Application and interpretation of vessel wall magnetic resonance imaging for intracranial atherosclerosis: a narrative review
Introduction
Common diagnostic methods for intracranial atherosclerosis include magnetic resonance angiography (MRA), computed tomography angiography (CTA), transcranial doppler (TCD) sonography, and digital subtraction angiography (DSA). The commonality of these methods is that they perform intravascular imaging; however, such imaging is not suitable for evaluating the vessel wall. These methods mainly focus on assessing the degree and length of stenosis of the diseased vessel. However, the degree of luminal stenosis is not the only indicator of the severity of atherosclerosis; plaque stability and vascular remodeling are also important factors in developing ischemic stroke (1,2). Vessel wall magnetic resonance imaging (VWMRI) is a reliable examination method that enables the in-vivo analysis of the intracranial vessel wall. Compared to traditional imaging evaluation methods (i.e., MRA, CTA, TCD, and DSA), this technique can analyze the composition and properties of atherosclerotic plaques, determine plaque stability, and evaluate plaque burden and vascular remodeling. Thus, it compensates for the deficiencies of traditional imaging evaluation tools and improves the diagnostic accuracy of intracranial atherosclerotic lesions (3,4).
This review introduces the technical conditions and expert consensus on intracranial arterial VWMRI, summarizes the diagnostic points and clinical significance of VWMRI for intracranial atherosclerotic lesions, and discusses the current limitations and future direction of VWMRI. As the demand for intracranial VWMRI increases in clinical practice, radiologists should be aware of the selection of imaging parameters and how they affect image quality, clinical indications, evaluation methods, and limitations in interpreting these images. Therefore, this review focused on describing how to perform and interpret VWMRI of intracranial atherosclerotic lesions. We present the following article in accordance with the Narrative Review reporting checklist (available at https://atm.amegroups.com/article/view/10.21037/atm-22-2364/rc).
Methods
In this review, we focus on the application of VWMRI in intracranial atherosclerotic diseases. We searched the PubMed database for relevant literature between January 1, 2000 and March 31, 2022. Literature types include reviews, meta-analyses, case reports, and original studies—the language of literature in English. After the database search, the titles and abstracts were screened independently by two authors, and all authors evaluated whether the literature could be included in our study. A detailed summary of the search strategy is shown in Table 1.
Table 1
Items | Specification |
---|---|
Date of search (specified to date, month and year) | January 31, 2022 to April 7, 2022 |
Databases and other sources searched | All from the PubMed database |
Search terms used (including MeSH and free text search terms and filters) | “Vessel wall magnetic resonance imaging (VWMRI)”, “Intracranial vessel wall magnetic resonance imaging”, “Black blood imaging”, “high-resolution magnetic resonance imaging”, “Vessel wall”, “Intracranial atherosclerosis”, “Atherosclerotic disease”, “Middle cerebral artery”, “Basilar artery”, and “Atherosclerotic intracranial arterial” |
Timeframe | January 1, 2000 to March 31, 2022 |
Inclusion and exclusion criteria (study type, language restrictions etc.) | English studies including clinical trial, meta-analysis, case report, and review were collected for reviewing |
Selection process (who conducted the selection, whether it was conducted independently, how consensus was obtained, etc.) | Fangbing Li and Yilin Wang collected the studies and extracted the relevant information. All the authors jointly discussed and selected the studies to obtain the consensus of the review. |
Any additional considerations, if applicable | None |
Technical conditions for VWMRI
The minimum requirement of VWMRI is 1.5T MRI (5). Intracranial arteries are mainly medium-small arteries with tortuous travel (6). To improve image quality, 3.0T MRI is recommended for scanning. It has been confirmed that high-field strength MRI >7.0T has better tissue resolution (7), but it has not been widely used in clinical practice. Further, it has been shown that the higher the number of coil channels, the higher the tissue resolution and signal-to-noise-ratio (SNR) (8). A high-resolution head coil with at least 8 channels is recommended for scanning to obtain the appropriate image SNR.
No other special preparations are necessary before a VWMRI examination. However, it is essential to note that as the examination is usually lengthy, the patient should be informed explicitly of the approximate time required for the examination and instructed to keep their head stationary during the scan. Motion artifact is the main factor leading to examination failure; thus, avoiding motion artifacts during scanning is necessary.
In a VWMRI examination, the patient is placed in a supine position with hands on either side of the body. The long axis of the body is aligned with the long axis of the bed. The head is placed on a bracket, and the head coil is positioned with the line of the inner and outer canthus as the center. If a patient suffers from involuntary head movements, the head can be fixed with sponge pads on either side of the head to prevent head movement.
