Network pharmacology-based analysis of the effects of puerarin on sarcopenia
Introduction
The aging process is characterized by a variety of changes in body composition; for example, muscle mass peaks around the age of 30 and then begins to decline, with a loss of approximately 20–40% by the age of 70, and corresponding muscle dysfunction may occur (1). One study reported that after the age of 50, leg muscle mass decreases by 1–2% per year and muscle strength decreases by 1.5–5% per year (2).
The concept of sarcopenia was first introduced by Rosenberg in 1989 (3) and was included in the International Classification of Disease 10th revision as a chronic muscle disease (M62.84) in 2016 (4). Under the 2019 Consensus Update on Sarcopenia Diagnosis and Treatment, sarcopenia is defined as an age-related reduction in muscle mass, accompanied by a decrease in muscle strength and/or reduced physical performance (5). Sarcopenia can be divided into primary and secondary sarcopenia. Primary sarcopenia is a manifestation of aging and is common in the elderly population, while secondary sarcopenia can be associated with reduced activity, disease, and malnutrition (6). The prevalence of sarcopenia ranges from 5.5–25.7%, and it is more prevalent in men than women (5). Sarcopenia is a complex disease affected by environmental and genetic factors, and its pathogenesis has not yet been fully elucidated. It may be related to neuromuscular dysfunction (7), age-related hormone changes, inflammation, myocyte apoptosis, genetic factors, nutritional factors, and inactivity (8). Sarcopenia increases the likelihood of adverse outcomes, such as falls, fractures, disability, and death (8,9). It can also increase the hospitalization rates and medical expenses of the elderly, seriously affect their quality of life, and even shorten their life expectancy. The prevalence of sarcopenia increases with age (10,11), and it is expected that by 2050, >200 million people worldwide will suffer from sarcopenia (12). With the acceleration of population aging, sarcopenia will place a heavy burden on families and society (13), which has attracted more and more attention worldwide. Thus, effective treatments are urgently needed to slow down the development of sarcopenia.
The treatment of sarcopenia (14) mainly comprises exercise interventions (15-17), nutrition interventions (18), and pharmacological interventions (19). For some patients, exercise therapy is limited by advanced age and disease conditions, while evidence of the effectiveness of nutritional interventions alone has not yet been gathered by high-quality studies (8,20). Thus, the development of effective therapeutic drugs is of great importance. No specific drugs have been approved for the treatment of sarcopenia, and clinical guidelines have recommended some drugs that may be beneficial to the skeletal muscle, such as vitamin D, anabolic hormones, growth hormone, antibodies to myostatin or activin II receptors, angiotensin converting enzyme inhibitors and β adrenergic receptor antagonists. However, the efficiency and safety of new medications is currently unknown, and there is inadequate evidence to recommend the use of any of these drugs at present for the management of sarcopenia. The development of new drugs is a time-consuming, laborious, and high-risk process. As the famous saying goes, the best way to discover a new drug is to start with an old one. If new indications are discovered for existing drugs, time and resources need not be expended on the development of new drugs. Thus, this approach could prove both efficient and cost effective.
Puerarin is an effective monomer isolated from the traditional Chinese medicine Pueraria lobata (Willd.) Ohwi, which has various pharmacological activities, such as anti-inflammatory, anti-oxidant, vasodilation, and apoptosis-regulating effects. It is relatively inexpensive and easy to extract, making it a promising natural agent with broad application prospects. Puerarin has been used clinically to treat a variety of diseases, such as cardiovascular disease, retinal arteriovenous obstruction, sudden deafness, diabetes, and diabetic complications (21,22). The pathogenesis of sarcopenia is associated with inflammation (23-25), oxidative stress (26,27), and myocyte apoptosis (28-30), and puerarin has anti-inflammatory, anti-oxidant, and apoptosis-regulating effects (31). Thus, we conjectured that puerarin may have therapeutic effects in the treatment of sarcopenia. Research has shown that puerarin reduces skeletal muscle atrophy in diabetic rats (32) and mice with sciatic nerve injury (33). It has also been shown that Radix Pueraria lobata, the source of puerarin, prevented skeletal muscle atrophy induced by a high-fat diet in mice (34). However, further investigations need to be conducted to determine whether puerarin can ameliorate other causes of muscle atrophy or sarcopenia and its specific mechanism of action.
With the development of computer and high-throughput omics technology, a large number of bioinformatics libraries have been established. Network pharmacology, based on systems biology and pharmacology, enables the prediction of hidden associations between drugs, diseases, and targets. The research method of network pharmacology is to obtain disease-related targets and drug intervention targets through high-throughput sequencing or public databases, and then identify the targets of drug intervention in the disease and construct a protein-protein interaction network, screen out core targets and conduct enrichment analysis, and finally explain the mechanism of drug action on the disease from the perspective of biological network balance. A network pharmacological strategy can be adopted to systematically study the intervention mechanisms of drugs on diseases, thereby providing a theoretical basis for drug development and elucidating the mechanisms of action. There are many literatures on the mechanism of action of puerarin based on network pharmacology. However, no research has been reported on the mechanism of action of puerarin on sarcopenia using a network pharmacological approach. Thus, the present study was designed to explore the potential targets and molecular mechanisms of puerarin in the treatment of sarcopenia through a network pharmacological approach to provide a theoretical basis for subsequent drug development and pharmacological experiments. We present the following article in accordance with the MDAR reporting checklist (available at https://atm.amegroups.com/article/view/10.21037/atm-22-2360/rc).
