be a promising tumor therapeutic strategy for cancer patients. We present the following article in accordance with the STREGA reporting checklist (available at https://atm.amegroups.com/article/view/10.21037/atm-22-1112/rc).
Methods
Data processing and differential expression analysis
Raw data were downloaded from TCGA (containing 11,069 samples from 33 types of cancer). The downloaded data and expression levels were compared between cancer samples and matched standard samples in 31 cancers. Expression data were log2-transformed, and two sets of t tests were conducted on these tumor types; P<0.05 was considered to indicate differential expression between tumor and normal tissues. Data analysis was conducted using R software (Version 4.0.2; https://www.Rproject.org), and the R package “ggpubr” was used to draw box plots. The study was conducted in accordance with the Declaration of Helsinki (as revised in 2013).
Differentially expressed genes (DEGs)
The limma package of the R language package was used to screen the DEGs between cancer samples and normal samples. Adjusted P<0.05 and |log2fold change (FC)| >1 were chosen as the cutoff criteria.
Comprehensive analysis of the PPI network
The Search Tool for the Retrieval of Interacting Genes Database (STRING) (https://cn.string-db.org/) was used to assess protein-protein interaction (PPI) information (24). In addition, to explore the relationship between DEGs, we used the STRING database and converted the results visually using Cytoscape software, a general-purpose, open-source software platform for network biology analysis and visualization (25). A confidence score >0.7 was set as significant.
Statistical analysis
All analyses were conducted three times and represented data from three separate experiments. Two-tailed Student’s t test was utilized to determine the significance of differences between subgroups. Statistical analysis was processed via SPSS 16.0. Statistical significance was set at probability values of P<0.05.
Results
ASPM and TROAP are relatively broad-spectrum malignant tumor markers
Prognostic factors are crucial indicators for tumor malignancy evaluation. We analyzed 31 cancer types and listed the top 10 genes in each cancer type related to poor prognosis based on the GEPIA results (Table S1) (http://gepia.cancer-pku.cn/). In Table 1, 16 genes that appeared more than once among the 31 cancer types were classified into upregulation (UP), no significant change (NS), and downregulation (DOWN) according to their expression levels. We found that ASPM and TROAP were significantly elevated in most cancer types (Figure S1, Figure 1A,1B), and the high expression of ASPM and TROAP led to a decreased life expectancy in cancer patients (Figure 1C,1D). High expression of both ASPM and TROAP appeared in 20/31 cancer types, while high expression of either ASPM or TROAP was represented in 5 more malignancies (64.52%) (Figure 1E). Therefore, ASPM and TROAP can be used as part of a broader spectrum of malignant tumor markers.
Table 1
No. | Gene symbol | UP | DOWN | NS |
---|---|---|---|---|
1 | TROAP | 23 | 1 | 7 |
2 | ASPM | 22 | 1 | 8 |
3 | TICRR | 9 | 1 | 20 |
4 | GJB4 | 8 | 1 | 22 |
5 | RAB27A | 4 | 4 | 23 |
6 | SYNDIG1 | 4 | 4 | 23 |
7 | NLGN3 | 2 | 6 | 23 |
8 | BCL2L14 | 6 | 1 | 24 |
9 | SIX2 | 5 | 1 | 25 |
10 | FBRSL1 | 5 | 0 | 26 |
11 | PCDH19 | 4 | 1 | 26 |
12 | CACNB4 | 1 | 3 | 27 |
13 | LA16c-329F2.2 | 0 | 1 | 30 |
14 | RP13-580B18.4 | 0 | 1 | 30 |
15 | RP11-295P9.8 | 0 | 0 | 31 |
16 | HSPA8P1 | 0 | 0 | 31 |
UP, upregulation; DOWN, downregulation; NS, no significant.
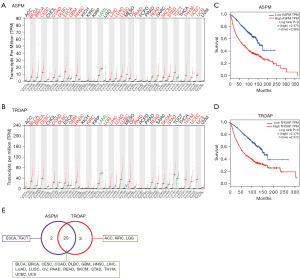
ASPM and TROAP are positively correlated in the tumor microenvironment
We analyzed the correlation between ASPM and TROAP in tumors. By measuring changes in their expression in various cancers, we found that these 2 genes often changed simultaneously (Figure 2A), suggesting that ASPM and TROAP play a role in specific types of cancer, thereby regulating tumorigenesis. We also analyzed the correlation between the expression levels of these genes. The results showed a high degree of identity (Figure 2B), with a statistical P value equal to 0. This suggests that, in most types of cancer, ASPM and TROAP show a strict positive correlation.