The scanning scope of VWMRI includes at least: C3 to C7 segments of the bilateral internal carotid arteries, A1 and A2 segments of the anterior cerebral artery, M1 and M2 segments of the middle cerebral artery (MCA) in the anterior circulation, P1 and P2 segments of the posterior cerebral artery in the basilar artery (BA), and V4 segments of the bilateral vertebral arteries in the posterior circulation. If the responsible vessel is unclear, the black-blood T1 sequence can be extended to the entire intracranial artery. The image quality is then divided into the following 4 levels based on the clarity of the vessel wall structure in the analyzed imaging sequence: level 1, non-diagnostic; level 2, not suitable for diagnostic purposes; level 3, adequate for diagnostic purposes; and level 4, high quality for diagnostic purposes (9).
Consensus on technical specifications for VWMRI
The current expert consensus on the technical specifications for VWMRI are as follows: (I) high spatial resolution; (II) multiplanar two-dimensional (2D) and 3D imaging; (III) multiple tissue-weighted sequences; and (IV) the suppression of blood and cerebrospinal fluid (10,11).
High spatial resolution
The physiological anatomy of the intracranial vessel wall requires VWMRI with high spatial resolution; that is, high image quality and a high-contrast SNR. Submillimeter spatial resolution is generally needed to characterize intracranial plaques well. The recommended spatial resolution requires at least <1 mm. The SNR and resolution can be improved by using high magnetic field strength and channel coils or extending the scan time. Additionally, the zero-fill interpolation within the magnetic resonance layers can also reconstruct the image resolution to <0.5 mm (12). It is important to note that extending the scan time increases the likelihood of motion artifacts; thus, the balance between the scan time and spatial resolution should be reasonably managed to avoid motion artifacts due to a long scan time.
Multiplanar 2D and 3D imaging
Various 2D and 3D imaging techniques are necessary for intracranial artery imaging. Fast spin-echo (FSE) sequences are the most commonly used 2D sequences with high-resolution and high-contrast SNR. However, FSE sequences require straighter target vessels, and tortuous vessels are prone to the “partial volume effect.” The recommended voxel size for 2D imaging sequences is 2.0×0.4×0.4 mm, which balances spatial resolution and SNR (13). Due to the limited coverage of 2D sequences, scans need to be targeted to specific lesions to maintain a short imaging time. The scans rely on the accurate localization of the extent of the lesion.
3D volumetric scanning with isotropic resolution allows custom reconstruction in any direction, enables fast and large-area imaging in a shorter time, and minimizes some volume effects. However, the current 3D sequences are inferior to 2D sequences in terms of image SNR and quality. The current 3D imaging voxels can be 0.4–1 mm (14).
The most widely used 3D sequences are variable-flip-angle fast/turbo spin-echo (VFA/FSE) sequences. These sequences provide good image quality, coverage, and flow suppression in a short scan time. VFA/FSE has been used for T1-weighted imaging (T1WI), T2-weighted imaging (T2WI), proton density-weighted imaging (PDWI), and gadolinium-based contrast enhancement T1WI (15). Other 3D techniques include flow-dephasing–prepared fast spoiled gradient recalled echo (16).
Multiple tissue-weighted sequences
The recommended sequences for VWMRI include three-dimensional time-of-flight (3D TOF) MRA, 3D and 2D T1WI, T2WI, PDWI, and contrast-enhanced T1WI sequences. Most of the intracranial VWMRI scanning protocols currently in clinical use rely on T1WI because the contrast-enhanced pre- and post-scan image characteristics allow the better differentiation of the plaques and their surrounding tissue structures and the better evaluation of plaque characteristics (17). The commonly used VWMRI sequence parameters are set out in Table 2.
Table 2
Sequences | TR (ms) | TE (ms) | Flip angle | Slice thickness (mm) | FOV (mm) | NEX | Locs per slab | Matrix | Acquisition time |
---|---|---|---|---|---|---|---|---|---|
3D TOFMRA | 23 | 2.5 | 20 | 1.4 | 220 | 3 | 32 | 320×256 | 4 min 1 s |
3D CUBE T1WI | 1,140 | 14 | – | 1 | 180 | 1 | 160 | 320×228 | 7 min 5 s |
3D CUBE T1WI with contrast agent* | 1,140 | 14 | – | 1 | 180 | 1 | 160 | 320×228 | 7 min 5 s |
3D CUBE PDWI | 2,500 | 35 | – | 1 | 180 | 1 | 160 | 320×228 | 9 min 46 s |
2D FSE T2WI | 4,000 | 42 | 125 | 2 | 130 | 4 | 16 | 256×224 | 6 min 8 s |
*, the ideal start time is 5 minutes after contrast agent injection. #, the original data in Table 2 were obtained from our published literature (18) (https://doi.org/10.3389/fneur.2021.693397). VWMRI, vessel wall magnetic resonance imaging; GE, General Electric Company; MRI, magnetic resonance imaging; 3D, three-dimensional; 2D, two-dimensional; T1WI, T1-weighted imaging; PDWI, proton density-weighted imaging; FSE, fast spin-echo; T2WI, T2-weighted imaging; TOF, time-of-flight; MRA, magnetic resonance angiography; TR, repetition time; TE, echo time; FOV, field of view; NEX, number of excitations; Locs per slab, total number of locations (slices) generated from a slab; min, minute; s, second; CUBE, variable-flip-angle turbo-spin-echo.
