TGFβ signaling: a friend or a foe to hepatic fibrosis and tumorigenesis
Transforming growth factor-β (TGFβ) signaling pathway is an important regulator of cell survival, proliferation, differentiation, migration and immunosurveillance (1). It translates extracellular cues into appropriate gene expression response. It possesses relatively simple machinery, but it is finely tuned to a variety of processes, both temporally and spatially at different levels, including ligand expression and activation, receptor complex formation, effector activation, modification and translocation and availability of transcriptional partners in the nucleus. Therefore, the readout of TGFβ signals strongly depends on the cellular context.
The TGFβ family consists of large number structurally and functionally related cytokines, all grouped in following subfamilies: TGFβ, BMPs, AMH, GDFs, activins and inhibins (2). There are three largely homologous TGFβ isoforms in humans: TGFβ1, TGFβ2 and TGFβ3. All TGFβ isoforms bind transmembrane receptor TGFβ receptor type II (TBR2), which leads to the recruitment of TGF-β receptor type I (TBR1) to the complex. Both receptors have serine/threonine kinase activity. Canonical TGFβ signaling propagates intracellular signal by the SMAD family of proteins. Upon ligand activation, the TBR1 phosphorylates SMAD2/3 at a serine-rich C-terminal motif, and the phospho-SMAD2/3 associates with SMAD4, subsequently being shuttled into the nucleus to regulate transcription. Availability of phospho-SMAD partners establishes the final output of the pathway. It determines which genes will be targeted, as well as will their expression be activated or repressed. In non-canonical pathway, TBR2 interacts with TGFβ-activated kinase 1 (TAK1), tumor necrosis factor receptor-associated factor 6 (TRAF6), phosphoinositide 3-kinase (PI3K), Akt, mitogen-activated protein kinase (MAPK), and integrin (3-5). Additional layer of complexity to the TGFβ pathway is related to its cooperation with other signaling pathways, including Wnt and Ras pathways (6,7).
TGFβ pathway has dual role in cancer progression. It has been shown that malignant cells have to avoid cytostatic effect of exogenous TGFβ for hepatocellular carcinoma (HCC) to develop. TBR2 expression and phosphorylation of SMAD3 were found to be down-regulated in human HCCs compared to adjacent, normal liver tissues (2). With the autonomous TGFβ pathway eliminated in malignant cells, cancer cells can generate TGFβ-rich tumor microenvironment that can favor tumor progression through its effect on tumor stroma. Moreover, residual epithelial TGFβ can additionally promote tumor progression by stimulating epithelial to mesenchymal transition (EMT).
A new study, published in Gastroenterology by Mu et al. offers insights into our understanding of hepatic epithelial TGFβ signaling pathway in hepatic fibrosis and during liver carcinogenesis. Using mice with cell-specific deletions of TBR2, the authors found that TGFβ signaling in the liver epithelial cells does not contribute to the liver fibrosis or to the development of DEN-induced HCC in mice. However, it constrains proliferation of cholangiocytes and prevents cholangiocarcinoma development in the context of hepatic PTEN deletion (8).
Using double transgenic mice expressing floxed TBR2 and Albumin-Cre to inhibit TGFβ signaling in the liver epithelium and mouse models of toxicity-induced fibrosis (CCl4 injections) and cholestatic liver fibrosis (common bile duct ligation and Mdr2 knockout mice), Mu et al. found that epithelial TGFβ signaling does not contribute to liver injury and does not contribute to biliary liver fibrosis. These observations somewhat contradict previously published studies (9,10). Dooley et al. (9) used SMAD7 overexpression to inhibit TGFβ signaling in liver epithelial cells, and observed decreased liver damage and fibrosis after CCl4 treatment. Possible reasons for conflicting results could be different mouse strains used (FVB vs. C57BL/6), different CCl4 treatment (3 times a week for 8 weeks vs. 8 injections total), or TGFβ-independent function of SMAD7. However, both studies do agree on the activation of TGFβ signaling in the liver epithelial cells after liver injury in the mouse models and in the patients with chronic liver disease.