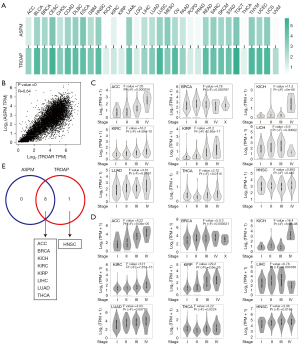
Based on these results, ASPM and TROAP can be used as indicators of malignant cancer, as they have a significant correlation with a decline in patient survival. In addition, the data showed that ASPM and TROAP have clear synchronicity in expression levels in multiple types of cancer. We analyzed the differences in the expression of ASPM and TROAP in different cancer stages and found that ASPM and TROAP is highly expressed in kidney chromophobe (KICH) with stage IV compared with other stages. Meanwhile, most tumors such as adrenocortical carcinoma (ACC) and kidney renal papillary cell carcinoma (KIRP) with stage III or stage IV presented relatively high ASPM and TROAP expression (Figure 2C,2D). This may be a cause of the significant reduction in survival rates of cancer patients. In addition, the synchronicity of expression between ASPM and TROAP was reflected in the significantly high expression of these genes in stage III and stage IV of cancer (F>2), with the exception of head and neck squamous cell carcinoma (HNSC) (Figure 2E). Therefore, ASPM and TROAP show a high degree of synchronization and may be related to the decline in survival rate in advanced stages of these cancers.
Clinicopathologic features of ASPM and TROAP
After analyzing the basic features of ASPM and TROAP using the GEPIA platform, we investigated the physiological characteristics of these genes. Because GEPIA is a resource for RNA data, the overall phenotypes of cancer significantly rely on protein expression. Therefore, we used the HPA database, which provides resources relating to the protein expression of genes under pathological conditions, such as cancer, to analyze the clinical and pathologic phenotypes of ASPM and TROAP (26-28).
We first analyzed the features of ASPM to identify its tissue-specific and cell-specific characteristics (Figure 3A,3B). The expression level of ASPM differed in various tissues, with high expression in bone marrow, lymph nodes, tonsils, testes, and other organs (Figure 3A). ASPM is also highly expressed in erythrocytes, spermatocytes, spermatogonia, extravillous trophoblasts, Hofbauer cells, cytotrophoblasts, basal keratinocytes, undifferentiated cells, T cells, Kupffer cells, B cells, late-stage sperm cells, and other immune or male reproduction-related cells (Figure 3B). This suggests that high expression of ASPM may be involved in immune regulation in the tumor microenvironment. In consistent with TROAP, the expression level of ASPM among tumors also presented low cancer specificity (Figure 3C). We used HPA data to analyze the poor prognosis of 4 specific cancer types related to ASPM: liver hepatocellular carcinoma (LIHC), pancreatic adenocarcinoma (PAAD), lung adenocarcinoma (LUAD), and uterine corpus endometrial carcinoma (UCEC). The results showed that the high expression of ASPM was indeed associated with poor prognosis in these 4 cancer types (Figure 3D). Moreover, the immunofluorescence staining results of 3 cell lines (A-431: human skin squamous cell carcinoma; U2-OS: human osteosarcoma cells; and U-251 MG: human glioma cells) showed that ASPM is mainly expressed in the cytoplasmic matrix and interacts with cytoplasmic matrix microtubules (Figure 3E).