Methods
Identification of potential therapeutic targets of puerarin
The targets of puerarin were obtained from the Traditional Chinese Medicine Systems Pharmacology Database and Analysis Platform (TCMSP) database (35) (https://tcmsp-e.com) and standardized through the UniProt Database (36) (https://www.uniprot.org).
Screening of targets related to sarcopenia
The keyword “sarcopenia” was used to search the GeneCards database (37) (https://www.genecards.org), the DisGeNET database (38) (https://www.disgenet.org), the Online Mendelian Inheritance in Man (OMIM) database (39) (https://www.omim.org), and the Therapeutic Target Database (TTD) (40) (http://db.idrblab.net/ttd/) for potential targets of sarcopenia. In the Genecards database, the score value reflects the association between the target and the disease, and targets with a score ≥ median were selected as potential targets for sarcopenia. Similarly, the screening threshold in the DisGeNET database was set as a Scoregda ≥ maximum × 0.3. We merged the targets from the 4 databases, removed duplicate targets, and standardized the targets through the UniProt database. The study was conducted in accordance with the Declaration of Helsinki (as revised in 2013).
Protein-protein interaction (PPI) network construction and core targets screening
PPI networks of puerarin and sarcopenia were constructed using the Cytoscape 3.8.2 (41) plug-in BisoGenet (42), and the intersection network of the 2 PPI networks was extracted using the Merge function in Cytoscape. The median degree was calculated, and all nodes with a degree ≥ twice the median (i.e., the “Hit hubs”) were selected. We used the CytoNCA plug-in (43) to analyze the topological parameters of each node in the Hit-hubs network, and calculated the median of degree, betweenness, and closeness of these nodes. Nodes met the following conditions at the same time were selected as the core targets: attribute values ≥ the median of degree, attribute values ≥ the median of betweenness, and attribute values ≥ the median of closeness. The resultant network was analyzed by the Molecular Complex Detection (MCODE) algorithm to identify the densely connected network components, and a pathway and process enrichment analysis was then applied to each MCODE component independently. The 3 best-scoring terms by P value were retained as functional descriptions of the corresponding components.
Gene Ontology (GO) and Kyoto Encyclopedia of Genes and Genomes (KEGG) enrichment analysis of core targets
Core targets were imported into the Metascape platform (44) (http://metascape.org/gp/index.html) for the GO and KEGG enrichment analyses. The GO analysis examined the following 3 aspects (45): biological process (BP), cellular component (CC), and molecular function (MF). The results of the BP, CC, MF, and KEGG data were saved, and the top 20 items with the lowest P values were selected for visualization using the online bioinformatics tool (http://www.bioinformatics.com.cn).
Statistical analysis
The topological analysis was performed by Cytoscape version 3.8.2. for Windows. GO and KEGG enrichment analysis was performed by Metascape platform. The statistical significance threshold of enrichment analysis was P<0.05.
Results
Identification of potential therapeutic targets of puerarin
Potential therapeutic targets of puerarin were obtained from the TCMSP database, which were then verified by UniProt to exclude invalid targets. Ultimately, 53 targets were identified (see Figure 1).
Screening of targets related to sarcopenia
The GeneCards database, DisGeNET database, OMIM database, and TTD were searched and filtered according to the corresponding conditions, and 113, 18, 4, and 0 targets were obtained, respectively. After verification by UniProt and the removal of duplicated targets, a total of 129 sarcopenia-related targets remained (see Figure 2).
PPI network construction and screening of core targets
From the PPI network constructed by Bisogenet, we found that the potential targets of puerarin could interact directly or indirectly with 3,500 targets, and their interrelationships reached 86,431; there were 3,828 targets directly or indirectly related to sarcopenia, and the interrelationships between the targets reached 93,928. Taking the intersection of the 2 networks, we established a PPI network comprising 2,148 nodes and 58,905 edges (see Figure 3).
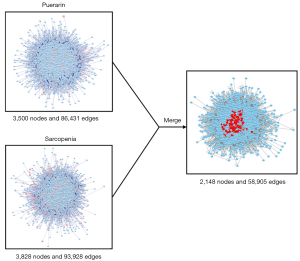
The CytoNCA plug-in was applied to calculate the topological parameters of each node in the intersection PPI network, and a total of 206 core targets were obtained after screening. The screening strategy is shown in Figure 4A, and the PPI network of the core targets is shown in Figure 4B.
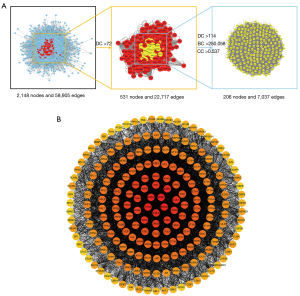
The core PPI network was analyzed by the MCODE algorithm to identify the densely connected network components, which were considered to have specific biological functions (see Table 1). Ultimately, 3 sets with biological significance were obtained. The representative BPs were related to telomere organization, protein synthesis, and protein catabolic process.