Going forward, Mu et al. found that epithelial TGFβ signaling does not affect DEN induced HCCs. Mice lacking TBR2 developed tumors at the same rate as control mice when injected with DEN. In contrast to genotoxic hepatocarcinogenesis, Mu et al. observed significant role of TBR2 in tumorigenesis caused by PTEN loss. They generated a triple transgenic strain where Albumin-Cre drives loss of TBR2 and PTEN simultaneously in liver epithelium. Interestingly, the mice deficient for both TBR2 and PTEN develop tumors and die at 5–7 months of age, while the PTEN deficient mice are tumor free at that age. As expected, the tumors that developed in older single PTEN knockout mice were HCCs (Figure 1A), however, the tumors developed in double knockout background had characteristics of cholangiocarcinomas (Figure 1B). The tumors were keratin positive and had high expression of cholangiocyte and cholangiocarcinoma markers. Because Albumin-Cre causes deletion of TBR2 in both cholangiocytes and hepatocytes, they investigated which type of epithelial cells was affected by inhibition of TGFβ signaling in PTEN knockout background. They found that epithelial TGFβ signaling controls proliferation of cholangiocytes, but not hepatocytes.
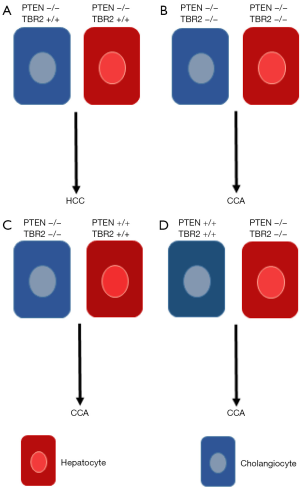
To distinguish between TGFβ signaling in hepatocytes vs. cholangiocytes, they used cell type specific ablation strategies to delete TBR2. For cholangiocyte-specific deletion of TBR2 and PTEN, they used two different types of triple-transgenic mice: one strain co-expressing Prom-1-CreERT2 with floxed PTEN and TBR2, and the other co-expressing K19-CreERT with floxed PTEN and TBR2. Because keratin19 and prominin are not specific only for cholangiocytes, they put mice on DDC diet to trigger cholestatic liver injury before tumors develop in the other organs, for example in pancreas. Mice without TBR2 in cholangiocytes developed cholangiocarcinomas faster (Figure 1C). They demonstrated that TGFβ signaling in cholangiocytes restricts their proliferation, protecting them from tumorigenesis. Using reporter mouse strain, Mu et al. showed that cell origin of cholangiocarcinoma in this mouse models are cholangiocytes.
To investigate role of TGFβ signaling in hepatocytes, they used double transgenic TBR2 and PTEN floxed mice and AAV8-TBG-Cre virus infection. In contrast to Albumin-Cre mice, all mice with hepatocyte-specific deletion of TBR2 and PTEN survived for 1 year. Surprisingly, when sacrificed at that age, they harbored tumors with characteristics of cholangiocarcinoma (Figure 1D). Interestingly, by co-labeling the Cre with GFP, they demonstrated that developed cholangiocarcinomas have hepatocyte origin. In non-tumor region of AAV8-TBG-Cre infected PTEN and TBR2 floxed mice, they identified, at low numbers, hepatocyte-derived progenitors that were GFP and keratin or osteopontin positive, suggesting that loss of TBR2 in PTEN-deficient hepatocytes results in cholangiocarcinoma development through an increase in proliferation of hepatocyte-derived cholangiocyte-like cells. Microarray studies where Mu et al., compared expression profile of these murine tumors with human cholangiocarcinomas, confirmed that this indeed are true cholangiocarcinomas and that they cluster with “proliferation” class human cholangiocarcinomas.
The results obtained by Mu et al. contrast data published by Yang et al. (10) in which TGFβ signaling in TAK-1 deleted livers contributes to liver fibrosis and tumorigenesis. Because the output of TGFβ signaling is highly cell context dependent, it is easy to imagine that different liver injury drivers will utilize TGFβ pathway differently. Furthermore, TAK-1 is a part of non-canonical TGF-β pathway hence inhibiting TGFβ can affect the driver of liver injury in this mouse model.