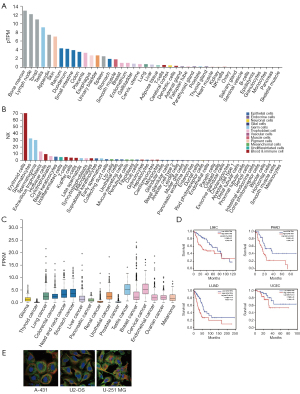
We used the same method of analysis to investigate TROAP. The data showed that TROAP and ASPM have similar tissue expression characteristics. That is, they are highly expressed in organs, such as testes, bone marrow, tonsils, and lymph nodes (Figure 4A). However, TROAP has its own cell-specific expression characteristics. TROAP is highly expressed in early sperm cells, late sperm cells, extravillous trophoblasts, spermatocytes, Hofbauer cells, spermatogonia, cytotrophoblasts, Ito cells, undifferentiated cells, basal keratinocytes, syncytiotrophoblast cells, Kupffer cells, T cells, mucus secreting cells, and other cells (Figure 4B). According to HPA data, the expression level of TROAP among tumors presented low cancer specificity (Figure 4C). Immunohistochemical staining showed discrepant protein levels of TROAP in liver cancer, lung cancer, breast cancer, prostate cancer, and colon cancer (Figure 4D). Therefore, based on protein levels, TROAP is closely related to the progression of malignant tumors. However, in terms of prognosis, the HPA database shows that TROAP leads to a poor prognosis only in LIHC (Figure 4E).
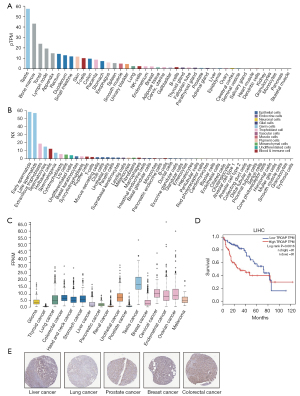
ASPM and TROAP regulate cell replication in the S&G2 phase
As the process of carcinogenesis is caused by an uncontrolled cell cycle, previously reported malignant cancer or tumor markers have been related to cell cycle regulation (29-31). The cell cycle is divided into 2 phases: interphase and division (32). Cancer cells also have a cell cycle, but their cycle regulation mechanism is destroyed (33), leading to uncontrolled cell growth and the transformation of normal cells into tumor cells. Using the GEPIA platform and the HPA database, we screened ASPM and TROAP as malignant tumor markers and analyzed their cell cycle changes.
We found that TROAP was more highly expressed than ASPM (Figure 5A,5B). The overall expression level of ASPM declined in the S transition (S-tr) period, but there was no significant difference between TROAP levels in the G1 and S-tr periods. ASPM and TROAP reached their highest expression levels in the S&G2 phase, but due to large variation within the group, the results were not significant. However, the S&G2 phase should still be considered the period when ASPM and TROAP play a role in cell cycle regulation. In the S&G2 phase, cell proliferation may become malignant due to an error in regulation, which eventually leads to cancer formation. Our results showed that ASPM and TROAP have very similar change profiles. Although the overall level of TROAP was still relatively high, there was a simultaneous rise and fall in the expression of both genes. This suggests that ASPM and TROAP may both regulate cell replication in the S&G2 phase of the cell cycle.
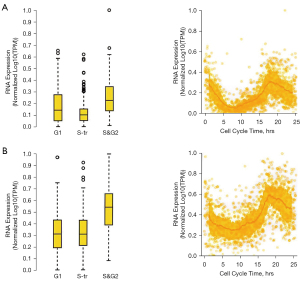
The interaction network of ASPM and TROAP
As ASPM and TROAP are highly correlated in a variety of cancers, we used the GCBI database to analyze the regulatory network of these 2 genes. The results showed that ASPM was mainly regulated by its interaction with proteins and less regulated by long non-coding RNA (lncRNA) and miRNA, while TROAP was mainly regulated by lncRNA (Figure 6A,6B). The regulatory factors shared by ASPM and TROAP were 2 miRNAs, namely hsa-miR-215-5p and hsa-miR-192-5p (Figure 6C). This finding suggests that these 2 miRNAs may be putative targets to weaken the functionality of ASPM and TROAP. In addition, we screened the potential TFs of ASPM and TROAP (Figure 6D,6E) and found that these genes may share 22 TFs (Figure 6F).
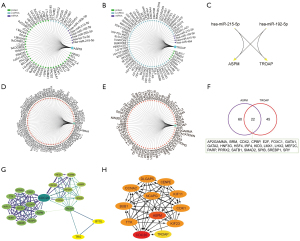
We performed a protein-protein interaction network (PPI) analysis of ASPM and TROAP (Figure 6G,6H). The results showed that ASPM is regulated by more factors than TROAP, which is consistent with previous reports (Figure 6A-6F). In addition, we found that CDC20 plays an important role in various regulatory factors. CDC20 is regulated by TROAP and is upstream of ASPM. Interestingly, TROAP itself can directly regulate ASPM; that is, ASPM is also a downstream factor of TROAP (Figure 6H). Although TROAP is upstream of regulatory factors, such as DNA topoisomerase II alpha (TOP2A) and sperm-associated antigen 5 (SPAG5), these regulatory factors regulated by TROAP depend on CDC20 for their function. Therefore, CDC20 may be a key regulator of the ASPM-TROAP protumor pathway, and targeting CDC20 may weaken the tumor promoting effect.