Table 1
Module network | GO term | Description | Log10 (P) |
---|---|---|---|
![]() |
GO: 0032200 | Telomere organization | –55.4 |
GO: 0006335 | DNA replication-dependent nucleosome assembly | –52.1 | |
GO: 0034723 | DNA replication-dependent nucleosome organization | –52.1 | |
![]() |
GO: 0002181 | Cytoplasmic translation | –17.2 |
GO: 0006412 | Translation | –16.6 | |
GO: 0043043 | Peptide biosynthetic process | –16.4 | |
![]() |
GO: 0006511 | Ubiquitin-dependent protein catabolic process | –5.7 |
GO: 0019941 | Modification-dependent protein catabolic process | –5.7 | |
GO: 0043632 | Modification-dependent macromolecule catabolic process | –5.6 |
PPI, protein-protein interaction; GO, Gene Ontology.
GO and KEGG enrichment analyses of core targets
The Metascape platform was used to perform the GO and KEGG enrichment analyses of the 206 core targets. The top 20 items with the lowest P values for the BP, CC, MF, and KEGG results were selected and visualized in a bubble chart (see Figure 5). The size of the bubble represents the number of enriched targets, and the color of the bubble indicates the P value of enrichment.
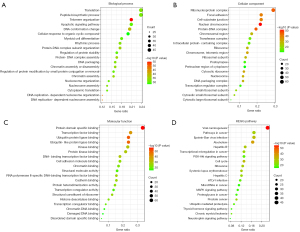
As Figure 5 shows, the BPs of the top enriched GO terms included translation, peptide biosynthetic process, telomere organization, the apoptotic signaling pathway, and regulation of protein stability. These BPs were related to cell cycle, apoptosis, protein anabolism and catabolism, suggesting that puerarin may play a regulatory role in cell cycle, apoptosis, and skeletal muscle protein homeostasis in sarcopenia. In the analysis of the CCs, the relevant targets were enriched in the ribonucleoprotein complex, protein-deoxyribonucleic acid (DNA) complex, transferase complex, ribosome, and transcription regulator complex. The MFs of the relevant targets included protein domain specific binding, transcription factor binding, ubiquitin protein ligase binding, ubiquitin-like protein ligase binding, and kinase binding.
Figure 5D shows the top 20 pathways of the KEGG enrichment analysis in terms of the P values, including the phosphatidylinositol-3-kinase and protein kinase B (PI3K-Akt) signaling pathway, cell cycle, microtubule-associated protein kinase (MAPK) signaling pathway, and ubiquitin-mediated proteolysis. Additionally, the forkhead transcription factors of the O class (FOXO) signaling pathway, apoptosis, nuclear factor kappa B (NF-κB) signaling pathway, and mammalian target of rapamycin (mTOR) signaling pathway were also enriched and statistically significant.
Discussion
The pathogenesis of sarcopenia is complex, while network pharmacology shows a unique advantage in predicting and explaining the action mechanism of traditional Chinese medicine. Although network pharmacology is widely used in traditional Chinese medicine, the study on sarcopenia is relatively scarce and on the primary stage. Zhao et al. (46) explored the mechanism of Magnoliae Cortex on sarcopenia through network pharmacological methods, and found four core targets, including AKT1, EGFR, INS and PIK3CA. Enrichment analysis found that the mechanism of drug action may relate to PI3K-Akt signaling pathway, EGFR tyrosine kinase inhibitor resistance, longevity regulating pathway, and other cellular and innate immune signaling pathways. It showed that Magnoliae Cortex may be a candidate drug for sarcopenia. This provided a theoretical basis for further pharmacological research. However, this study has not been verified by experiments. Future studies can be carried out to clarify the intervention effect and mechanism of Magnoliae Cortex on sarcopenia based on the results of network pharmacology.
In the present study, through a network pharmacological approach, we screened out the core targets and core networks of puerarin in the treatment of sarcopenia, and then performed GO and KEGG enrichment analyses to identify the functions of the targets, thereby revealing the potential pharmacological mechanisms of puerarin in treating sarcopenia, which can provide a theoretical basis for subsequent drug development and pharmacological experiments.
We obtained 53 targets of puerarin from the TCMSP database, including AKT1, BCL2, BAX, CASP3, NFKBIA, TNF, SOD1, etc. These targets are related to cell growth, apoptosis, inflammation, and oxidative stress. As the targets are interconnected in a large interaction network, the effects of drugs on diseases are not limited to the direct effects, and any study of drug action mechanisms should also explore the indirect action in the PPI network. In this study, a PPI network of 53 targets of puerarin was constructed to establish a network comprising 3,500 nodes, which was the potential target network of puerarin acting on the human body. Similarly, we obtained 129 targets for sarcopenia from the GeneCards database, DisGeNET database, OMIM database, and TTD, including AKT1, mTOR, FOXO, BCL2, BAX, NFKB1, TNF, SOD1, etc. These targets are also related to cell growth, apoptosis, inflammation and oxidative stress. AKT1, BCL2, BAX, TNF and SOD1 are the main common targets of puerarin and sarcopenia, indicating that puerarin may have therapeutic effects on sarcopenia through the regulation of cell growth, apoptosis, inflammation and oxidative stress. The PPI network was also constructed, resulting in a network comprising 3,828 nodes, which was the network of potential targets related to sarcopenia. Taking the intersection of the 2 networks, a PPI network comprising 2,148 nodes was established, which was the potential target network of puerarin in the treatment of sarcopenia. To further determine the core network, we calculated the topological parameters of each node. The Hit-hubs network was first extracted by selecting nodes with a degree ≥ twice the median, and a core network comprising 206 nodes, which were considered the key targets of puerarin in the treatment of sarcopenia, was then screened out by degree, betweenness, and closeness values. The PPI network was analyzed using the MCODE algorithm, and 3 sets with biological significance were obtained. The representative BPs were related to telomere organization, protein synthesis, and proteolysis, indicating that the mechanism of puerarin in the treatment of sarcopenia may be related to the regulation of telomere organization and protein metabolism. To analyze the functions of these targets and the related pathways, we performed GO functional annotation and KEGG pathway enrichment analyses. We found that the functions of these targets included ubiquitin ligase binding, transcription factor binding, and kinase binding. The BPs were associated with telomere organization, apoptosis, protein synthesis, and proteolysis. The signaling pathways involved included cell cycle, apoptosis, the PI3K-Akt signaling pathway, and the mTOR signaling pathway, which were related to protein synthesis, and pathways associated with proteolysis, such as the MAPK signaling pathway, NF-κB signaling pathway, FOXO signaling pathway, and ubiquitin-mediated proteolysis.