3D TOF MRA represents the “bright-blood” technique, which is mainly used to identify intraluminal abnormalities and as a locator for subsequent VWMRI. The main advantages of the 3D TOF MRA are the short repetition time and echo time, and fast scanning speed. Conversely, the main disadvantage is the loss of blood flow signal at the bifurcation or curvature of the vessel due to eddy currents, which affects the accurate assessment of lumen stenosis (19).
T1WI is a basic vessel wall imaging sequence that can assess plaque location, determine plaque hemorrhage, and inject contrast agents for enhanced scanning (20). By injecting gadolinium-based contrast agents, T1-enhanced sequences have higher tissue resolution than T1WI and can better distinguish plaque boundaries, describe plaque morphology, and calculate plaque burden (21). T1WI-enhanced sequences are also an essential sequence for evaluating plaque stability (22). T1WI enhancement scans are generally performed 5 minutes after the intravenous injection of a gadolinium contrast agent (flow rate: 1–2 mL/s, contrast dose: 0.1 mmol/kg) (23).
PDWI may be superior to T1WI in terms of SNR and show a higher display of fiber caps than T1 sequences, but PDWI is usually accompanied by poor cerebrospinal fluid suppression and contrast enhancement (24). Compared with TIWI, T2WI has a higher SNR and contrast SNR, is more sensitive to showing the fibrous cap and lipid core of plaques, and has better cerebrospinal fluid suppression of specific value for the differential diagnosis of vasculitis and atherosclerotic lesions (25).
Blood and cerebrospinal fluid suppression (black-blood technique)
Black-blood techniques are the focus of VWMRI, and the most commonly used black-blood 3D techniques in clinical intracranial VWMRI today are VFA/FSE, including VISTA (Philips Healthcare) and SPACE (Siemens Healthineers), and CUBE (GE Healthcare). These sequences have a long echo train, which can shorten the scan time, but signal attenuation occurs with too long an echo chain, leading to image blurring, which can be minimized by changing the flip angle, resulting in a relatively stable signal (26). A commonly used clinical technique for 2D black-blood techniques is FSE (27).
Imaging characteristics and the clinical significance of intracranial atherosclerotic lesions in VWMRI
Normal intracranial VWMRI characteristics
The normal intracranial vascular lumen diameter is about 3–5 mm, with a thin wall of about 0.2–0.3 mm thickness (28). The normal wall is difficult to observe on VWMRI (see Figure 1). Defining the VWMRI features of normal intracranial arteries is the basis for diagnosing atherosclerotic disease. The normal vessel wall should be of uniform concentric thickness, with a regular lumen and outer wall edges, and no significant enhancement of the vessel wall should be observed on the enhancement scans (29).
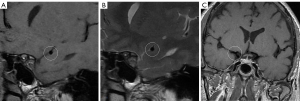
Intracranial atherosclerotic plaque pathology
Atherosclerotic plaques are composed of lipid components, platelets and fibrin, cellular material, and connective tissue matrices. Plaque progression is usually associated with increased lipid core volume within the plaque, the rupture of the fibrous cap, and intraplaque hemorrhage (IPH) (30).
Degree and length of stenosis
The Warfarin Aspirin Symptomatic Intracranial Disease criteria is widely used to measure intracranial artery stenosis as follows: stenosis rate (%) = 1 − (diameter of stenosis/diameter of normal) 100% (31), where the “diameter of stenosis” is the diameter of the residual lumen at the site of the most severe stenosis, and the “diameter of normal” is the diameter of the normal blood vessel in the proximal part of the stenosis. If the stenosis is located at the beginning of the target vessel, the widest portion of the distal lumen of the stenosis is measured as the reference diameter. Mild stenosis is defined as a stenosis rate <50%, moderate stenosis as 50–69%, severe stenosis as ≥70%, and occlusion as 100%. The stenosis length is defined as the distance from the proximal end to the distal end of the intracranial artery stenosis (32).
The degree of stenosis is associated with ischemic events; the more severe the stenosis, the higher the probability of an ischemic event. Thus, the degree of stenosis is often used to guide clinical diagnosis and treatment and determine prognosis. For example, patients with severe stenosis or occlusion may require treatment with vascular stenting (33).
Vascular remodeling caused by plaque
Vascular remodeling includes positive remodeling, negative remodeling, and no remodeling. The remodeling ratio is used to judge vascular remodeling. The ratio between the vascular area at the plaque and the vascular area proximal to the plaque is defined as remodeling ratio. Specifically, a value <0.95 indicates negative remodeling, and a value between 0.95 and 1.05 indicates no remodeling (34).