ASPM as a useful cancer marker based on previous clinical case studies
To determine whether TROAP and ASMP have been reported in previous cancer-related studies, we searched NCBI for bioinformatic data, including ASMP and TROAP. Because ASPM is a downstream factor of TROAP, we used ASPM as an example to study its role in different cancer subtypes.
Case 1: Aromatase inhibitors (AIs) play an important role in the treatment of breast cancer. However, the efficacy rate of adjuvant therapy, including AI, is only 50–70%, and the efficacy rate declines when treating advanced disease. Researchers need accurate biomarkers to predict treatment response and determine which individuals will benefit from assisted AI therapy. In this study, researchers recorded the transcriptome changes of the patients before treatment, 2 weeks after the commencement of treatment, and 3 months after the commencement of treatment. We used ASPM as the standard for analysis (GSE59515). The results showed that, after 2 weeks of treatment, the expression of ASPM decreased significantly, but after 3 months of treatment, the expression of ASPM showed an upward trend (Figure 7A). This suggests that AI as an adjuvant therapy is most effective after 2 weeks. If the medication is continued, there will be no more significant therapeutic effect. Although the curative effect of AI is limited, the expression of ASPM still showed a nonsignificant downward trend compared with that before the medication, indicating that, even with long-term medication, AI itself will not aggravate the cancer process.
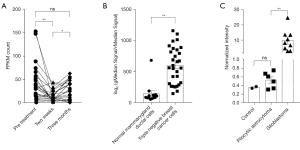
Case 2: Triple-negative breast cancer refers to cases in which the results of immunohistochemical examination of the cancer tissue are negative for estrogen receptor (ER), progesterone receptor (PR), and human epidermal growth factor receptor 2 (Her2) oncogene. This type of breast cancer accounts for 10.0% to 20.8% of all pathological types of breast cancer. It exhibits special biological behavior and clinicopathological characteristics and has a worse prognosis than other types. In this study, researchers extracted transcriptome samples of triple-negative breast cancer cells and normal breast duct cells. We analyzed the expression of ASPM in these cells (GSE38959). The results showed that the expression of ASPM in triple-negative breast cancer was significantly higher than that in normal breast duct cells (Figure 7B). This indicates that the high expression of ASPM is related to poor prognosis in this type of breast cancer.
Case 3: Tumor aggressiveness is one of the key indicators of malignancy. In this study, researchers extracted the transcriptome data of highly aggressive glioblastoma and typical benign pilocytic astrocytoma and used normal cells as a control. We used ASPM as a malignant marker (GSE7330), and our results showed that the expression level of ASPM in highly aggressive glioblastoma was significantly higher than that in benign pilocytic astrocytoma. The latter and normal cells both had similar ASPM expression levels (Figure 7C). Therefore, ASPM as an indicator of malignant tumors may be related to the aggressiveness of tumor cells.
Discussion
Malignant tumors are significant threats to human life. Accurate markers help us understand the occurrence and development of malignant cancer and provide targets for achieving precise treatment. In this study, we used the TCGA database, the HPA database, and the GEPIA platform to screen ASPM and TROAP, two potential markers of cancers. Our results showed that these genes demonstrate malignant tumor marker characteristics in terms of RNA level, prognostic analysis, pathological protein level, and cell cycle changes.
The TROAP gene is a member of the housekeeping gene family, which has been reported to play a role in various tumors and cancers (12-14,34). Our results indicate that it is valuable as a new malignant cancer marker. The ASPM gene is the human ortholog of the Drosophila “abnormal spindle” gene (asp), which is responsible for the function of the mitotic spindle in embryonic neuroblasts (35,36). Studies in mice have shown that this gene plays a role in the regulation of the mitotic spindle and has a preferential role in the regulation of neurogenesis (37,38). Mutations in this gene are associated with primary type 5 microcephaly. Multiple transcript variants of this gene encoding different isoforms have been found. Therefore, this gene regulates the cell proliferation cycle, and the disorder caused by its high expression may cause the cell to eventually become cancerous (39,40). A previous study reported ASPM’s cancer-promoting effects but did not confirm its status as an oncogene. Our results prove this to a certain extent.