In healthy adult muscle tissue, skeletal muscle satellite cells are in the quiescent phase and have a number of functions, such as self-repair and the renewal of muscle fibers (47). When the skeletal muscle system suffers from injury, skeletal muscle satellite cells may be activated to proliferate and differentiate into myoblasts. Myoblasts have the ability to proliferate, differentiate, fuse to form multinucleated myotubes, and develop into mature muscle fibers. In the presence of myogenic regulatory factors, myoblasts proliferate and accumulate in muscle tissue, and then initiate the differentiation program. Myoblasts exit the cell cycle in an irreversible manner, gradually fuse to form multinucleated myotubes, and further differentiate to form myofibers, which migrate to injured muscle tissue to achieve the self-repair of muscle fibers (47,48). The process of myoblast proliferation and fusion to form myotubes governs the development of skeletal muscle. The change in skeletal muscle mass is the result of a change in the balance of protein synthesis and catabolism (49).
It has been suggested that the decline of the number of skeletal muscle satellite cells is an important cause of the development of sarcopenia (50). With the aging of the body, both the number of skeletal muscle satellite cells and the skeletal muscle mass declines (51). Thus, it is important to promote the proliferation, differentiation, and fusion of myoblasts, and maintain the balance between skeletal muscle protein synthesis and degradation metabolism, which will be conducive to the repair and regeneration of skeletal muscle tissue and is of great significance in the prevention and treatment of sarcopenia. In the present study, we found that the functions and pathways involved in the core targets of puerarin in the treatment of sarcopenia were related to cell cycle and cell proliferation; thus, it was hypothesized that puerarin plays a therapeutic role in sarcopenia by regulating the proliferation of myoblasts. However, no few research has been conducted to examine the effect of puerarin on myoblasts, and future studies need to be conducted to explore whether puerarin promotes the proliferation and differentiation of myoblasts and thus plays a therapeutic role in sarcopenia.
The pathogenesis of sarcopenia is associated with the disturbance of muscle protein homeostasis, manifested by a decrease in muscle protein anabolism and an increase in catabolism (52). The PI3K-Akt signaling pathway is one of the key pathways that regulate protein homeostasis, promoting protein synthesis and inhibiting protein degradation. The activation of the PI3K-Akt signaling pathway inhibits glycogen synthase kinase-3 (GSK3) and activates mammalian target of rapamycin complex-1 (mTORC-1), thereby increasing protein synthesis. Additionally, PI3K-Akt activation also phosphorylates and inactivates the FOXO transcription factor, thereby preventing protein degradation (52-56). Yin et al. found that puerarin increased protein synthesis through the upregulation of the Akt-mTOR signaling pathway and the inhibition of autophagy, thereby ameliorating muscle wasting induced by high glucose in L6 myotubes; in addition, phosphorylation of the transcription factor FOXO3a was also shown to be upregulated by puerarin (32). In the present study, the PI3K-Akt signaling pathway, mTOR signaling pathway, and FOXO signaling pathway were enriched in the KEGG analysis. Thus, it is hypothesized that puerarin may affect the synthesis and degradation of skeletal muscle proteins by regulating the PI3K-Akt signaling pathway, mTOR signaling pathway, and FOXO signaling pathway, thus exerting a therapeutic effect on sarcopenia.
Protein degradation is mainly mediated by the following 4 mechanisms (52): (I) the ubiquitin proteasomal system (UPS) (57); (II) the lysosomal proteolytic system (58,59); (III) the calcium-dependent calpains (60); and (IV) the cysteine-dependent aspartate-specific proteases (61). When skeletal muscle is stimulated by oxidative stress and inflammatory cytokines, muscle RING finger-containing protein 1 (MuRF-1) and muscle atrophy F-box protein (MAFbx) can be activated through the MAPK and NF-κB signaling pathways, which can activate the ubiquitin-proteasome system and thus promote proteolysis (52).