VWMRI can accurately evaluate vascular remodeling. Conversely, conventional imaging methods cannot accurately determine vascular remodeling and underestimate the degree of luminal stenosis. Positive remodeling is a compensatory external expansion of the vessel wall that may be caused by IPH and inflammation (35). The present meta-analysis confirmed that positive remodeling is a specific vulnerability marker in intracranial plaques (36,37). As a marker of plaque vulnerability, the vessel wall with positive remodeling generally has a larger lipid core and inflammatory cell infiltration. At the same time, these inflammatory cells are usually concentrated at the edge of the plaque, where the thin fibrous cap is most likely to rupture (38).
Vessel wall thickening shape and plaque distribution
The eccentricity index evaluates vessel wall thickening. The eccentricity index = the maximum vessel wall thickness—the minimum vessel wall thickness/the maximum vessel wall thickness. An eccentricity index ≥0.5 indicates the thickening of the eccentric vessel wall; otherwise, it indicates the thickening of the centripetal (circumferential) vessel wall (39). The shape of atherosclerotic plaque resulting in the thickening of vessel walls is usually eccentric but can also appear centripetal.
In a cross-section of the long axis of the vessel, the vessel cross-section is divided equally into 4 quadrants using the center of the lumen as the center of the circle, and the vessel walls in each quadrant of the MCA are the superior, inferior, ventral, and dorsal, respectively, while the BA is the ventral, dorsal, and left and right (see Figure 1). When plaque is involved in >1 quadrant, the quadrant in which the thickest wall is located is selected as the plaque distribution. The distribution of plaque is associated with the risk of stroke (40), and plaque in the superior of the MCA is more likely to cause ischemic cerebral infarction than plaque in the ventral MCA. The location of basilar plaque should be considered during basilar angioplasty. The Snowplowing effect should be avoided during stent implantation when basilar plaque is involved in branching artery openings (41).
Plaque signal characteristics
Plaque fibrous cap: the fibrous cap and lipid core are not distinguishable in any sequence, and in many cases, VWMRI cannot distinguish well between the 2. A typical fibrous cap shows iso/hypointensity on T1WI, iso/hyperintensity on T2WI, and iso/hypointensity on PDWI (see Figure 2). T2WI can more accurately assess the status of the fibrous cap of the plaque. The uniformity of the thinness, surface smoothness, and degree of enhancement of the fibrous cap are important factors in evaluating plaque stability (42).
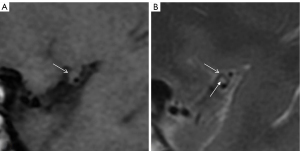
Plaque lipid core: the lipid core in the plaque shows iso-intensity on T1WI, hypointensity on T2WI, and iso/hypointensity on PDWI. The difference in MRI signal between plaque lipid core and normal adipose tissue may be due to the following factor: the direct reflection of the MRI signal in atheromatous plaques if the relatively lipid-rich core is water rather than lipid. The lipids of atheromatous plaques are mainly cholesterol and cholesteryl esters, which do not cause a shortening of T1 relaxation time similar to extravascular lipids (43). Additionally, contrast-enhanced T1WI is the best sequence to show the extent of plaque. Specifically, it shows the enhancement of the fibrous cap and extravascular membrane around the plaque, but not the plaque, and thus provides a more accurate outline of the extent of plaque or measurement of plaque volume. The plaque lipid core is also associated with plaque stability, and a previous study suggested that the risk of plaque rupture increases when the plaque lipid core volume reaches >60% of the entire volume of the plaque (10).
IPH: the most sensitive sequence for IPH is T1WI (see Figure 3). IPH generally shows hyperintensity on T1WI and hypo/hyperintensity on T2WI, but the signal of plaque hemorrhage is complex and is correlated with the timing of the hemorrhage (44). IPH is generally considered a biological feature of plaque instability.
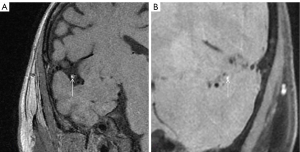
Plaque calcification: VWMRI is inadequate at detecting calcification, which generally shows hypointensity on VWMRI sequences. A previous study reported that the internal carotid artery was the segment most frequently affected by intracranial arterial calcification (IAC) (60%), while the MCA and the BA were rarely affected (5%, respectively) (45). Intimal IAC often coexists with luminal stenosis, larger plaque burden, eccentric plaque, and IPH (46).