Although tumor cells have the ability to proliferate malignantly, their replication process also conforms to basic cell cycle principles. The spindle is an important element in the regulation of the cell cycle in eukaryotes (41,42). There are two main functions of the spindle in the cell cycle. One is to arrange and divide chromosomes. The integrity of the spindle determines the accuracy of chromosome division as well as the time and space of the division process. In addition, as chromosome division occurs, parts of the microtubules in the spindle do not divide to the poles along with the chromosome but rest in the center to form a central spindle. In the center of the spindle, the midbody is where two sets of microtubules of opposite polarities overlap, called the spindle midzone. These structures all ensure that the cell can divide properly. In recent years, there have been many reports of abnormal spindles in cancer cells (43). In breast cancer, for example, spindle abnormalities serve as indicators of malignancy (44). Dysfunction of the spindle is often seen in malignant cancer cells, which theoretically cannot pass cell cycle checkpoints (45), but there are still unknown mechanisms that help the spindle enter the cell cycle through checkpoints. Our results show that ASPM and TROAP are involved in spindle function and may be key to helping abnormal spindles pass through cell cycle checkpoints.
The results of this study showed that ASPM and TROAP are strongly positively correlated, implying that these genes may interact with each other. The cell cycle analysis showed that ASPM and TROAP have similar expression profiles and high expression in the S&G2 phase. This suggests that these genes may promote tumor malignancy and development in the S&G2 phase of the cell cycle. Therefore, therapies targeting the ASPM and TROAP genes may be a potential treatment for malignant cancer.
Acknowledgments
Funding: This work was supported in part by the National Natural Science Foundation of China (No. 82072892), the Natural Science Foundation of Shanghai (No. 21ZR1454900), and the Key Discipline Project of Shanghai Jiading District (No. 2020-jdyxzdxk-13).
Footnote
Reporting Checklist: The authors have completed the STREGA reporting checklist. Available at https://atm.amegroups.com/article/view/10.21037/atm-22-1112/rc
Conflicts of Interest: All authors have completed the ICMJE uniform disclosure form (available at https://atm.amegroups.com/article/view/10.21037/atm-22-1112/coif). The authors have no conflicts of interest to declare.
Ethical Statement: The authors are accountable for all aspects of the work in ensuring that questions related to the accuracy or integrity of any part of the work are appropriately investigated and resolved. The study was conducted in accordance with the Declaration of Helsinki (as revised in 2013).
Open Access Statement: This is an Open Access article distributed in accordance with the Creative Commons Attribution-NonCommercial-NoDerivs 4.0 International License (CC BY-NC-ND 4.0), which permits the non-commercial replication and distribution of the article with the strict proviso that no changes or edits are made and the original work is properly cited (including links to both the formal publication through the relevant DOI and the license). See: https://creativecommons.org/licenses/by-nc-nd/4.0/.