The FOXO signaling pathway is also asso ciated with protein degradation. The FOXO transcription factor upregulates the expression of MuRF-1 and MAFbx, which induces UPS proteasomal degradation. Notably, it also promotes the autophagy-lysosomal system by controlling the transcription of core components of autophagosomes and lysosomes (56,62-64). Yin et al. found that puerarin downregulates the expression of muscle atrophy markers, such as Atrogin-1 and MuRF-1, in diabetic rats (32). In the present study, the MAPK signaling pathway, the NF-κB signaling pathway, ubiquitin-mediated proteolysis, and the FOXO signaling pathway were enriched in the KEGG analysis. Thus, it is speculated that puerarin may affect skeletal muscle proteolysis by regulating the MAPK signaling pathway, the NF-κB signaling pathway, ubiquitin-mediated proteolysis, and the FOXO signaling pathway, and thus play a therapeutic role in sarcopenia.
Another interesting finding from our study was that the therapeutic effects of puerarin on sarcopenia might also be achieved via the regulation of apoptosis. Myocyte apoptosis and mitochondrial dysfunction are associated with muscle mass loss (52,65), which might be the pathogenesis of sarcopenia. Park et al. found that the apoptosis of semitendinosus skeletal muscle cells increased with age (66). It has also been reported that the level of myocyte apoptosis is significantly higher in the elderly than the young (67). Aging, oxidative stress, low growth factors, and immobility can trigger the caspase-dependent or caspase-independent pathways that lead to cell death. Li et al. found that puerarin reduced apoptosis in cardiac myocytes (68). In the present study, the apoptosis signaling pathway was enriched in the KEGG analysis. Thus, it is hypothesized that puerarin may have a therapeutic effect on sarcopenia via the regulation of apoptosis.
Network pharmacology integrates multiple disciplines such as systems biology and computational biology, and can explore the relationship between drugs and diseases on a holistic level. It saves the cost for the development of new drugs, and to some extent, can break through the limitations of “single component-single target-single pathway” in traditional Chinese medicine research. At present, the application of network pharmacology in traditional Chinese medicine mainly includes the identification of active pharmaceutical ingredients, the elucidation of pharmacological mechanisms, and the development of new drugs. Although network pharmacology has many advantages, it still faces some challenges. Firstly, network pharmacology is based on databases and computer analysis, the databases may have some limitations, and the reliability of the results of network pharmacology needs to be further verified. Secondly, some of the active pharmaceutical ingredients identified by network pharmacology have a low content in the original drugs, which may only have theoretical significance and do not play important roles in clinical practice. Thirdly, the efficacy of drugs could not be detected by network pharmacology, which need to be verified by experiments.
In this study, through a network pharmacological approach, we find that puerarin might have protective effects on sarcopenia through the regulation of protein homeostasis, cell cycle and myocyte apoptosis. Further experiments need to be conducted to explore the protective effects of puerarin on sarcopenia, and real-time quantitative polymerase chain reaction (qPCR) and western blot technologies could be used to verify the molecular mechanisms of puerarin on sarcopenia. Sarcopenia mouse model could be constructed, and the efficacy of puerarin in the treatment of sarcopenia can be evaluated by muscle mass, muscle strength, and body function. The tolerability of puerarin in the treatment of sarcopenia can be evaluated by the incidence of adverse reactions, such as nausea, vomit, allergic reactions, etc. The PI3K-Akt signaling pathway is one of the key pathways that regulate protein homeostasis, promoting protein synthesis and inhibiting protein degradation. In our study, AKT1 is the common target of puerarin and sarcopenia, and PI3K-Akt signaling pathway has the largest gene count in pathways related to sarcopenia from KEGG analysis. Therefore, we recommend that further studies focus on this pathway. We could explore the messenger ribonucleic acid (mRNA) and protein expressions of relevant genes and proteins using qPCR and western blot techniques, and functional experiments could be carried out through the activation or inhibition of Akt.
Conclusions
In this study, the possible use of puerarin in the treatment of sarcopenia was proposed, and the potential targets and mechanisms of action were explored through a network pharmacological approach. Our findings suggest that puerarin targets multiple proteins and subsequently initiates complex signal transduction regulating protein homeostasis, cell cycle and myocyte apoptosis, which in turn restrain the progression of sarcopenia and promote the prognosis of patients. Our findings provide a theoretical basis for drug development and pharmacological experiments for sarcopenia. Further in-vivo and in-vitro experiments need to be conducted to explore the protective effects of puerarin on sarcopenia.
Acknowledgments
The authors would like to express their gratitude to everyone who participated in this work and public databases (TCMSP, GeneCards, DisGeNET, OMIM, TTD, UniProt, Metascape).
Funding: None.
Footnote
Reporting Checklist: The authors have completed the MDAR reporting checklist. Available at https://atm.amegroups.com/article/view/10.21037/atm-22-2360/rc
Conflicts of Interest: All authors have completed the ICMJE uniform disclosure form (available at https://atm.amegroups.com/article/view/10.21037/atm-22-2360/coif). The authors have no conflicts of interest to declare.
Ethical Statement: The authors are accountable for all aspects of the work in ensuring that questions related to the accuracy or integrity of any part of the work are appropriately investigated and resolved. The study was conducted in accordance with the Declaration of Helsinki (as revised in 2013).