Plaque enhancement
The degree of plaque enhancement refers to the strengthening of the pituitary stalk or the brain parenchyma adjacent to the intracranial artery (see Figure 4). Plaque enhancement is classified into the following3 types: (I) significant enhancement: the degree of enhancement is higher than or equal to that of the pituitary stalk; (II) mild enhancement: the degree of enhancement is weaker than that of the pituitary stalk; and (III) no enhancement: there is no significant change compared to T1WI (47). The mechanisms by which enhancement occurs in intracranial arterial plaques are multifactorial. The mechanism of plaque enhancement that causes symptomatic intracranial atherosclerotic disease is similar to that of unstable carotid plaques, for which the causes of plaque enhancement include intraplaque neovascularization and inflammatory infiltration (48). An ultrastructural study of ruptured coronary plaques showed an increased density of thin-walled microvessels within the ruptured plaque with damaged endothelial cell junctions and extensions from the outer membrane, which may account for the infiltration of gadolinium-based contrast agents within the plaque (49). Intracranial artery plaque enhancement may be similar to this mechanism (49).
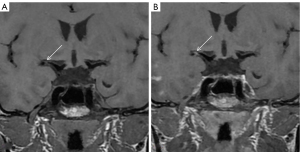
Intracranial plaque enhancement is strongly associated with ischemic stroke, independent of the degree of stenosis. Significant plaque enhancement may suggest plaque instability and an increased risk of ischemic stroke (50). Additionally, the different enhancement characteristics of plaques can identify the cause of stenoses, such as arteritis or atherosclerotic lesions (51).
Evaluation of plaque stability
Unstable plaques, also known as vulnerable plaques, are atherosclerotic plaques that are at risk of rupture. Plaque stability is highly correlated with ischemic stroke and stroke recurrence (52), and the following imaging features may indicate plaques at risk of instability: an uneven thickness and surface irregularity of the fibrous cap; localized breakage of the fibrous cap; MCA plaques located in the superior wall, and BA plaques located in the lateral wall and arterial openings; large intraplaque lipid cores that are >60% of the whole plaque volume; IPH; positive remodeling, and varying degrees of homogeneous or non-homogeneous enhancement. A meta-analysis concluded that severe luminal stenosis, positive remodeling, plaque surface irregularities, and plaque enhancement are strongly associated with the development of ischemic stroke (53).
Development directions and limitations of VWMRI
The application of VWMRI to the comprehensive evaluation of intracranial atherosclerosis disease (ICAD) and the basis for developing clinical treatment strategies depends on optimizing and harmonizing different scanning device sequences and the reliable and accurate interpretation of the images. Future research needs to be conducted to improve the scanning efficiency and diagnostic accuracy and facilitate clinical applications. Additionally, image refinement and the standardization of VWMRI feature definitions are key areas to consider for future development. VWMRI is a future research direction for improving image resolution and tissue contrast, shortening the scan time, fusing magnetic resonance hemodynamics, vessel wall shear and vessel wall imaging, and conducting large-scale integrated evaluations of head-neck vessels. With the accumulation of many multiparametric data from VWMRI, artificial intelligence may hopefully provide more reliable and accurate data for ICAD and stroke risk assessments.
With the continuous development of magnetic resonance hardware and software, VWMRI technology is gradually maturing, the imaging technique is being progressively optimized, and the combined use of various pulse sequences is improving the effect of blood flow suppression. The spatial coverage of the responsible vessels has been increased, and the detection of plaque, the quantitative analysis of plaque, and the display of the lumen structure have been made more accurate. VWMRI has very high accuracy and reproducibility in evaluating plaque stenosis, plaque burdening, remodeling ratio, and vessel wall thickness by automated, semi-automated, or manual methods. However, only a few prospective, multicenter studies with large samples have been conducted (54,55). Unlike internal carotid artery VWMRI, intracranial artery VWMRI is still unavailable for obtaining in-vivo plaque pathology specimens for imaging-pathology control. VWMRI requires longer imaging times and has higher economic costs, limiting its application under specific conditions.
Summary
VWMRI complements conventional imaging methods in evaluating ICAD, and thus allows for the earlier diagnosis of ICAD and the provision of more informative imaging information. VWMRI has a wide range of clinical applications and can address important clinical questions and provide critical information for treatment decisions.
Acknowledgments
Funding: The project was funded by Natural Science Foundation of Liaoning Province (No. 2019-ZD-0762).
Footnote
Reporting Checklist: The authors have completed the Narrative Review reporting checklist. Available at https://atm.amegroups.com/article/view/10.21037/atm-22-2364/rc
Conflicts of Interest: All authors have completed the ICMJE uniform disclosure form (available at https://atm.amegroups.com/article/view/10.21037/atm-22-2364/coif). Y Wu reports funding from the Natural Science Foundation of Liaoning Province (No. 2019-ZD-0762). The other authors have no conflicts of interest to declare.
Ethical Statement: The authors are accountable for all aspects of the work in ensuring that questions related to the accuracy or integrity of any part of the work are appropriately investigated and resolved.