References
- Feng RM, Zong YN, Cao SM, et al. Current cancer situation in China: good or bad news from the 2018 Global Cancer Statistics? Cancer Commun (Lond) 2019;39:22. [Crossref] [PubMed]
- Bray F, Ferlay J, Soerjomataram I, et al. Global cancer statistics 2018: GLOBOCAN estimates of incidence and mortality worldwide for 36 cancers in 185 countries. CA Cancer J Clin 2018;68:394-424. [Crossref] [PubMed]
- Blum A, Wang P, Zenklusen JC. SnapShot: TCGA-Analyzed Tumors. Cell 2018;173:530. [Crossref] [PubMed]
- Uhlen M, Oksvold P, Fagerberg L, et al. Towards a knowledge-based Human Protein Atlas. Nat Biotechnol 2010;28:1248-50. [Crossref] [PubMed]
- Clough E, Barrett T. The Gene Expression Omnibus Database. Methods Mol Biol 2016;1418:93-110. [Crossref] [PubMed]
- Gao ZY, Yu F, Jia HX, et al. ASPM predicts poor prognosis and regulates cell proliferation in bladder cancer. Kaohsiung J Med Sci 2020;36:1021-9. [Crossref] [PubMed]
- Feng Z, Zhang J, Zheng Y, et al. Overexpression of abnormal spindle-like microcephaly-associated (ASPM) increases tumor aggressiveness and predicts poor outcome in patients with lung adenocarcinoma. Transl Cancer Res 2021;10:983-97. [Crossref] [PubMed]
- Yuan YJ, Sun Y, Gao R, et al. Abnormal spindle-like microcephaly-associated protein (ASPM) contributes to the progression of Lung Squamous Cell Carcinoma (LSCC) by regulating CDK4. J Cancer 2020;11:5413-23. [Crossref] [PubMed]
- Jiang K, Rezabkova L, Hua S, et al. Microtubule minus-end regulation at spindle poles by an ASPM-katanin complex. Nat Cell Biol 2017;19:480-92. [Crossref] [PubMed]
- Chen X, Huang L, Yang Y, et al. ASPM promotes glioblastoma growth by regulating G1 restriction point progression and Wnt-β-catenin signaling. Aging (Albany NY) 2020;12:224-41. [Crossref] [PubMed]
- Yang S, Liu X, Yin Y, et al. Tastin is required for bipolar spindle assembly and centrosome integrity during mitosis. FASEB J 2008;22:1960-72. [Crossref] [PubMed]
- Ye J, Chu C, Chen M, et al. TROAP regulates prostate cancer progression via the WNT3/survivin signalling pathways. Oncol Rep 2019;41:1169-79. [PubMed]
- Li K, Zhang R, Wei M, et al. TROAP Promotes Breast Cancer Proliferation and Metastasis. Biomed Res Int 2019;2019:6140951. [Crossref] [PubMed]
- Hu H, Xu L, Chen Y, et al. The Upregulation of Trophinin-Associated Protein (TROAP) Predicts a Poor Prognosis in Hepatocellular Carcinoma. J Cancer 2019;10:957-67. [Crossref] [PubMed]
- Lian Y, Fan W, Huang Y, et al. Downregulated Trophinin-Associated Protein Plays a Critical Role in Human Hepatocellular Carcinoma Through Upregulation of Tumor Cell Growth and Migration. Oncol Res 2018;26:691-701. [Crossref] [PubMed]
- Schrock MS, Stromberg BR, Scarberry L, et al. APC/C ubiquitin ligase: Functions and mechanisms in tumorigenesis. Semin Cancer Biol 2020;67:80-91. [Crossref] [PubMed]
- Wu F, Dai X, Gan W, et al. Prostate cancer-associated mutation in SPOP impairs its ability to target Cdc20 for poly-ubiquitination and degradation. Cancer Lett 2017;385:207-14. [Crossref] [PubMed]
- Mao DD, Gujar AD, Mahlokozera T, et al. A CDC20-APC/SOX2 Signaling Axis Regulates Human Glioblastoma Stem-like Cells. Cell Rep 2015;11:1809-21. [Crossref] [PubMed]
- Paul D, Ghorai S, Dinesh US, et al. Cdc20 directs proteasome-mediated degradation of the tumor suppressor SMAR1 in higher grades of cancer through the anaphase promoting complex. Cell Death Dis 2017;8:e2882. [Crossref] [PubMed]
- Kidokoro T, Tanikawa C, Furukawa Y, et al. CDC20, a potential cancer therapeutic target, is negatively regulated by p53. Oncogene 2008;27:1562-71. [Crossref] [PubMed]
- Stegmeier F, Rape M, Draviam VM, et al. Anaphase initiation is regulated by antagonistic ubiquitination and deubiquitination activities. Nature 2007;446:876-81. [Crossref] [PubMed]
- Zhang Q, Huang H, Liu A, et al. Cell division cycle 20 (CDC20) drives prostate cancer progression via stabilization of β-catenin in cancer stem-like cells. EBioMedicine 2019;42:397-407. [Crossref] [PubMed]