Open Access Statement: This is an Open Access article distributed in accordance with the Creative Commons Attribution-NonCommercial-NoDerivs 4.0 International License (CC BY-NC-ND 4.0), which permits the non-commercial replication and distribution of the article with the strict proviso that no changes or edits are made and the original work is properly cited (including links to both the formal publication through the relevant DOI and the license). See: https://creativecommons.org/licenses/by-nc-nd/4.0/.
References
- Kalyani RR, Corriere M, Ferrucci L. Age-related and disease-related muscle loss: the effect of diabetes, obesity, and other diseases. Lancet Diabetes Endocrinol 2014;2:819-29. [Crossref] [PubMed]
- Keller K, Engelhardt M. Strength and muscle mass loss with aging process. Age and strength loss. Muscles Ligaments Tendons J 2013;3:346-50. [Crossref] [PubMed]
- Rosenberg IH. Summary comments. The American Journal of Clinical Nutrition 1989;50:1231-3. [Crossref]
- Cao L, Morley JE. Sarcopenia Is Recognized as an Independent Condition by an International Classification of Disease, Tenth Revision, Clinical Modification (ICD-10-CM) Code. J Am Med Dir Assoc 2016;17:675-7. [Crossref] [PubMed]
- Chen LK, Woo J, Assantachai P, et al. Asian Working Group for Sarcopenia: 2019 Consensus Update on Sarcopenia Diagnosis and Treatment. J Am Med Dir Assoc 2020;21:300-307.e2. [Crossref] [PubMed]
- Cruz-Jentoft AJ, Bahat G, Bauer J, et al. Sarcopenia: revised European consensus on definition and diagnosis. Age Ageing 2019;48:16-31. [Crossref] [PubMed]
- Dobrowolny G, Barbiera A, Sica G, et al. Age-Related Alterations at Neuromuscular Junction: Role of Oxidative Stress and Epigenetic Modifications. Cells 2021;10:1307. [Crossref] [PubMed]
- Cruz-Jentoft AJ, Sayer AA. Sarcopenia. Lancet 2019;393:2636-46. [Crossref] [PubMed]
- Kitamura A, Seino S, Abe T, et al. Sarcopenia: prevalence, associated factors, and the risk of mortality and disability in Japanese older adults. J Cachexia Sarcopenia Muscle 2021;12:30-8. [Crossref] [PubMed]
- Volpato S, Bianchi L, Cherubini A, et al. Prevalence and clinical correlates of sarcopenia in community-dwelling older people: application of the EWGSOP definition and diagnostic algorithm. J Gerontol A Biol Sci Med Sci 2014;69:438-46. [Crossref] [PubMed]
- Gariballa S, Alessa A. Sarcopenia: prevalence and prognostic significance in hospitalized patients. Clin Nutr 2013;32:772-6. [Crossref] [PubMed]
- Cruz-Jentoft AJ, Baeyens JP, Bauer JM, et al. Sarcopenia: European consensus on definition and diagnosis: Report of the European Working Group on Sarcopenia in Older People. Age Ageing 2010;39:412-23. [Crossref] [PubMed]
- Dhillon RJ, Hasni S. Pathogenesis and Management of Sarcopenia. Clin Geriatr Med 2017;33:17-26. [Crossref] [PubMed]
- Dent E, Morley JE, Cruz-Jentoft AJ, et al. International Clinical Practice Guidelines for Sarcopenia (ICFSR): Screening, Diagnosis and Management. J Nutr Health Aging 2018;22:1148-61. [Crossref] [PubMed]
- Vlietstra L, Hendrickx W, Waters DL. Exercise interventions in healthy older adults with sarcopenia: A systematic review and meta-analysis. Australas J Ageing 2018;37:169-83. [Crossref] [PubMed]
- Lozano-Montoya I, Correa-Pérez A, Abraha I, et al. Nonpharmacological interventions to treat physical frailty and sarcopenia in older patients: a systematic overview - the SENATOR Project ONTOP Series. Clin Interv Aging 2017;12:721-40. [Crossref] [PubMed]
- Hita-Contreras F, Bueno-Notivol J, Martínez-Amat A, et al. Effect of exercise alone or combined with dietary supplements on anthropometric and physical performance measures in community-dwelling elderly people with sarcopenic obesity: A meta-analysis of randomized controlled trials. Maturitas 2018;116:24-35. [Crossref] [PubMed]
- Robinson SM, Reginster JY, Rizzoli R, et al. Does nutrition play a role in the prevention and management of sarcopenia? Clin Nutr 2018;37:1121-32. [Crossref] [PubMed]
- De Spiegeleer A, Beckwée D, Bautmans I, et al. Pharmacological Interventions to Improve Muscle Mass, Muscle Strength and Physical Performance in Older People: An Umbrella Review of Systematic Reviews and Meta-analyses. Drugs Aging 2018;35:719-34. [Crossref] [PubMed]
- Bhasin S, Apovian CM, Travison TG, et al. Effect of Protein Intake on Lean Body Mass in Functionally Limited Older Men: A Randomized Clinical Trial. JAMA Intern Med 2018;178:530-41. [Crossref] [PubMed]