Open Access Statement: This is an Open Access article distributed in accordance with the Creative Commons Attribution-NonCommercial-NoDerivs 4.0 International License (CC BY-NC-ND 4.0), which permits the non-commercial replication and distribution of the article with the strict proviso that no changes or edits are made and the original work is properly cited (including links to both the formal publication through the relevant DOI and the license). See: https://creativecommons.org/licenses/by-nc-nd/4.0/.
References
- Teng Z, Peng W, Zhan Q, et al. An assessment on the incremental value of high-resolution magnetic resonance imaging to identify culprit plaques in atherosclerotic disease of the middle cerebral artery. Eur Radiol 2016;26:2206-14. [Crossref] [PubMed]
- Zhang DF, Chen YC, Chen H, et al. A High-Resolution MRI Study of Relationship between Remodeling Patterns and Ischemic Stroke in Patients with Atherosclerotic Middle Cerebral Artery Stenosis. Front Aging Neurosci 2017;9:140. [Crossref] [PubMed]
- Lu SS, Ge S, Su CQ, et al. MRI of plaque characteristics and relationship with downstream perfusion and cerebral infarction in patients with symptomatic middle cerebral artery stenosis. J Magn Reson Imaging 2018;48:66-73. [Crossref] [PubMed]
- Holmstedt CA, Turan TN, Chimowitz MI. Atherosclerotic intracranial arterial stenosis: risk factors, diagnosis, and treatment. Lancet Neurol 2013;12:1106-14. [Crossref] [PubMed]
- Sakurai K, Miura T, Sagisaka T, et al. Evaluation of luminal and vessel wall abnormalities in subacute and other stages of intracranial vertebrobasilar artery dissections using the volume isotropic turbo-spin-echo acquisition (VISTA) sequence: a preliminary study. J Neuroradiol 2013;40:19-28. [Crossref] [PubMed]
- Cogswell PM, Lants SK, Davis LT, et al. Vessel wall and lumen characteristics with age in healthy participants using 3T intracranial vessel wall magnetic resonance imaging. J Magn Reson Imaging 2019;50:1452-60. [Crossref] [PubMed]
- Zwartbol MHT, van der Kolk AG, Ghaznawi R, et al. Intracranial Vessel Wall Lesions on 7T MRI (Magnetic Resonance Imaging). Stroke 2019;50:88-94. [Crossref] [PubMed]
- Hu X, Li Y, Zhang L, et al. A 32-channel coil system for MR vessel wall imaging of intracranial and extracranial arteries at 3T. Magn Reson Imaging 2017;36:86-92. [Crossref] [PubMed]
- Wu Y, Li F, Wang Y, et al. High-Resolution Vessel Wall Magnetic Resonance Imaging of the Middle Cerebral Artery: Comparison of 3D CUBE T1-Weighted Sequence with and without Fat Suppression. Med Sci Monit 2020;26:e928931. [Crossref] [PubMed]
- Mandell DM, Mossa-Basha M, Qiao Y, et al. Intracranial Vessel Wall MRI: Principles and Expert Consensus Recommendations of the American Society of Neuroradiology. AJNR Am J Neuroradiol 2017;38:218-29. [Crossref] [PubMed]
- Lindenholz A, van der Kolk AG, Zwanenburg JJM, et al. The Use and Pitfalls of Intracranial Vessel Wall Imaging: How We Do It. Radiology 2018;286:12-28. [Crossref] [PubMed]
- Luo J, Mou Z, Qin B, et al. Fast single image super-resolution using estimated low-frequency k-space data in MRI. Magn Reson Imaging 2017;40:1-11. [Crossref] [PubMed]
- Wong SK, Mobolaji-Iawal M, Arama L, et al. Atherosclerosis imaging using 3D black blood TSE SPACE vs 2D TSE. World J Radiol 2014;6:192-202. [Crossref] [PubMed]
- Lou X, Ma N, Shen H, et al. Noninvasive visualization of the basilar artery wall and branch ostia with high-resolution three-dimensional black-blood sequence at 3 tesla. J Magn Reson Imaging 2014;39:911-6. [Crossref] [PubMed]
- Mattay RR, Saucedo JF, Lehman VT, et al. Current Clinical Applications of Intracranial Vessel Wall MR Imaging. Semin Ultrasound CT MR 2021;42:463-73. [Crossref] [PubMed]
- Mazzacane F, Mazzoleni V, Scola E, et al. Vessel Wall Magnetic Resonance Imaging in Cerebrovascular Diseases. Diagnostics (Basel) 2022;12:258. [Crossref] [PubMed]
- Chaganti J, Woodford H, Tomlinson S, et al. Black blood imaging of intracranial vessel walls. Pract Neurol 2020; Epub ahead of print. [Crossref] [PubMed]