It is important to note that mutations in SMAD4 are prevalent in human cholangiocarcinomas (11,12), but not in human HCCs, which increases significance of this study. Cholangiocarcinomas are very aggressive form of human liver cancers, second by the frequency of incidence in liver cancer patients. However, a therapy does not exist. There is a genuine need for more extensive research in this area as well as a need for better animal models to aid the research. The murine models developed by Mu et al., can significantly contribute to our understanding of this type of liver cancer.
Additionally, Mu et al. suggested that hepatocytes could be the origin of cholangiocarcinoma in PTEN deficient livers, after they transdifferentiate into progenitors with cholangiocyte characteristics and succumb to unrestricted proliferation due to the lack of TGFβ control. How hepatocytes obtain cholangiocytes characteristics is an exciting question raised by this study.
Because TGFβ signaling in HSCs is the key pathway of liver fibrosis, targeting it holds a big promise in anti-fibrosis therapy. Indeed, there are several clinical trials for TGFβ inhibitory drugs. However, as suggested by Mu et al., one can cure fibrosis but inhibition of that signaling could increase risk of cholangiocarcinoma development.
Acknowledgements
Funding: P Raychaudhuri is supported by a grant (CA175380) from the National Cancer Institute and also by a Merit Review Grant (BX000131) from the Veteran Administration.
Footnote
Provenance: This is a Guest Commentary commissioned by Guest Editor Haitao Zhao, MD, PhD (Department of Liver Surgery, Peking Union Medical College Hospital, Chinese Academy of Medical Sciences and Peking Union Medical College, Beijing, China).
Conflicts of Interest: The authors have no conflicts of interest to declare.
References
- Massagué J. TGFβ signalling in context. Nat Rev Mol Cell Biol 2012;13:616-30. [Crossref] [PubMed]
- Zhang S, Sun WY, Wu JJ, et al. TGF-β signaling pathway as a pharmacological target in liver diseases. Pharmacol Res 2014;85:15-22. [Crossref] [PubMed]
- Javelaud D, Mauviel A. Crosstalk mechanisms between the mitogen-activated protein kinase pathways and Smad signaling downstream of TGF-beta: implications for carcinogenesis. Oncogene 2005;24:5742-50. [Crossref] [PubMed]
- Landström M. The TAK1-TRAF6 signalling pathway. Int J Biochem Cell Biol 2010;42:585-9. [Crossref] [PubMed]
- Margadant C, Sonnenberg A. Integrin-TGF-beta crosstalk in fibrosis, cancer and wound healing. EMBO Rep 2010;11:97-105. [Crossref] [PubMed]
- Remy I, Montmarquette A, Michnick SW. PKB/Akt modulates TGF-beta signalling through a direct interaction with Smad3. Nat Cell Biol 2004;6:358-65. [Crossref] [PubMed]
- Minoo P, Li C. Cross-talk between transforming growth factor-beta and Wingless/Int pathways in lung development and disease. Int J Biochem Cell Biol 2010;42:809-12. [Crossref] [PubMed]
- Mu X, Pradere JP, Affò S, et al. Epithelial Transforming Growth Factor-β Signaling Does Not Contribute to Liver Fibrosis but Protects Mice From Cholangiocarcinoma. Gastroenterology 2016;150:720-33. [Crossref] [PubMed]
- Dooley S, Hamzavi J, Ciuclan L, et al. Hepatocyte-specific Smad7 expression attenuates TGF-beta-mediated fibrogenesis and protects against liver damage. Gastroenterology 2008;135:642-59. [Crossref] [PubMed]
- Yang L, Inokuchi S, Roh YS, et al. Transforming growth factor-β signaling in hepatocytes promotes hepatic fibrosis and carcinogenesis in mice with hepatocyte-specific deletion of TAK1. Gastroenterology 2013;144:1042-1054.e4.
- Ong CK, Subimerb C, Pairojkul C, et al. Exome sequencing of liver fluke-associated cholangiocarcinoma. Nat Genet 2012;44:690-3. [Crossref] [PubMed]
- Chan-On W, Nairismägi ML, Ong CK, et al. Exome sequencing identifies distinct mutational patterns in liver fluke-related and non-infection-related bile duct cancers. Nat Genet 2013;45:1474-8. [Crossref] [PubMed]