- Chu Z, Zhang X, Li Q, et al. CDC20 contributes to the development of human cutaneous squamous cell carcinoma through the Wnt/β catenin signaling pathway. Int J Oncol 2019;54:1534-44. [Crossref] [PubMed]
- Szklarczyk D, Gable AL, Nastou KC, et al. The STRING database in 2021: customizable protein-protein networks, and functional characterization of user-uploaded gene/measurement sets. Nucleic Acids Res 2021;49:D605-12. [Crossref] [PubMed]
- Shannon P, Markiel A, Ozier O, et al. Cytoscape: a software environment for integrated models of biomolecular interaction networks. Genome Res 2003;13:2498-504. [Crossref] [PubMed]
- Pontén F, Jirström K, Uhlen M. The Human Protein Atlas--a tool for pathology. J Pathol 2008;216:387-93. [Crossref] [PubMed]
- Ouyang W, Winsnes CF, Hjelmare M, et al. Analysis of the Human Protein Atlas Image Classification competition. Nat Methods 2019;16:1254-61. [Crossref] [PubMed]
- Fu J, Wei C, He J, et al. Evaluation and characterization of HSPA5 (GRP78) expression profiles in normal individuals and cancer patients with COVID-19. Int J Biol Sci 2021;17:897-910. [Crossref] [PubMed]
- Schafer KA. The cell cycle: a review. Vet Pathol 1998;35:461-78. [Crossref] [PubMed]
- New insights into the regulation of cell cycle. J Mol Cell Biol 2018;10:1. [Crossref] [PubMed]
- Benz C, Urbaniak MD. Organising the cell cycle in the absence of transcriptional control: Dynamic phosphorylation co-ordinates the Trypanosoma brucei cell cycle post-transcriptionally. PLoS Pathog 2019;15:e1008129. [Crossref] [PubMed]
- Williams GH, Stoeber K. The cell cycle and cancer. J Pathol 2012;226:352-64. [Crossref] [PubMed]
- Woynarowski JM, Woynarowska BA, Trevino AV, et al. Cell cycle effects and induction of premitotic apoptosis by irofulven in synchronized cancer cells. Cancer Biol Ther 2004;3:1137-42; discussion 1143-4. [Crossref] [PubMed]
- Jiao Y, Li Y, Lu Z, et al. High Trophinin-Associated Protein Expression Is an Independent Predictor of Poor Survival in Liver Cancer. Dig Dis Sci 2019;64:137-43. [Crossref] [PubMed]
- Bond J, Roberts E, Mochida GH, et al. ASPM is a major determinant of cerebral cortical size. Nat Genet 2002;32:316-20. [Crossref] [PubMed]
- Zhong X, Liu L, Zhao A, et al. The abnormal spindle-like, microcephaly-associated (ASPM) gene encodes a centrosomal protein. Cell Cycle 2005;4:1227-9. [Crossref] [PubMed]
- Vulcani-Freitas TM, Saba-Silva N, Cappellano A, et al. ASPM gene expression in medulloblastoma. Childs Nerv Syst 2011;27:71-4. [Crossref] [PubMed]
- Williams SE, Garcia I, Crowther AJ, et al. Aspm sustains postnatal cerebellar neurogenesis and medulloblastoma growth in mice. Development 2015;142:3921-32. [PubMed]
- Fujimori A, Itoh K, Goto S, et al. Disruption of Aspm causes microcephaly with abnormal neuronal differentiation. Brain Dev 2014;36:661-9. [Crossref] [PubMed]
- Makhdoom EUH, Waseem SS, Iqbal M, et al. Modifier Genes in Microcephaly: A Report on WDR62, CEP63, RAD50 and PCNT Variants Exacerbating Disease Caused by Biallelic Mutations of ASPM and CENPJ. Genes (Basel) 2021;12:731. [Crossref] [PubMed]
- Maiato H, Logarinho E. Mitotic spindle multipolarity without centrosome amplification. Nat Cell Biol 2014;16:386-94. [Crossref] [PubMed]
- Choi JH, Ro JY. Cutaneous Spindle Cell Neoplasms: Pattern-Based Diagnostic Approach. Arch Pathol Lab Med 2018;142:958-72. [Crossref] [PubMed]
- Pease JC, Tirnauer JS. Mitotic spindle misorientation in cancer--out of alignment and into the fire. J Cell Sci 2011;124:1007-16. [Crossref] [PubMed]
- Carter MR, Hornick JL, Lester S, et al. Spindle cell (sarcomatoid) carcinoma of the breast: a clinicopathologic and immunohistochemical analysis of 29 cases. Am J Surg Pathol 2006;30:300-9. [Crossref] [PubMed]
- Bharadwaj R, Yu H. The spindle checkpoint, aneuploidy, and cancer. Oncogene 2004;23:2016-27. [Crossref] [PubMed]