- Wu Y, Li F, Wang Y, et al. Standard-Dose Atorvastatin Treatment in Patients With Symptomatic Middle Cerebral Artery Atherosclerotic Stenosis: A Vessel Wall Magnetic Resonance Imaging Study. Front Neurol 2021;12:693397. [Crossref] [PubMed]
- Gupta A, Baradaran H, Al-Dasuqi K, et al. Gadolinium Enhancement in Intracranial Atherosclerotic Plaque and Ischemic Stroke: A Systematic Review and Meta-Analysis. J Am Heart Assoc 2016;5:003816. [Crossref] [PubMed]
- Kim DK, Verdoorn JT, Gunderson TM, et al. Comparison of non-contrast vessel wall imaging and 3-D time-of-flight MRA for atherosclerotic stenosis and plaque characterization within intracranial arteries. J Neuroradiol 2020;47:266-71. [Crossref] [PubMed]
- Zhang L, Zhang N, Wu J, et al. High resolution three dimensional intracranial arterial wall imaging at 3 T using T1 weighted SPACE. Magn Reson Imaging 2015;33:1026-34. [Crossref] [PubMed]
- Jang J, Kim TW, Hwang EJ, et al. Assessment of Arterial Wall Enhancement for Differentiation of Parent Artery Disease from Small Artery Disease: Comparison between Histogram Analysis and Visual Analysis on 3-Dimensional Contrast-Enhanced T1-Weighted Turbo Spin Echo MR Images at 3T. Korean J Radiol 2017;18:383-91. [Crossref] [PubMed]
- Ryu CW, Jahng GH, Shin HS. Gadolinium enhancement of atherosclerotic plaque in the middle cerebral artery: relation to symptoms and degree of stenosis. AJNR Am J Neuroradiol 2014;35:2306-10. [Crossref] [PubMed]
- Mossa-Basha M, Hwang WD, De Havenon A, et al. Multicontrast high-resolution vessel wall magnetic resonance imaging and its value in differentiating intracranial vasculopathic processes. Stroke 2015;46:1567-73. [Crossref] [PubMed]
- Settecase F, Rayz VL. Advanced vascular imaging techniques. Handb Clin Neurol 2021;176:81-105. [Crossref] [PubMed]
- Young CC, Bonow RH, Barros G, et al. Magnetic resonance vessel wall imaging in cerebrovascular diseases Neurosurg Focus 2019;47:E4. [Crossref] [PubMed]
- Pacheco FT, Cruz LCHD Junior, Padilha IG, et al. Current uses of intracranial vessel wall imaging for clinical practice: a high-resolution MR technique recently available. Arq Neuropsiquiatr 2020;78:642-50. [Crossref] [PubMed]
- Bhogal P, Navaei E, Makalanda HL, et al. Intracranial vessel wall MRI. Clin Radiol 2016;71:293-303. [Crossref] [PubMed]
- Zwartbol MH, van der Kolk AG, Kuijf HJ, et al. Intracranial vessel wall lesions on 7T MRI and MRI features of cerebral small vessel disease: The SMART-MR study. J Cereb Blood Flow Metab 2021;41:1219-28. [Crossref] [PubMed]
- Fuster V, Moreno PR, Fayad ZA, et al. Atherothrombosis and high-risk plaque: part I: evolving concepts. J Am Coll Cardiol 2005;46:937-54. [Crossref] [PubMed]
- Samuels OB, Joseph GJ, Lynn MJ, et al. A standardized method for measuring intracranial arterial stenosis. AJNR Am J Neuroradiol 2000;21:643-6. [PubMed]
- Wu Y, Li F, Wang Y, et al. Utility of minimum intensity projection images based on three-dimensional CUBE T1 weighted imaging for evaluating middle cerebral artery stenosis. Br J Radiol 2021;94:20210145. [Crossref] [PubMed]
- Bonati LH, Jansen O, de Borst GJ, et al. Management of atherosclerotic extracranial carotid artery stenosis. Lancet Neurol 2022;21:273-83. [Crossref] [PubMed]
- Qiao Y, Anwar Z, Intrapiromkul J, et al. Patterns and Implications of Intracranial Arterial Remodeling in Stroke Patients. Stroke 2016;47:434-40. [Crossref] [PubMed]
- Song JW, Pavlou A, Xiao J, et al. Vessel Wall Magnetic Resonance Imaging Biomarkers of Symptomatic Intracranial Atherosclerosis: A Meta-Analysis. Stroke 2021;52:193-202. [Crossref] [PubMed]
- Zhang DF, Wu XY, Zhang WD, et al. The Relationship between Patterns of Remodeling and Degree of Enhancement in Patients with Atherosclerotic Middle Cerebral Artery Stenosis: A High-Resolution MRI Study. Neurol India 2021;69:1663-9. [Crossref] [PubMed]
- Won SY, Cha J, Choi HS, et al. High-Resolution Intracranial Vessel Wall MRI Findings Among Different Middle Cerebral Artery Territory Infarction Types. Korean J Radiol 2022;23:333-42. [Crossref] [PubMed]