- Zhou YX, Zhang H, Peng C. Puerarin: a review of pharmacological effects. Phytother Res 2014;28:961-75. [Crossref] [PubMed]
- Zhang L. Pharmacokinetics and drug delivery systems for puerarin, a bioactive flavone from traditional Chinese medicine. Drug Deliv 2019;26:860-9. [Crossref] [PubMed]
- Wilson D, Jackson T, Sapey E, et al. Frailty and sarcopenia: The potential role of an aged immune system. Ageing Res Rev 2017;36:1-10. [Crossref] [PubMed]
- Pan L, Xie W, Fu X, et al. Inflammation and sarcopenia: A focus on circulating inflammatory cytokines. Exp Gerontol 2021;154:111544. [Crossref] [PubMed]
- Chhetri JK, de Souto Barreto P, Fougère B, et al. Chronic inflammation and sarcopenia: A regenerative cell therapy perspective. Exp Gerontol 2018;103:115-23. [Crossref] [PubMed]
- Pascual-Fernández J, Fernández-Montero A, Córdova-Martínez A, et al. Sarcopenia: Molecular Pathways and Potential Targets for Intervention. Int J Mol Sci 2020;21:8844. [Crossref] [PubMed]
- Bernabeu-Wittel M, Gómez-Díaz R, González-Molina Á, et al. Oxidative Stress, Telomere Shortening, and Apoptosis Associated to Sarcopenia and Frailty in Patients with Multimorbidity. J Clin Med 2020;9:2669. [Crossref] [PubMed]
- Cho J, Choi Y, Sajgalik P, et al. Exercise as a Therapeutic Strategy for Sarcopenia in Heart Failure: Insights into Underlying Mechanisms. Cells 2020;9:2284. [Crossref] [PubMed]
- Cheema N, Herbst A, McKenzie D, et al. Apoptosis and necrosis mediate skeletal muscle fiber loss in age-induced mitochondrial enzymatic abnormalities. Aging Cell 2015;14:1085-93. [Crossref] [PubMed]
- Marzetti E, Leeuwenburgh C. Skeletal muscle apoptosis, sarcopenia and frailty at old age. Exp Gerontol 2006;41:1234-8. [Crossref] [PubMed]
- Zhou BG, Zhao HM, Lu XY, et al. Effect of Puerarin Regulated mTOR Signaling Pathway in Experimental Liver Injury. Front Pharmacol 2018;9:1165. [Crossref] [PubMed]
- Yin L, Chen X, Li N, et al. Puerarin ameliorates skeletal muscle wasting and fiber type transformation in STZ-induced type 1 diabetic rats. Biomed Pharmacother 2021;133:110977. [Crossref] [PubMed]
- Wu M, Zhao G, Yang X, et al. Puerarin accelerates neural regeneration after sciatic nerve injury. Neural Regen Res 2014;9:589-93. [Crossref] [PubMed]
- Jung HW, Kang AN, Kang SY, et al. The Root Extract of Pueraria lobata and Its Main Compound, Puerarin, Prevent Obesity by Increasing the Energy Metabolism in Skeletal Muscle. Nutrients 2017;9:33. [Crossref] [PubMed]
- Ru J, Li P, Wang J, et al. TCMSP: a database of systems pharmacology for drug discovery from herbal medicines. J Cheminform 2014;6:13. [Crossref] [PubMed]
- UniProt Consortium. UniProt: the universal protein knowledgebase in 2021. Nucleic Acids Res 2021;49:D480-9. [Crossref] [PubMed]
- Stelzer G, Rosen N, Plaschkes I, et al. The GeneCards Suite: From Gene Data Mining to Disease Genome Sequence Analyses. Curr Protoc Bioinformatics 2016;54:1.30.1-1.30.33.
- Piñero J, Bravo À, Queralt-Rosinach N, et al. DisGeNET: a comprehensive platform integrating information on human disease-associated genes and variants. Nucleic Acids Res 2017;45:D833-9. [Crossref] [PubMed]
- Amberger JS, Bocchini CA, Schiettecatte F, et al. OMIM.org: Online Mendelian Inheritance in Man (OMIM®), an online catalog of human genes and genetic disorders. Nucleic Acids Res 2015;43:D789-98. [Crossref] [PubMed]
- Li YH, Yu CY, Li XX, et al. Therapeutic target database update 2018: enriched resource for facilitating bench-to-clinic research of targeted therapeutics. Nucleic Acids Res 2018;46:D1121-7. [Crossref] [PubMed]
- Otasek D, Morris JH, Bouças J, et al. Cytoscape Automation: empowering workflow-based network analysis. Genome Biol 2019;20:185. [Crossref] [PubMed]
- Martin A, Ochagavia ME, Rabasa LC, et al. BisoGenet: a new tool for gene network building, visualization and analysis. BMC Bioinformatics 2010;11:91. [Crossref] [PubMed]
- Tang Y, Li M, Wang J, et al. CytoNCA: a cytoscape plugin for centrality analysis and evaluation of protein interaction networks. Biosystems 2015;127:67-72. [Crossref] [PubMed]
- Zhou Y, Zhou B, Pache L, et al. Metascape provides a biologist-oriented resource for the analysis of systems-level datasets. Nat Commun 2019;10:1523. [Crossref] [PubMed]