- Zhang D, Wang M, Wu L, et al. Assessing the characteristics and diagnostic value of plaques for patients with acute stroke using high-resolution magnetic resonance imaging. Quant Imaging Med Surg 2022;12:1529-38. [Crossref] [PubMed]
- Liang J, Guo J, Liu D, et al. Application of High-Resolution CUBE Sequence in Exploring Stroke Mechanisms of Atherosclerotic Stenosis of Middle Cerebral Artery. J Stroke Cerebrovasc Dis 2019;28:156-62. [Crossref] [PubMed]
- Lu SS, Ge S, Su CQ, et al. Plaque Distribution and Characteristics in Low-Grade Middle Cerebral Artery Stenosis and Its Clinical Relevance: A 3-Dimensional High-Resolution Magnetic Resonance Imaging Study. J Stroke Cerebrovasc Dis 2018;27:2243-9. [Crossref] [PubMed]
- Song JW, Pavlou A, Burke MP, et al. Imaging endpoints of intracranial atherosclerosis using vessel wall MR imaging: a systematic review. Neuroradiology 2021;63:847-56. [Crossref] [PubMed]
- Jiang Y, Zhu C, Peng W, et al. Ex-vivo imaging and plaque type classification of intracranial atherosclerotic plaque using high resolution MRI. Atherosclerosis 2016;249:10-6. [Crossref] [PubMed]
- Yang WJ, Wong KS, Chen XY. Intracranial Atherosclerosis: From Microscopy to High-Resolution Magnetic Resonance Imaging. J Stroke 2017;19:249-60. [Crossref] [PubMed]
- Xiao J, Padrick MM, Jiang T, et al. Acute ischemic stroke versus transient ischemic attack: Differential plaque morphological features in symptomatic intracranial atherosclerotic lesions. Atherosclerosis 2021;319:72-8. [Crossref] [PubMed]
- Ahmed M, McPherson R, Abruzzo A, et al. Carotid Artery Calcification: What We Know So Far. Cureus 2021;13:e18938. [Crossref] [PubMed]
- Du H, Li J, Yang W, et al. Intracranial Arterial Calcification and Intracranial Atherosclerosis: Close but Different. Front Neurol 2022;13:799429. [Crossref] [PubMed]
- Lu Y, Ye MF, Zhao JJ, et al. Gadolinium enhancement of atherosclerotic plaque in the intracranial artery. Neurol Res 2021;43:1040-9. [Crossref] [PubMed]
- Kim JM, Jung KH, Sohn CH, et al. Intracranial plaque enhancement from high resolution vessel wall magnetic resonance imaging predicts stroke recurrence. Int J Stroke 2016;11:171-9. [Crossref] [PubMed]
- Sluimer JC, Kolodgie FD, Bijnens AP, et al. Thin-walled microvessels in human coronary atherosclerotic plaques show incomplete endothelial junctions relevance of compromised structural integrity for intraplaque microvascular leakage. J Am Coll Cardiol 2009;53:1517-27. [Crossref] [PubMed]
- Kwee RM, Qiao Y, Liu L, et al. Temporal course and implications of intracranial atherosclerotic plaque enhancement on high-resolution vessel wall MRI. Neuroradiology 2019;61:651-7. [Crossref] [PubMed]
- Wu F, Ma Q, Song H, et al. Differential Features of Culprit Intracranial Atherosclerotic Lesions: A Whole-Brain Vessel Wall Imaging Study in Patients With Acute Ischemic Stroke. J Am Heart Assoc 2018;7:009705. [Crossref] [PubMed]
- Saam T, Hatsukami TS, Takaya N, et al. The vulnerable, or high-risk, atherosclerotic plaque: noninvasive MR imaging for characterization and assessment. Radiology 2007;244:64-77. [Crossref] [PubMed]
- Lee HN, Ryu CW, Yun SJ. Vessel-Wall Magnetic Resonance Imaging of Intracranial Atherosclerotic Plaque and Ischemic Stroke: A Systematic Review and Meta-Analysis. Front Neurol 2018;9:1032. [Crossref] [PubMed]
- Dieleman N, van der Kolk AG, Zwanenburg JJ, et al. Imaging intracranial vessel wall pathology with magnetic resonance imaging: current prospects and future directions. Circulation 2014;130:192-201. [Crossref] [PubMed]
- Chung JW, Cha J, Lee MJ, et al. Intensive Statin Treatment in Acute Ischaemic Stroke Patients with Intracranial Atherosclerosis: a High-Resolution Magnetic Resonance Imaging study (STAMINA-MRI Study). J Neurol Neurosurg Psychiatry 2020;91:204-11. [Crossref] [PubMed]
Cite this article as: Li F, Wang Y, Hu T, Wu Y. Application and interpretation of vessel wall magnetic resonance imaging for intracranial atherosclerosis: a narrative review. Ann Transl Med 2022;10:714.