- Ashburner M, Ball CA, Blake JA, et al. Gene ontology: tool for the unification of biology. The Gene Ontology Consortium. Nat Genet 2000;25:25-9. [Crossref] [PubMed]
- Zhao X, Yuan F, Wan H, et al. Mechanisms of magnoliae cortex on treating sarcopenia explored by GEO gene sequencing data combined with network pharmacology and molecular docking. BMC Genom Data 2022;23:15. [Crossref] [PubMed]
- Collins CA, Partridge TA. Self-renewal of the adult skeletal muscle satellite cell. Cell Cycle 2005;4:1338-41. [Crossref] [PubMed]
- Wei C, Ren L, Li K, et al. The regulation of survival and differentiation of neural stem cells by miR-124 via modulating PAX3. Neurosci Lett 2018;683:19-26. [Crossref] [PubMed]
- Li H, Guan K, Li X, et al. MFG-E8 induced differences in proteomic profiles in mouse C2C12 cells and its effect on PI3K/Akt and ERK signal pathways. Int J Biol Macromol 2019;124:681-8. [Crossref] [PubMed]
- Nederveen JP, Joanisse S, Snijders T, et al. The influence of capillarization on satellite cell pool expansion and activation following exercise-induced muscle damage in healthy young men. J Physiol 2018;596:1063-78. [Crossref] [PubMed]
- Boldrin L, Ross JA, Whitmore C, et al. The effect of calorie restriction on mouse skeletal muscle is sex, strain and time-dependent. Sci Rep 2017;7:5160. [Crossref] [PubMed]
- Wiedmer P, Jung T, Castro JP, et al. Sarcopenia - Molecular mechanisms and open questions. Ageing Res Rev 2021;65:101200. [Crossref] [PubMed]
- Yoon MS. mTOR as a Key Regulator in Maintaining Skeletal Muscle Mass. Front Physiol 2017;8:788. [Crossref] [PubMed]
- Laplante M, Sabatini DM. mTOR signaling in growth control and disease. Cell 2012;149:274-93. [Crossref] [PubMed]
- Baraldo M, Geremia A, Pirazzini M, et al. Skeletal muscle mTORC1 regulates neuromuscular junction stability. J Cachexia Sarcopenia Muscle 2020;11:208-25. [Crossref] [PubMed]
- Pratiwi YS, Lesmana R, Goenawan H, et al. Nutmeg Extract Increases Skeletal Muscle Mass in Aging Rats Partly via IGF1-AKT-mTOR Pathway and Inhibition of Autophagy. Evid Based Complement Alternat Med 2018;2018:2810840. [Crossref] [PubMed]
- Rom O, Reznick AZ. The role of E3 ubiquitin-ligases MuRF-1 and MAFbx in loss of skeletal muscle mass. Free Radic Biol Med 2016;98:218-30. [Crossref] [PubMed]
- Pasiakos SM, Carbone JW. Assessment of skeletal muscle proteolysis and the regulatory response to nutrition and exercise. IUBMB Life 2014;66:478-84. [Crossref] [PubMed]
- Jackson MP, Hewitt EW. Cellular proteostasis: degradation of misfolded proteins by lysosomes. Essays Biochem 2016;60:173-80. [Crossref] [PubMed]
- Bartoli M, Richard I. Calpains in muscle wasting. Int J Biochem Cell Biol 2005;37:2115-33. [Crossref] [PubMed]
- Du J, Wang X, Miereles C, et al. Activation of caspase-3 is an initial step triggering accelerated muscle proteolysis in catabolic conditions. J Clin Invest 2004;113:115-23. [Crossref] [PubMed]
- Kang SH, Lee HA, Kim M, et al. Forkhead box O3 plays a role in skeletal muscle atrophy through expression of E3 ubiquitin ligases MuRF-1 and atrogin-1 in Cushing's syndrome. Am J Physiol Endocrinol Metab 2017;312:E495-507. [Crossref] [PubMed]
- Cid-Díaz T, Santos-Zas I, González-Sánchez J, et al. Obestatin controls the ubiquitin-proteasome and autophagy-lysosome systems in glucocorticoid-induced muscle cell atrophy. J Cachexia Sarcopenia Muscle 2017;8:974-90. [Crossref] [PubMed]
- Jiao J, Demontis F. Skeletal muscle autophagy and its role in sarcopenia and organismal aging. Curr Opin Pharmacol 2017;34:1-6. [Crossref] [PubMed]
- Hiona A, Leeuwenburgh C. The role of mitochondrial DNA mutations in aging and sarcopenia: implications for the mitochondrial vicious cycle theory of aging. Exp Gerontol 2008;43:24-33. [Crossref] [PubMed]
- Park SY, Kim HY, Lee JH, et al. The age-dependent induction of apoptosis-inducing factor (AIF) in the human semitendinosus skeletal muscle. Cell Mol Biol Lett 2010;15:1-12. [Crossref] [PubMed]
- Giresi PG, Stevenson EJ, Theilhaber J, et al. Identification of a molecular signature of sarcopenia. Physiol Genomics 2005;21:253-63. [Crossref] [PubMed]
- Li WQ, Wu JY, Xiang DX, et al. Micelles Loaded With Puerarin And Modified With Triphenylphosphonium Cation Possess Mitochondrial Targeting And Demonstrate Enhanced Protective Effect Against Isoprenaline-Induced H9c2 Cells Apoptosis. Int J Nanomedicine 2019;14:8345-60. [Crossref] [PubMed]
(English Language Editor: L. Huleatt)